SARS-CoV-2感染は細胞内のヘパラン硫酸とACE2に依存する
SARS-CoV-2 Infection Depends on Cellular Heparan Sulfate and ACE2
SARS-CoV-2感染は細胞内のヘパラン硫酸とACE2に依存する
元→SARS-CoV-2 Infection Depends on Cellular Heparan Sulfate and ACE2
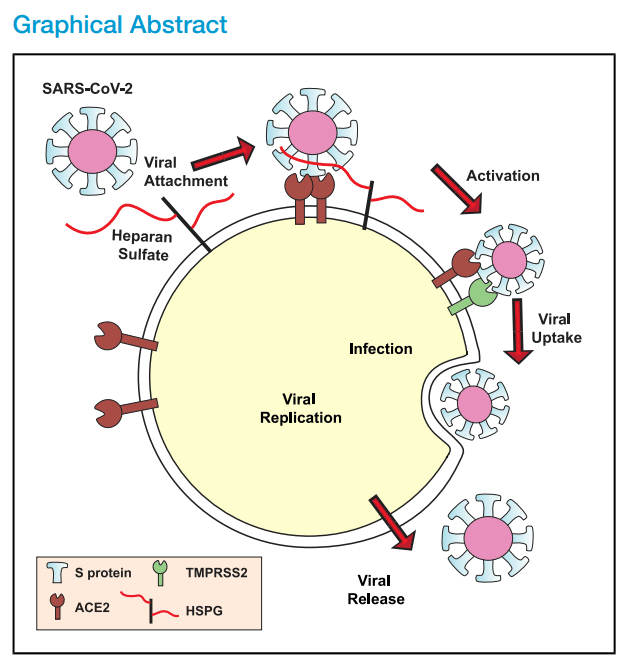
Highlights
・SARS-CoV-2 spike protein interacts with heparan sulfate and ACE2 through the RBD
SARS-CoV-2 スパイクタンパク質は、RBD を介してヘパラン硫酸および ACE2 と相互作用します
・Heparan sulfate promotes Spike-ACE2 interaction
ヘパラン硫酸はスパイクと ACE2 の相互作用を促進します
・SARS-CoV-2 infection is co-dependent on heparan sulfate and ACE2
SARS-CoV-2 感染はヘパラン硫酸と ACE2 に依存している
・Heparin and non-anticoagulant derivatives block SARS-CoV-2 binding and infection
ヘパリンと非抗凝固誘導体は SARS-CoV-2 の結合と感染を阻害する
Summary
We show that SARS-CoV-2 spike protein interacts with both cellular heparan sulfate and angiotensin-converting enzyme 2 (ACE2) through its receptor-binding domain (RBD).
SARS-CoV-2 スパイクタンパク質は、受容体結合ドメイン (RBD) を介して細胞ヘパラン硫酸とアンジオテンシン変換酵素 2 (ACE2) の両方と相互作用することを示しています。
Docking studies suggest a heparin/heparan sulfate-binding site adjacent to the ACE2-binding site.
ドッキング研究は、ACE2 結合部位に隣接するヘパリン/ヘパラン硫酸結合部位を示唆しています。
Both ACE2 and heparin can bind independently to spike protein in vitro, and a ternary complex can be generated using heparin as a scaffold.
ACE2 とヘパリンは両方とも in vitro でスパイクタンパク質に独立して結合することができ、ヘパリンを足場として使用して三元複合体を生成できます。
Electron micrographs of spike protein suggests that heparin enhances the open conformation of the RBD that binds ACE2.
スパイクタンパク質の電子顕微鏡写真は、ヘパリンが ACE2 に結合する RBD のオープン構造を強化することを示唆しています。
On cells, spike protein binding depends on both heparan sulfate and ACE2.
細胞上では、スパイクタンパク質の結合はヘパラン硫酸と ACE2 の両方に依存します。
Unfractionated heparin, non-anticoagulant heparin, heparin lyases, and lung heparan sulfate potently block spike protein binding and/or infection by pseudotyped virus and authentic SARS-CoV-2 virus.
未分画ヘパリン、非抗凝固ヘパリン、ヘパリンリアーゼ、および肺ヘパラン硫酸は、偽型ウイルスおよび本物の SARS-CoV-2 ウイルスによるスパイクタンパク質の結合および/または感染を強力にブロックします。
We suggest a model in which viral attachment and infection involves heparan sulfate-dependent enhancement of binding to ACE2.
ウイルスの付着と感染には、ヘパラン硫酸に依存した ACE2 への結合の強化が関与しているというモデルを提案します。
Manipulation of heparan sulfate or inhibition of viral adhesion by exogenous heparin presents new therapeutic opportunities.
ヘパラン硫酸の操作、または外因性ヘパリンによるウイルス付着の阻害は、新たな治療の機会をもたらします。
Introduction
The COVID-19 pandemic, caused by SARS-CoV-2, has swept across the world, resulting in serious clinical morbidities and mortality, as well as widespread disruption to all aspects of society.
SARS-CoV-2によって引き起こされたCOVID-19パンデミックは世界中に広がり、深刻な臨床的罹患率と死亡率、そして社会のあらゆる側面への広範な混乱をもたらしました。
As of September 1, 2020, the virus has spread to 215 countries, causing more than 25.4 million confirmed infections and at least 851,000 deaths (World Health Organization).
2020年9月1日現在、ウイルスは215か国に広がり、2,540万人以上の感染が確認され、少なくとも85万1,000人が死亡しています(世界保健機関)。
Current isolation/social-distancing strategies seek to flatten the infection curve to avoid overwhelming hospitals and to give the medical establishment and pharmaceutical companies time to develop and test antiviral drugs and vaccines.
現在の隔離/社会的距離戦略は、病院の過密を避け、医療機関と製薬会社に抗ウイルス薬とワクチンの開発と試験の時間を与えるために、感染曲線を平坦化することを目指しています。
Currently, only one antiviral agent, Remdesivir, has been approved for adult COVID-19 patients, and vaccines may be 6–12 months away.
現在、成人のCOVID-19患者に承認されている抗ウイルス薬はレムデシビルのみであり、ワクチンは6〜12か月先になる可能性があります。
Understanding the mechanism for SARS-CoV-2 infection and its mechanism of infection could reveal other targets to interfere with viral infection and spread.
SARS-CoV-2感染のメカニズムとその感染メカニズムを理解することで、ウイルス感染と拡散を阻止する他のターゲットが明らかになる可能性があります。
The glycocalyx is a complex mixture of glycans and glycoconjugates surrounding all cells.
グリコカリックスは、すべての細胞を取り囲むグリカンと複合糖質の複雑な混合物です。
Given its location, viruses and other infectious organisms must pass through the glycocalyx to engage receptors thought to mediate viral entry into host cells.
その位置を考えると、ウイルスやその他の感染性生物は、宿主細胞へのウイルスの侵入を仲介すると考えられる受容体と結合するために、グリコカリックスを通過する必要があります。
Many viral pathogens have evolved to utilize glycans as attachment factors, which facilitates the initial interaction with host cells, including influenza virus, herpes simplex virus, human immunodeficiency virus, and different coronaviruses (SARS-CoV-1 and MERS-CoV).
インフルエンザウイルス、単純ヘルペスウイルス、ヒト免疫不全ウイルス、およびさまざまなコロナウイルス(SARS-CoV-1 および MERS-CoV)など、多くのウイルス病原体は、グリカンを付着因子として利用するように進化しており、これにより宿主細胞との最初の相互作用が容易になります。
Several viruses interact with sialic acids, which are located on the ends of glycans found in glycolipids and glycoproteins.
