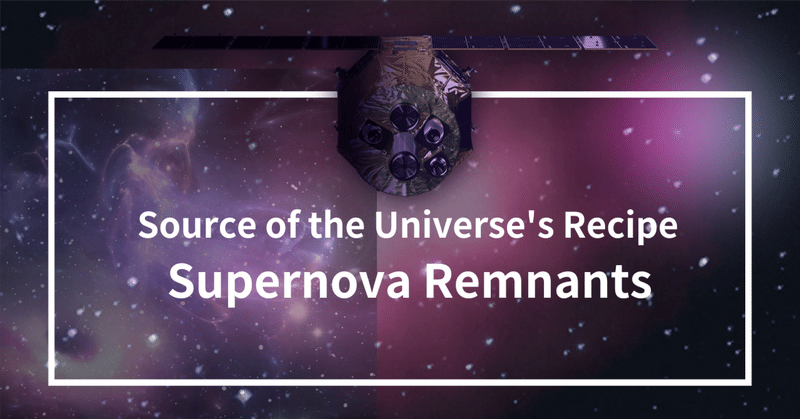
Source of the Universe's Recipe - Supernova Remnants
The matter around us is made up of various combinations of elements. For example, the material of the display you see now is glass. The main components of glass are oxygen and silicon. Oxygen and silicon are relatively common elements on Earth and in space, but where did they come from?
X-ray astronomy answers this question.
When we search the universe with X-rays, we find traces of what appear to be explosions here and there in the Milky Way. These are supernova remnants, and Figure 1 shows one of the examples. As the name suggests, they are remnants of exploded stars (supernovae).
Some stars explode at the end of their lives. The explosion is so powerful that it becomes a vast fireball of several million degrees Celsius and expands through space at a tremendous speed of several thousand kilometers per second. The supernova remnant cools as it expands, and after tens of thousands of years, it disperses and becomes invisible.
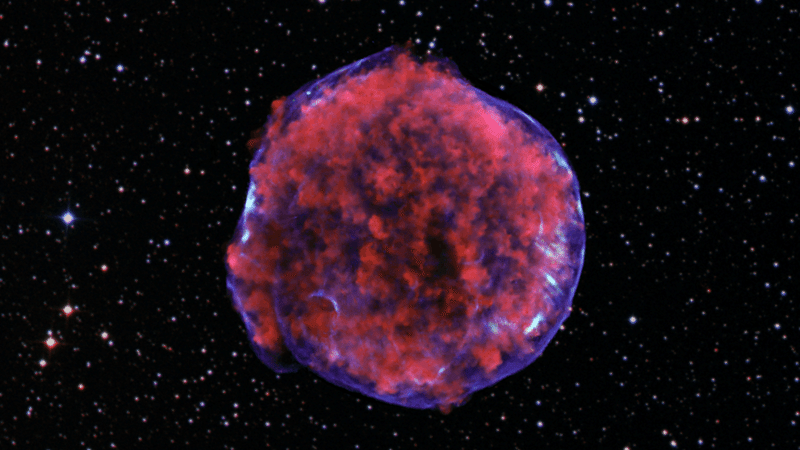
Therefore, the supernova remnant we see now tells us a supernova exploded there during the last tens of thousands of years.
The supernova remnant shown in Figure 1 is SN1006, a supernova remnant recorded worldwide as having exploded in 1006 A.D.
In 1006 A.D., during the Heian period in Japan, it is known that Fujiwara no Michinaga resolved to pray for a possible bad omen (Midou Kanpaku Ki), and Fujiwara no Teika left a memorandum (Meigetsuki Ki). In these records, a bright star suddenly appeared in the night sky, which people at that time thought was the birth of a new star, but the star exploded at that moment.
What is the phenomenon of a star exploding?
The most familiar star, the Sun, is thought to "not" explode. The Sun shines because of an internal nuclear fusion reaction. In another 5 billion years, it will run out of fuel, eventually cool down, and shrink to become a white dwarf. This is the final state of the Sun.
However, their end differs for massive stars, which are more than one order of magnitude heavier than the Sun. When a massive star runs out of fuel, its gravity causes it to contract at once, releasing enormous gravitational energy explosively and scattering the star's constituent elements into space. This is called a "gravitational collapse" supernova explosion. At the most compressed center, a neutron star or black hole is thought to remain.
In the case of white dwarfs, when a white dwarf receives a supply of gas from a neighboring star or merges with another white dwarf, the internal temperature rises, and a violent fusion reaction leads to an explosion. Astronomers call this a "Type Ia" supernova explosion. The supernova remnant in Figure 1 is an explosion of this type. Both are the spectacular end of a star and shine with a brightness comparable to that of the Milky Way Galaxy.
The enormous amount of energy released in a supernova explosion causes the synthesis of various elements inside the star (this is called explosive elemental synthesis), which are then scattered into space. The scattered "stellar debris" fills the interior of the giant fireball of a supernova remnant.
Elements synthesized in supernova explosions are thus continually supplied to the galaxy.
What elements exist there?
What elements are scattered in a supernova explosion?
The 19th-century French philosopher Auguste Comte declared that the composition of a celestial body is a question we will never answer. Intuitively, this is true. However, modern astronomy has made it possible.