いくつかのウイルスは、糖脂質や糖タンパク質に含まれるグリカンの末端にあるシアリン酸と相互作用します。
Other viruses interact with heparan sulfate (HS), a highly negatively charged linear polysaccharide that is attached to a small set of membrane or extracellular matrix proteoglycans.
他のウイルスは、ヘパラン硫酸 (HS) と相互作用します。HS は、膜または細胞外マトリックスのプロテオグリカンの小セットに付着した、非常に負に帯電した線状多糖です。
In general, glycan-binding domains on membrane proteins of the virion envelope mediate initial attachment of virions to glycan receptors.
一般的に、ウイルス粒子エンベロープの膜タンパク質上のグリカン結合ドメインは、ウイルス粒子のグリカン受容体への最初の付着を媒介します。
Attachment in this way can lead to the engagement of protein receptors on the host plasma membrane that facilitate membrane fusion or engulfment and internalization of the virion.
このように付着すると、宿主の細胞膜上のタンパク質受容体が関与し、ウイルス粒子の膜融合または取り込みと内部化が促進されます。
Like other macromolecules, HS can be divided into subunits, which are operationally defined as disaccharides based on the ability of bacterial enzymes or nitrous acid to cleave the chain into disaccharide units.
他の高分子と同様に、HS はサブユニットに分けられ、細菌酵素または亜硝酸が鎖を二糖単位に切断する能力に基づいて、二糖として操作的に定義されます。
The basic disaccharide subunit consists of β1–4 linked d-glucuronic acid (GlcA) and α1–4 linked N-acetyl-d-glucosamine (GlcNAc), which undergo various modifications by sulfation and epimerization as the copolymer assembles on a limited number of membrane and extracellular matrix proteins (only 17 HS proteoglycans are known).
基本的な二糖サブユニットは、β1–4 結合の d-グルクロン酸 (GlcA) と α1–4 結合の N-アセチル-d-グルコサミン (GlcNAc) で構成され、コポリマーが限られた数の膜タンパク質と細胞外マトリックスタンパク質 (17 種類の HS プロテオグリカンのみが知られています) 上に組み立てられると、硫酸化とエピマー化によってさまざまな修飾を受けます。
The variable length of the modified domains and their pattern of sulfation create unique motifs to which HS-binding proteins (HSBPs) interact.
修飾ドメインの可変長と硫酸化パターンにより、HS 結合タンパク質 (HSBP) が相互作用する独自のモチーフが作成されます。
Different tissues and cell types vary in the structure of HS, and HS structure can vary between individuals and with age.
組織や細胞の種類によって HS の構造は異なり、HS の構造は個人間や年齢によっても異なります。
These differences in HS composition may contribute to the tissue tropism and/or host susceptibility to infection by viruses and other pathogens.
HS 組成のこれらの違いは、組織向性や、ウイルスやその他の病原体による感染に対する宿主の感受性に寄与している可能性があります。
In this report, we show that the ectodomain of the SARS-CoV-2 spike (S) protein interacts with cell surface HS through the receptor-binding domain (RBD) in the S1 subunit.
このレポートでは、SARS-CoV-2 スパイク (S) タンパク質のエクトドメインが、S1 サブユニットの受容体結合ドメイン (RBD) を介して細胞表面 HS と相互作用することを示しています。
Binding of heparin to SARS-CoV-2 S protein shifts the structure to favor the RBD open conformation that binds ACE2.
ヘパリンが SARS-CoV-2 S タンパク質に結合すると、構造が変化し、ACE2 に結合する RBD オープン構造が有利になります。
S binding to cells requires engagement of both cellular HS and ACE2, suggesting that HS acts as a coreceptor priming the S for ACE2 interaction.
S が細胞に結合するには、細胞 HS と ACE2 の両方が関与する必要があるため、HS は S を ACE2 と相互作用させる共受容体として機能していることが示唆されます。
Therapeutic unfractionated heparin (UFH), non-anticoagulant heparin, and HS derived from human lung and other tissues block binding.
治療用未分画ヘパリン (UFH)、非抗凝固ヘパリン、およびヒトの肺やその他の組織に由来する HS は結合をブロックします。
UFH and heparin lyases also block infection of cells by S protein pseudotyped virus and authentic SARS-CoV-2.
UFH およびヘパリンリアーゼは、S タンパク質擬似型ウイルスおよび本物の SARS-CoV-2 による細胞感染もブロックします。
These findings identify cellular HS as a necessary co-factor for SARS-CoV-2 infection and emphasizes the potential for targeting S protein-HS interactions to attenuate virus infection.
これらの発見は、細胞内のHSがSARS-CoV-2感染に必要な補因子であることを確認し、Sタンパク質とHSの相互作用を標的にしてウイルス感染を弱める可能性を強調しています。
Results
Molecular Modeling Reveals an HS-Binding Site Adjacent to the ACE2-Binding Domain in the SARS-CoV-2 Spike Protein
分子モデリングにより、SARS-CoV-2スパイクタンパク質のACE2結合ドメインに隣接するHS結合部位が明らかに
The trimeric S proteins from SARS-CoV-1 and SARS-CoV-2 viruses are thought to engage human ACE2 with one or more RBDs in an “open” active conformation (Figure 1A).
SARS-CoV-1 および SARS-CoV-2 ウイルスの三量体 S タンパク質は、「開いた」活性構造の1 つ以上の RBD でヒト ACE2 と結合すると考えられています (図 1A)。
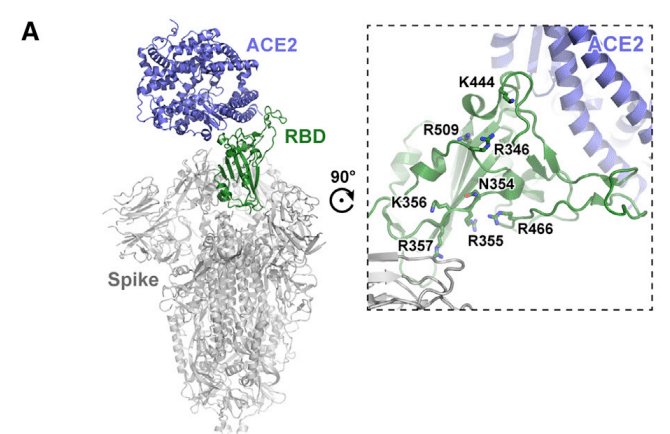
Adjacent to the ACE2-binding site and exposed in the RBD lies a group of positively charged amino acid residues that represents a potential site that could interact with heparin or HS (Figures 1A and S1).
ACE2 結合部位に隣接し、RBD に露出している正電荷を帯びたアミノ酸残基のグループが、ヘパリンまたは HS と相互作用する可能性のある部位を表しています (図 1A および S1)。
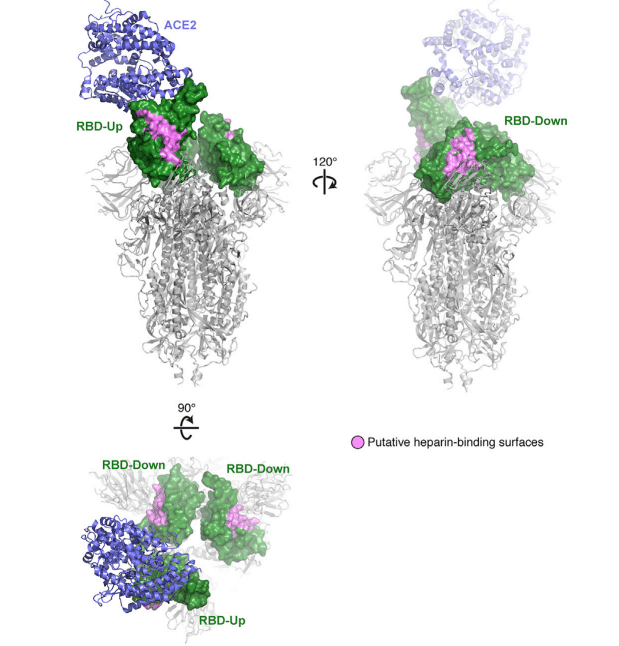
We calculated an electrostatic potential map of the RBD (from PDB ID 6M17), which revealed an extended electropositive surface with dimensions and turns/loops consistent with a heparin-binding site (Figure 1B).
RBD (PDB ID 6M17 より) の静電ポテンシャル マップを計算したところ、ヘパリン結合部位と一致する寸法とターン/ループを持つ拡張された電気陽性表面が明らかになりました (図 1B)。
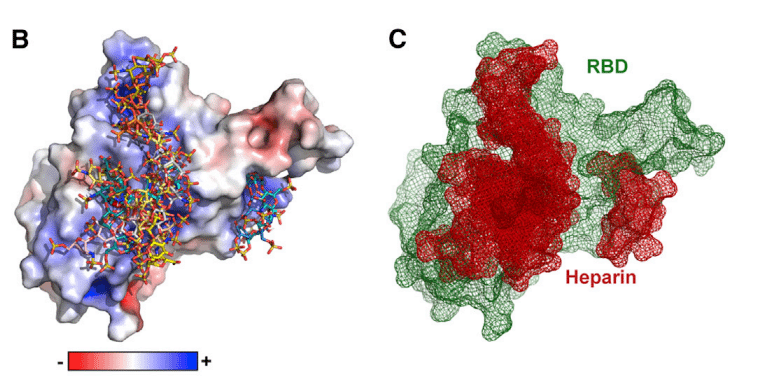
Docking studies using a tetrasaccharide (dp4) fragment derived from heparin demonstrated preferred interactions with this electropositive surface, which, based on its dimensions, could accommodate a chain of up to 20 monosaccharides (Figures 1B and 1C).
ヘパリン由来の四糖類 (dp4) フラグメントを使用したドッキング研究では、この電気陽性表面との優先的な相互作用が実証されました。この表面は、その寸法に基づいて、最大 20 個の単糖鎖を収容できます (図 1B および 1C)。
Evaluation of heparin-protein contacts and energy contributions using the Molecular Operating Environment (MOE) software suggested strong interactions with the positively charged amino acids R346, R355, K444, R466, and possibly R509 (Figures 1A, 1D, and 1E).
分子動作環境 (MOE) ソフトウェアを使用してヘパリンとタンパク質の接触とエネルギー寄与を評価したところ、正に帯電したアミノ酸 R346、R355、K444、R466、およびおそらく R509 との強い相互作用が示唆されました (図 1A、1D、および 1E)。
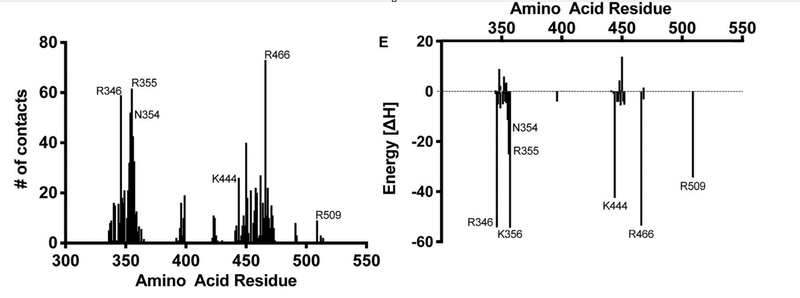
Other amino acids, notably F347, S349, N354, G447, Y449, and Y451, could coordinate the oligosaccharide through hydrogen bonds and hydrophobic interactions.
その他のアミノ酸、特に F347、S349、N354、G447、Y449、および Y451 は、水素結合および疎水性相互作用を介してオリゴ糖を配位する可能性があります。
Notably, the putative binding surface for oligosaccharides is adjacent to, but separate from, the ACE2-binding site, suggesting that a single RBD could simultaneously bind both cell surface HS and the ACE2 protein receptor.
注目すべきことに、オリゴ糖の推定結合表面は ACE2 結合部位に隣接していますが、離れているため、単一の RBD が細胞表面の HS と ACE2 タンパク質受容体の両方に同時に結合できることが示唆されます。
The putative HS-binding site is partially obstructed in the “closed” inactive RBD conformation while fully exposed in the open state (Figure S1).
推定 HS 結合部位は、「閉じた」不活性 RBD 構造では部分的に遮られていますが、開いた状態では完全に露出しています (図 S1)。
The amino acid sequence of S protein RBD of SARS-CoV-2 S is 73% identical to the RBD of SARS-CoV-1 S (Figure 1F), and these domains are highly similar in structure with an overall Cα RMSD of 0.929 Å (Figure 1G).
SARS-CoV-2 S の S タンパク質 RBD のアミノ酸配列は、SARS-CoV-1 S の RBD と 73% 同一であり (図 1F)、これらのドメインは構造的に非常に類似しており、全体的な Cα RMSD は 0.929 Å です (図 1G)。
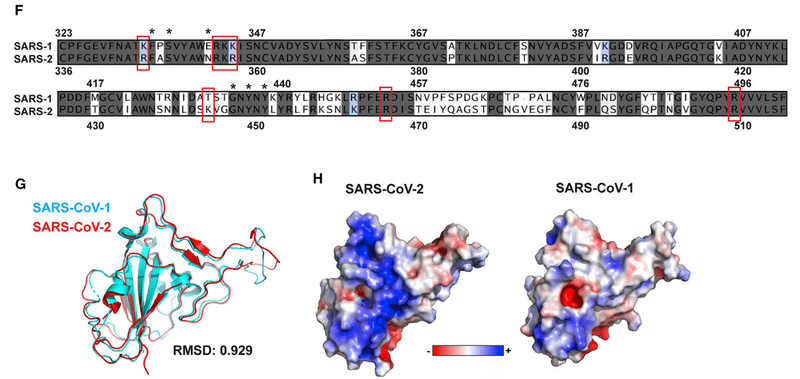
However, an electrostatic potential map of the SARS-CoV-1 S RBD does not show an electropositive surface like that observed in SARS-CoV-2 (Figure 1H).
ただし、SARS-CoV-1 S RBD の静電ポテンシャル マップでは、SARS-CoV-2 で観察されるような電気陽性表面は示されません (図 1H)。
Most of the positively charged residues comprising this surface are conserved between the two proteins, with the exception of SARS-CoV-2 K444, which is a threonine in SARS-CoV-1 (Figure 1F).
この表面を構成する正電荷残基のほとんどは、SARS-CoV-1 のトレオニンである SARS-CoV-2 K444 を除いて、2 つのタンパク質間で保存されています (図 1F)。
Additionally, the other amino acid residues predicted to coordinate with the oligosaccharide are conserved with the exception of Asn354 in SARS-CoV-2, which is a negatively charged glutamate residue in SARS-CoV-1.