This is where X-ray astronomy comes in. Elements heated to millions of degrees Celsius in hot gas, such as supernova remnants, glow with unique X-ray colors (see "Introduction - What Does XRISM Study in the Universe?" ). By measuring their color, we can derive what elements are present, even from a distance. This is the same logic as determining what kind of gunpowder was used in a fireworks display by looking at the "color" of the fireworks.
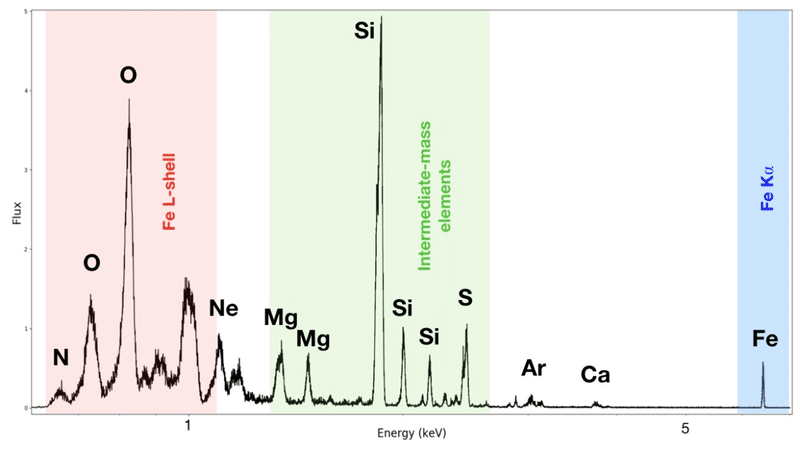
Figure 2 demonstrates how the spectrum of a Type Ia supernova remnant would look like by XRISM. Many unique X-rays (emission lines) emitted by the elements are visible. The differences in energy represent differences in "color," and the presence of elements such as nitrogen, oxygen, neon, magnesium, silicon, sulfur, argon, calcium, and iron can be seen.
The above discussion brings us to the answer to the first question; most of the oxygen and silicon in the universe today were created in supernova explosions.
Lighter elements, such as oxygen, are mainly responsible for the gravitational decay type, while heavier elements, such as silicon and iron, are mainly responsible for the Ia type. The elemental abundances of the observed supernova remnants, combined with the frequency of supernova explosions, can roughly explain the elemental composition of the universe (very heavy elements such as gold and lead are thought to have been created by neutron star mergers, and the gravitational waves emitted by such explosions are detected). The detection of gravitational waves emitted during such events made headlines in 2017).
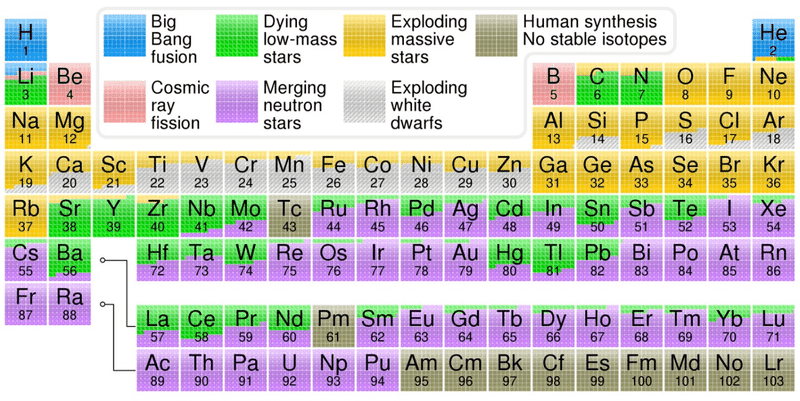
In the periodic table in Figure 3, yellow and gray are the elements supplied by gravitational decay and Type Ia, respectively. Phosphorus, chlorine, calcium, iron, and other elements that we are familiar with or make up our own bodies were created in supernova explosions that occurred somewhere in the universe before the solar system was formed.
The elements listed above can be detected by conventional X-ray astronomy satellites. However, XRISM has dramatically improved the ability to distinguish "color". Thus, XRISM's data enables us to detect rare elements that were difficult to find in the past. Furthermore, XRISM also enables us to derive their motion and ionization states. This allows us to see three-dimensionally what elements are inside a supernova remnant and how they are diffused into space.
Why is this important?
A Chemical Recipe for the Universe
In fact, what is happening inside a star at the moment of a supernova explosion, whether of the gravitational collapse type or Type Ia, is one of the great unsolved problems in astronomy that cannot be determined even with the best of modern physics.
If we can measure the dispersion of various elements with XRISM, we can estimate the internal structure of a star at the moment of explosion, just like playing a movie in reverse. This is the same logic as being able to estimate the arrangement of gunpowder in a fireworks display from the "shape" of the spreading fireworks. If we can determine this, we will be one step closer to elucidating the mechanism of supernova explosions.
As explained in note #01-01, galaxy clusters, the largest objects in the universe, contain large amounts of elements. Their source is the supernova explosions that occurred in individual galaxies. So to speak, XRISM can reveal what is happening at the starting point of the chemical recipe of the universe.
Author: Hiroyuki Uchida
(This article was translated from Japanese.)