さらに、オリゴ糖と協調すると予測される他のアミノ酸残基は、SARS-CoV-2 の Asn354 を除いて保存されています。これは、SARS-CoV-1 の負に帯電したグルタミン酸残基です。
SARS-CoV-1 has been shown to interact with cellular HS in addition to its entry receptors ACE2 and transmembrane protease, serine 2 (TMPRSS2).
SARS-CoV-1 は、エントリー受容体 ACE2 および膜貫通プロテアーゼ、セリン 2 (TMPRSS2) に加えて、細胞 HS と相互作用することが示されています。
Our analysis suggests that the putative heparin-binding site in SARS-CoV-2 S may mediate an enhanced interaction with heparin or HS compared to SARS-CoV-1, and that this change evolved through as few as two amino acid substitutions, Thr→Lys444 and Glu→Asn354.
私たちの分析は、SARS-CoV-2 Sの推定ヘパリン結合部位が、SARS-CoV-1と比較してヘパリンまたはHSとの強化された相互作用を媒介する可能性があり、この変化は、Thr→Lys444およびGlu→Asn354というわずか2つのアミノ酸置換によって進化したことを示唆しています。
The SARS-CoV-2 Spike Protein Binds Heparin through the RBD
SARS-CoV-2スパイクタンパク質はRBDを介してヘパリンに結合
To test experimentally if the SARS-CoV-2 S protein interacts with heparin/HS, recombinant ectodomain and RBD proteins were prepared and characterized.
SARS-CoV-2 Sタンパク質がヘパリン/HSと相互作用するかどうかを実験的にテストするために、組み換えエクトドメインおよびRBDタンパク質を調製し、特性評価しました。
Initial studies encountered difficulty in stabilizing the S ectodomain protein, a problem that was resolved by raising the concentration of NaCl to 0.3 M in HEPES (4-(2-hydroxyethyl)-1-piperazineethanesulfonic acid) buffer.
初期の研究では、Sエクトドメインタンパク質を安定化させることが困難でしたが、HEPES(4-(2-ヒドロキシエチル)-1-ピペラジンエタンスルホン酸)緩衝液中のNaCl濃度を0.3 Mに上げることで問題は解決しました。
Under these conditions, the protein could be stored at room temperature, 4°C, or at −80°C for at least 2 weeks. SDS-PAGE showed that each protein was ∼98% pure (Figure S2A).
これらの条件下では、タンパク質は室温、4°C、または-80°Cで少なくとも2週間保存できました。 SDS-PAGEでは、各タンパク質が約98%純粋であることが示されました(図S2A)。
Transmission electron micrographs of the S ectodomains revealed trimeric structures (Figure S2B).
Sエクトドメインの透過型電子顕微鏡写真では、三量体構造が明らかになりました(図S2B)。
The main component by size exclusion chromatography behaved as a glycosylated trimer with an apparent molecular mass of ∼740 kDa (Figure S2C).
サイズ排除クロマトグラフィーによる主成分は、見かけの分子量が約740 kDaのグリコシル化三量体として挙動しました(図S2C)。
A highly purified commercial preparation of RBD protein was used in some studies (SINO Biologics, Figure S2A), as well as recombinant RBD protein expressed in ExpiHEK cells (Figure S2D), both of which were judged >98% pure by SDS-PAGE and size exclusion chromatography (Figure S2E).
いくつかの研究では、RBD タンパク質の高度に精製された市販の製剤 (SINO Biologics、図 S2A) と、ExpiHEK 細胞で発現した組み換え RBD タンパク質 (図 S2D) が使用されました。どちらも SDS-PAGE およびサイズ排除クロマトグラフィーによって 98% を超える純度であると判断されました (図 S2E)。
Recombinant S ectodomain and RBD proteins were applied to a column of heparin-Sepharose.
組み換え S 細胞外ドメインおよび RBD タンパク質をヘパリン-セファロースカラムに適用しました。
Elution with a gradient of sodium chloride showed that the RBD eluted at ∼0.3 M NaCl, with a shoulder that eluted with higher salt (Figure 2A).
塩化ナトリウムの勾配による溶出では、RBD が約 0.3 M NaCl で溶出され、より高い塩濃度で溶出するショルダーが見られました (図 2A)。
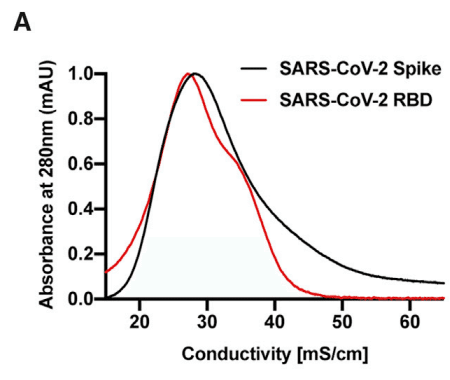
Recombinant S ectodomain also bound to heparin-Sepharose, but it eluted across a broader concentration of NaCl.
組み換え S 細胞外ドメインもヘパリン-セファロースに結合しましたが、NaCl のより広い濃度で溶出しました。
The elution profiles suggest that the preparations contained a population of molecules that bind to heparin, but that some heterogeneity in affinity for heparin occurs, which may reflect differences in glycosylation, oligomerization, or the number of binding sites in the open conformation.
溶出プロファイルから、調製物にはヘパリンに結合する分子の集団が含まれていましたが、ヘパリンに対する親和性に多少の不均一性が生じていることが示唆されています。これは、グリコシル化、オリゴマー化、またはオープン構造の結合部位の数の違いを反映している可能性があります。
The RBD protein from SARS-CoV-2 also bound in a saturable manner to heparin-BSA immobilized on a plate (Figure 2B).
SARS-CoV-2 由来の RBD タンパク質も、プレート上に固定されたヘパリン-BSA に飽和状態で結合しました (図 2B)。
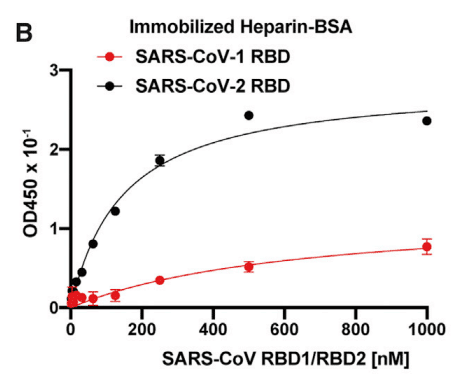
The RBD from SARS-CoV-1 showed significantly reduced binding to heparin-BSA and a higher KD value (640 nM [95% CI; 282–1852 nM] for SARS-CoV-1 RBD versus 150 nM [95% CI; 123–173 nM]) for SARS-CoV-2 RBD), in accordance with the difference in electropositive potential in the proposed HS-binding regions (Figure 1H).
SARS-CoV-1 由来の RBD は、ヘパリン-BSA への結合が大幅に減少し、KD 値が高くなりました (SARS-CoV-1 RBD では 640 nM [95% CI; 282–1852 nM]、SARS-CoV-2 RBD では 150 nM [95% CI; 123–173 nM])。これは、提案された HS 結合領域の電気陽性電位の差と一致しています (図 1H)。
A monomeric form of SARS-CoV-2 S ectodomain protein also bound in a saturable manner to heparin immobilized on a plate (Figure S3A).
SARS-CoV-2 Sエクトドメインタンパク質の単量体も、プレート上に固定されたヘパリンに飽和状態で結合しました(図S3A)。
The trimeric protein bound to heparin-BSA with an apparent KD value of 3.8 nM (95% CI; 3.1–4.6 nM) (Figure 2C).
三量体タンパク質は、見かけのKD値が3.8 nM(95%CI; 3.1~4.6 nM)でヘパリン-BSAに結合しました(図2C)。
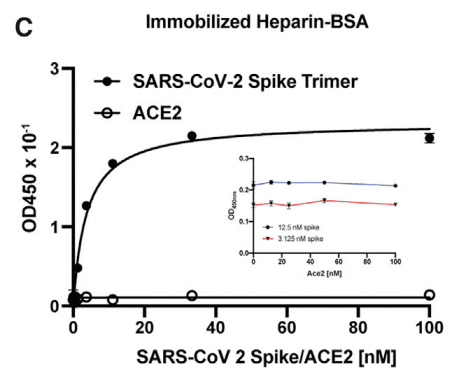
Binding of recombinant S ectodomain, mutated to lock the RBDs into a closed (Mut2), or one that favors an open (Mut7) conformation, showed that the heparin-binding site in the RBD is accessible in both conformations (Figure 2D).
RBD を閉じた構造 (Mut2) または開いた構造 (Mut7) にロックするように変異させた組み換え S 細胞外ドメインの結合により、RBD のヘパリン結合部位はどちらの構造でもアクセス可能であることが示されました (図 2D)。
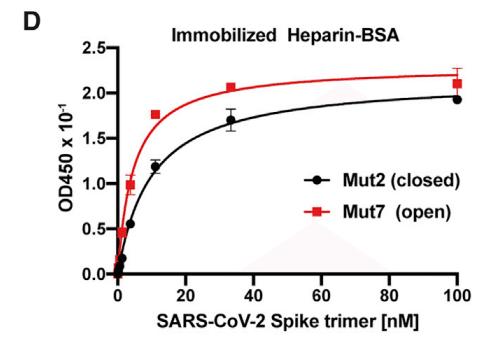
However, the KD value for Mut7 is lower (4.6 nM [95% CI; 3.8–5.5 nM] versus 9.9 nM [95% CI; 8.7–11.3 nM] for Mut2), which is in line with the partial obstruction of the site in the closed conformation (Figure S1).
しかし、Mut7 の KD 値は低く (4.6 nM [95% CI; 3.8–5.5 nM] に対して Mut2 は 9.9 nM [95% CI; 8.7–11.3 nM])、これは閉じた構造における部位の部分的な閉塞と一致しています (図 S1)。
As expected, only S trimers with an open RBD conformation bound to ACE2 (Figure 2E).
予想どおり、開いた RBD 構造を持つ S 三量体のみが ACE2 に結合しました (図 2E)。
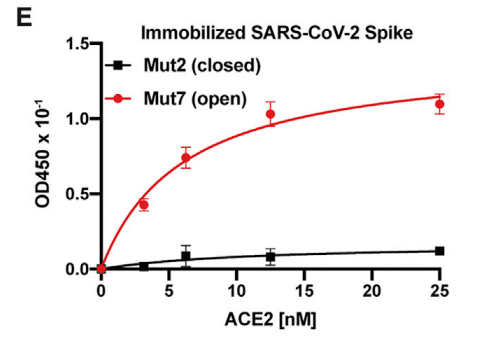
In contrast to S protein, ACE2 did not bind to heparin-BSA (Figure 2C).
S タンパク質とは対照的に、ACE2 はヘパリン BSA に結合しませんでした (図 2C)。
ACE2 also had no effect on binding of S protein to heparin-BSA at all concentrations that were tested (Figure 2C, inset).
ACE2 は、テストしたすべての濃度で S タンパク質のヘパリン BSA への結合にも影響を与えませんでした (図 2C、挿入図)。
Biotinylated ACE2 bound to immobilized S protein (Figure S3B), and a ternary complex of heparin, ACE2, and S protein could be demonstrated by titration of S protein bound to immobilized heparin-BSA with ACE2 (Figure 2F).
ビオチン化 ACE2 は固定化 S タンパク質に結合し (図 S3B)、固定化ヘパリン BSA に結合した S タンパク質を ACE2 で滴定すると、ヘパリン、ACE2、および S タンパク質の三元複合体が実証されました (図 2F)。
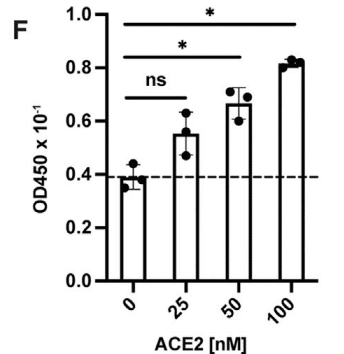
Binding of ACE2 under these conditions increased in proportion to the amount of S protein bound to the heparin-BSA.
これらの条件下での ACE2 の結合は、ヘパリン BSA に結合した S タンパク質の量に比例して増加しました。
Collectively, these findings show that (1) S protein can engage both heparin and ACE2 simultaneously and (2) that the heparin-binding site is somewhat occluded in the closed conformation, but it can still bind heparin, albeit with reduced affinity.
これらの結果を総合すると、(1) S タンパク質はヘパリンと ACE2 の両方に同時に結合できること、(2) ヘパリン結合部位は閉じた構造で多少閉塞していますが、親和性は低下しているものの、ヘパリンに結合できることが示されています。
SARS-CoV-2 Protein Binding to Heparin Increases ACE2 Occupancy of RBDs
SARS-CoV-2タンパク質のヘパリンへの結合によりRBDのACE2占有率が上昇
The simultaneous binding of ACE2 to S protein and heparin suggested the possibility that heparin binding might affect the conformation of the RBD, possibly increasing the open conformation that can bind ACE2.
ACE2 が S タンパク質とヘパリンに同時に結合したことから、ヘパリン結合が RBD の構造に影響を与え、ACE2 に結合できるオープン構造が増加する可能性が示唆されました。
To explore this possibility, S protein was mixed with ACE2 (6-fold molar ratio) with or without dp20 oligosaccharides derived from heparin (9-fold molar ratio).
この可能性を調べるために、S タンパク質を ACE2 (6 倍のモル比) と混合し、ヘパリン由来の dp20 オリゴ糖 (9 倍のモル比) の有無で混合しました。
The samples were then stained and analyzed by transmission electron microscopy, and the images were deconvoluted and sorted into 3D reconstructions to determine the number of trimers with zero, one, two, or three bound ACE2 (Figures 2G, 2H, S3C, and S3D).
その後、サンプルを染色して透過型電子顕微鏡で分析し、画像をデコンボリューションして 3D 再構成画像に分類し、結合した ACE2 が 0、1、2、または 3 個である三量体の数を判定しました (図 2G、2H、S3C、および S3D)。
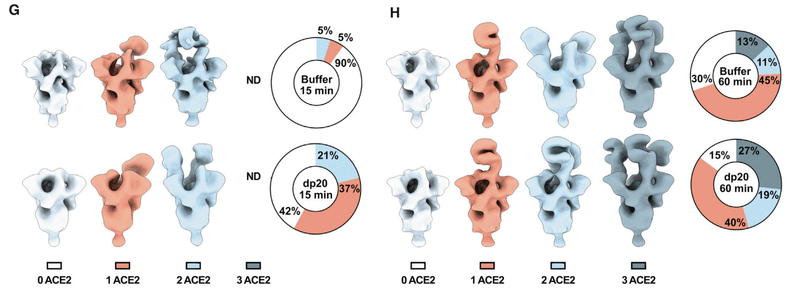
The different populations were counted and the percentage of particles belonging to each 3D class was calculated.
さまざまな集団を数え、各 3D クラスに属する粒子の割合を計算しました。
Two time points were evaluated after mixing ACE2 and trimeric S: at 15 min, 29,600 and 31,300 particles were analyzed in the absence or presence of dp20 oligosaccharides, respectively; at 60 min, 17,000 and 21,000 particles were analyzed in absence or presence of dp20 oligosaccharides, respectively.
ACE2 と三量体 S を混合した後、2 つの時点を評価しました:15 分後には、dp20 オリゴ糖の有無でそれぞれ 29,600 個と 31,300 個の粒子が分析されました;60 分後には、dp20 オリゴ糖の有無でそれぞれ 17,000 個と 21,000 個の粒子が分析されました。
At both time points, the presence of dp20 increased the total amount of ACE2 protein bound to S (Figures 2G and 2H).
どちらの時点でも、dp20 の存在により、S に結合した ACE2 タンパク質の総量が増加しました (図 2G および 2H)。
After 15 min in the absence of dp20, very few of the trimers had conformations with one or two bound ACE2 (5% each), whereas the inclusion of dp20 oligosaccharides greatly increased the proportion of trimers bearing one (37%) or two (21%) ACE2, with a proportional drop in the unbound conformers from 90% in the absence of heparin to 42% in its presence (Figure 2G).
dp20 が存在しない状態で 15 分経過すると、1 つまたは 2 つの ACE2 が結合したコンフォメーションを持つ三量体はほとんどなくなりました (それぞれ 5%)。一方、dp20 オリゴ糖を含めると、1 つ (37%) または 2 つ (21%) の ACE2 を含む三量体の割合が大幅に増加し、結合していないコンフォメーションの割合は、ヘパリンが存在しない状態では 90% でしたが、存在する状態では 42% に減少しました (図 2G)。
Extending the incubation to 60 min resulted in a mixture of trimers containing one (45%), two (11%), and three ACE2 (13%) in the absence of heparin. Inclusion of dp20 further increased the proportion of bound S trimers bearing two (19%) and three (27%) ACE2 (Figure 2H).
インキュベーションを 60 分に延長すると、ヘパリンなしで 1 個 (45%)、2 個 (11%)、3 個 (13%) の ACE2 を含む三量体の混合物ができました。dp20 を含めると、2 個 (19%) と 3 個 (27%) の ACE2 を含む結合 S 三量体の割合がさらに増加しました (図 2H)。
The imaging studies suggest that, under these experimental conditions, heparin may stabilize the ACE2 interaction, increasing the proportion of S bound to ACE2 as well as the occupancy of individual S proteins.
イメージング研究は、これらの実験条件下では、ヘパリンが ACE2 相互作用を安定化し、ACE2 に結合した S の割合と個々の S タンパク質の占有率を増加させる可能性があることを示唆しています。
The SARS-CoV-2 Spike Protein Depends on Cellular Heparan Sulfate for Cell Binding
SARS-CoV-2スパイクタンパク質は細胞結合に細胞内ヘパラン硫酸を必要とする
To extend these studies to HS on the surface of cells, S ectodomain protein was added to human H1299 cells, an adenocarcinoma cell line derived from type 2 alveolar cells (Figure 3A).
これらの研究を細胞表面の HS にまで広げるために、S 細胞外ドメインタンパク質を、2 型肺胞細胞由来の腺癌細胞株であるヒト H1299 細胞に加えました (図 3A)。
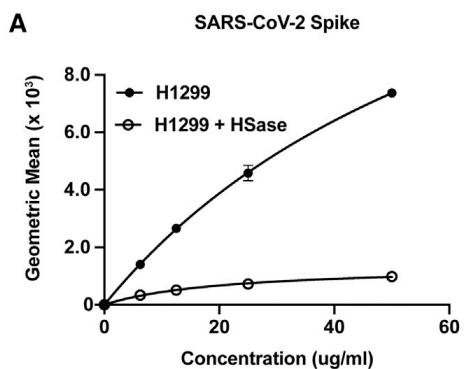
S ectodomains bound to H1299 cells, with half-maximal binding achieved at ∼75 nM.
S 細胞外ドメインは H1299 細胞に結合し、結合の半分は 75 nM で達成されました。
Treatment of the cells with a mixture of heparin lyases (HSase), which degrades cell surface HS, dramatically reduced binding (Figure 3A).
細胞表面の HS を分解するヘパリンリアーゼ (HSase) の混合物で細胞を処理すると、結合が大幅に減少しました (図 3A)。
The S ectodomain also bound to human A549 cells, another type 2 alveolar adenocarcinoma line, as well as human hepatoma Hep3B cells (Figure 3B).
S 細胞外ドメインは、ヒト A549 細胞、別の 2 型肺胞腺癌細胞株、およびヒト肝癌 Hep3B 細胞にも結合しました (図 3B)。
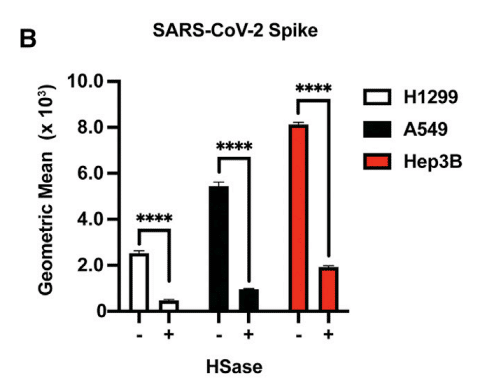
Removal of HS by enzymatic treatment dramatically reduced binding in both of these cell lines as well (Figure 3B).
酵素処理による HS の除去により、これらの細胞株の両方での結合も大幅に減少しました (図 3B)。
Recombinant RBD protein also bound to all three cell lines dependent on HS (Figure 3C).
組み換え RBD タンパク質も、HS に依存して 3 つの細胞株すべてに結合しました (図 3C)。
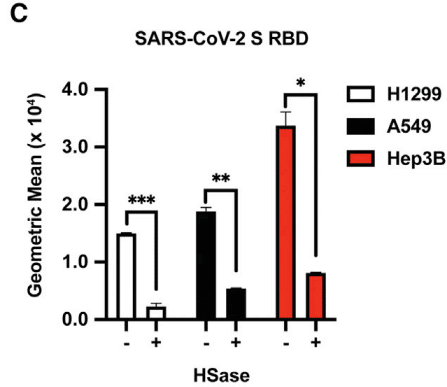
A melanoma cell line, A375, was tested independently and also showed HS-dependent binding (Figure 3D).
黒色腫細胞株 A375 を個別にテストしたところ、HS 依存の結合が見られました (図 3D)。
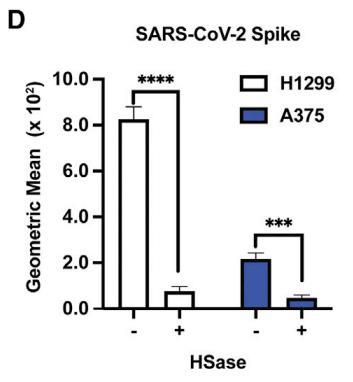
The extent of binding across the four cell lines varied ∼4-fold.
4 つの細胞株間の結合の程度は、約 4 倍異なりました。
This variation was not due to differences in HS expression as illustrated by staining of cell surface HS with mAb 10E4, which recognizes a common epitope in HS (Figure 3E).
この違いは、HS の共通エピトープを認識する mAb 10E4 による細胞表面 HS の染色で示されるように、HS 発現の違いによるものではありません (図 3E)。
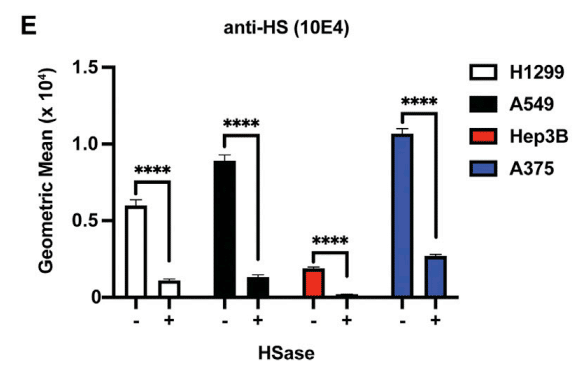
In fact, A375 cells have the highest expression of cell surface HS but the lowest extent of S protein binding (Figure 3D), whereas Hep3B cells have low amounts of cell surface HS and the highest binding of S protein.
実際、A375 細胞は細胞表面 HS の発現が最も高いものの、S タンパク質の結合度合いが最も低く (図 3D)、Hep3B 細胞は細胞表面 HS の量が少なく、S タンパク質の結合度合いが最も高い。
This lack of correlation could reflect differences in the structure of HS or the level of expression of ACE2.
この相関関係の欠如は、HS の構造または ACE2 の発現レベルの違いを反映している可能性がある。
We also measured binding of the S ectodomain and RBD proteins to a library of mutant Hep3B cells, carrying CRISPR-Cas9-induced mutations in biosynthetic enzymes essential for synthesizing HS.
また、HS 合成に必須の生合成酵素に CRISPR-Cas9 誘導変異を導入した変異 Hep3B 細胞のライブラリへの S 細胞外ドメインおよび RBD タンパク質の結合も測定しました。
Inactivation of EXT1, a subunit of the copolymerase required for synthesis of the backbone of HS, abolished binding to a greater extent than enzymatic removal of the chains with HSases (Figures 3F and S4), suggesting that the HSase treatment may underestimate the dependence on HS.
HS 骨格の合成に必要なコポリマーのサブユニットである EXT1 の不活性化により、HSase による鎖の酵素除去よりも結合が大きく消失しました (図 3F および S4)。これは、HSase 処理が HS への依存を過小評価している可能性があることを示唆しています。
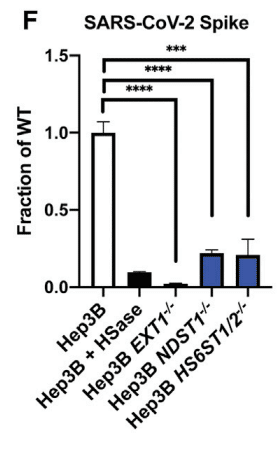
Targeting NDST1, a GlcNAc N-deacetylase-N-sulfotransferase that N-deacetylates and N-sulfates N-acetylglucosamine residues, and HS6ST1 and HS6ST2, which introduces sulfate groups in the C6 position of glucosamine residues, significantly reduced binding (Figures 3F and S4).
N-アセチルグルコサミン残基を N-脱アセチル化および N-硫酸化する GlcNAc N-脱アセチル化酵素-N-硫酸基転移酵素である NDST1 と、グルコサミン残基の C6 位置に硫酸基を導入する HS6ST1 および HS6ST2 を標的とすると、結合が大幅に減少しました (図 3F および S4)。
Although experiments with other sulfotransferases have not yet been done, the data suggest that the pattern of sulfation of HS affects binding to S and RBD.
他の硫酸基転移酵素を用いた実験はまだ行われていませんが、データは HS の硫酸化パターンが S および RBD への結合に影響を与えることを示唆しています。
Heparin and Heparan Sulfates Inhibit Binding of Spike Ectodomain Protein
ヘパリンとヘパラン硫酸はスパイクエクトドメインタンパク質の結合を阻害
To further examine how variation in HS structure affects binding, we isolated HS from human kidney, liver, lung, and tonsil.
HS 構造の多様性が結合にどのような影響を与えるかをさらに調べるため、ヒトの腎臓、肝臓、肺、扁桃腺から HS を分離しました。
The samples were depolymerized into disaccharides by treatment with HSases, and the disaccharides were then analyzed by LC-MS/MS.
サンプルは HSase 処理によって二糖類に脱重合され、その後二糖類は LC-MS/MS によって分析されました。
The disaccharide analysis showed that lung HS has a larger proportion of N-deacetylated and N-sulfated glucosamine residues (gray bars) and more 2-O-sulfated uronic acids (green bars) than HS preparations from the other tissues (Figure 4A).
二糖類分析により、肺の HS は他の組織からの HS 調製物よりも N-脱アセチル化および N-硫酸化グルコサミン残基 (灰色のバー) の割合が高く、2-O-硫酸化ウロン酸 (緑色のバー) が多いことがわかりました (図 4A)。
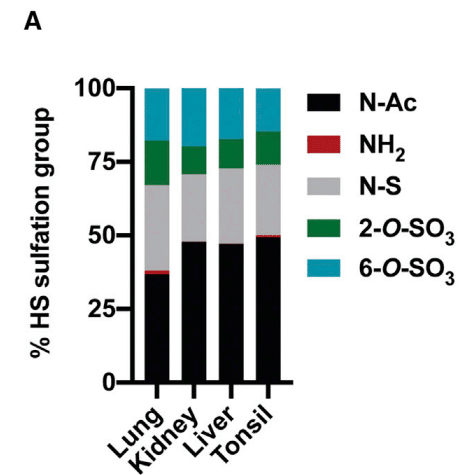
The different HS preparations also varied in their ability to block binding of RBD to H1299 cells (Figure 4B).
HS 調製物によって、RBD の H1299 細胞への結合を阻害する能力も異なります (図 4B)。
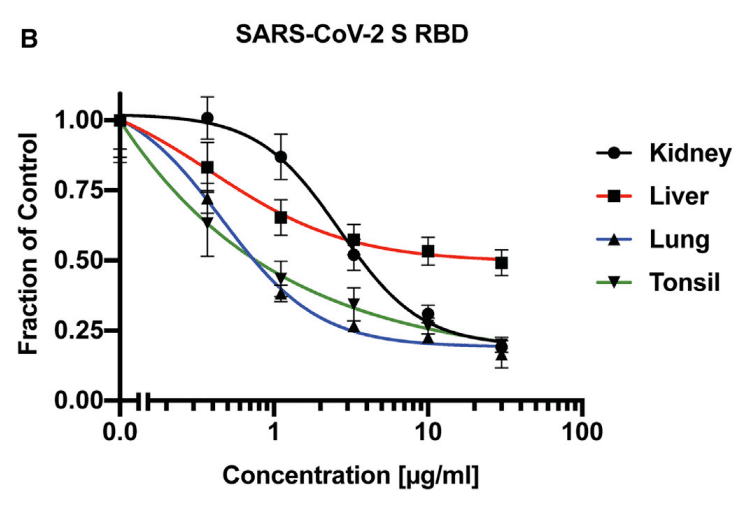
Interestingly, HS isolated from lung was more potent compared to kidney and liver HS, consistent with the greater degree of sulfation of HS from this organ (Table 1).
興味深いことに、肺から分離された HS は腎臓および肝臓の HS と比較して強力であり、この臓器からの HS の硫酸化度が高いことと一致しています (表 1)。
HS from tonsil was as potent as HS from lung, but the overall extent of sulfation was not as great, supporting the notion that the patterning of the sulfated domains in the chains may affect binding.
扁桃腺からの HS は肺からの HS と同程度の効力がありましたが、全体的な硫酸化の程度はそれほど大きくなく、鎖内の硫酸化ドメインのパターンが結合に影響を与える可能性があるという考えを裏付けています。
UFH is derived from porcine mucosa and possesses potent anticoagulant activity due to the presence of a pentasaccharide sequence containing a crucial 3-O-sulfated N-sulfoglucosamine unit, which confers high-affinity binding to antithrombin.
UFH はブタの粘膜から得られ、重要な 3-O-硫酸化 N-スルホグルコサミン単位を含む五糖配列の存在により、強力な抗凝固活性を有し、抗トロンビンへの高親和性結合を付与します。
Heparin is also very highly sulfated compared to HS, with an average negative charge of –3.4 per disaccharide (the overall negative charge density of typical HS is –1.8 to –2.2 per disaccharide).
ヘパリンは HS と比較して非常に高度に硫酸化されており、平均負電荷は二糖あたり –3.4 です (一般的な HS の全体的な負電荷密度は二糖あたり –1.8 ~ –2.2 です)。
MST cells, which were derived from a murine mastocytoma, make heparin-like HS that lacks the key 3-O-sulfate group and anticoagulant activity.
マウス肥満細胞腫から得られた MST 細胞は、重要な 3-O-硫酸基と抗凝固活性を欠くヘパリン様 HS を生成します。
The anticoagulant properties of heparin can also be removed by periodate oxidation, which oxidizes the vicinal hydroxyl groups in the uronic acids, resulting in what is called “split-glycol” heparin.
ヘパリンの抗凝固特性は、過ヨウ素酸酸化によっても除去できます。過ヨウ素酸酸化はウロン酸の隣接ヒドロキシル基を酸化し、いわゆる「スプリットグリコール」ヘパリンを生成します。
All of these agents significantly inhibited binding of the S protein to H1299 and A549 cells (Figures 4C and 4D) yielding IC50 values in the range of 0.01–0.12 μg/mL (Table 1).
これらの薬剤はすべて、S タンパク質の H1299 細胞および A549 細胞への結合を著しく阻害し (図 4C および 4D)、IC50 値は 0.01~0.12 μg/mL の範囲でした (表 1)。
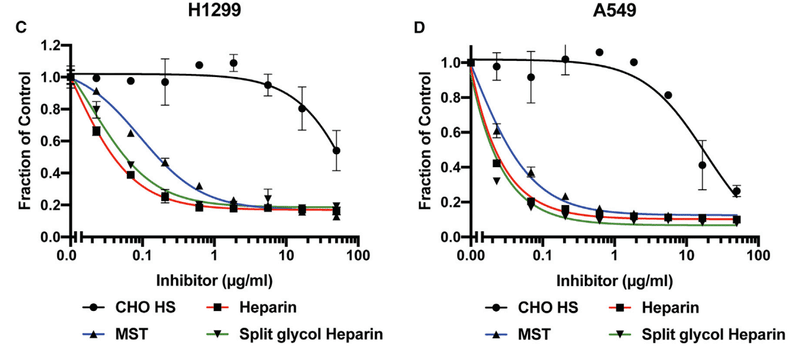
Interestingly, the lack of 3-O-sulfation, crucial for the anticoagulant activity of heparin, had little effect on its inhibition of S binding.
興味深いことに、ヘパリンの抗凝固活性に重要な 3-O-硫酸化の欠如は、S 結合の阻害にほとんど影響しませんでした。
In contrast, CHO cell HS (containing 0.8 sulfates per disaccharide) only weakly inhibited binding (IC50 values of 18 and 139 μg/mL for A549 and H1299, respectively) (Table 1).
対照的に、CHO 細胞 HS (二糖あたり 0.8 硫酸塩を含む) は、結合をわずかに阻害しただけです (A549 および H1299 の IC50 値はそれぞれ 18 μg/mL および 139 μg/mL) (表 1)。
These data suggest that inhibition by heparinoids is most likely charge dependent and independent of anticoagulant activity per se.
これらのデータは、ヘパリン類似物質による阻害は電荷に依存し、抗凝固活性自体とは無関係である可能性が高いことを示唆しています。
Binding of Spike Protein to ACE2 Also Depends on Heparan Sulfate
スパイクタンパク質のACE2への結合もヘパラン硫酸に依存
The experiments shown in Figures 2G and 2H indicate that binding of heparin to S protein can increase binding to ACE2.
図 2G および 2H に示す実験は、ヘパリンが S タンパク質に結合すると ACE2 への結合が増加することを示しています。
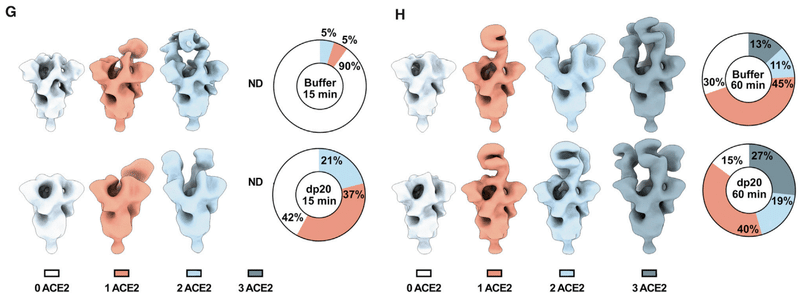
To explore if HS, ACE2, and S interact at the cell surface, we investigated the impact of ACE2 expression on S protein cell binding.
HS、ACE2、および S が細胞表面で相互作用するかどうかを調べるために、ACE2 発現が S タンパク質細胞結合に与える影響を調査しました。
Initial attempts were made to measure ACE2 levels by western blotting or flow cytometry with different mAbs and polyclonal antibodies, but a reliable signal was not obtained in any of the cell lines tested (A375, A549, H1299, and Hep3B).
最初は、さまざまな mAbs およびポリクローナル抗体を使用してウェスタンブロッティングまたはフローサイトメトリーで ACE2 レベルを測定する試みが行われました。しかし、テストしたどの細胞株 (A375、A549、H1299、および Hep3B) でも信頼性の高いシグナルは得られませんでした。
Nevertheless, expression of ACE2 mRNA was observed by qRT-PCR (Figure S5A).
それにもかかわらず、ACE2 mRNA の発現は qRT-PCR によって観察されました (図 S5A)。
Transfection of A375 cells with ACE2 cDNA resulted in robust expression of ACE2 (Figure 5A), resulting in an increase in S ectodomain protein binding by ∼4-fold (Figure 5B).
A375 細胞に ACE2 cDNA をトランスフェクションすると、ACE2 が強力に発現し (図 5A)、S 細胞外ドメインタンパク質結合が約 4 倍増加しました (図 5B)。
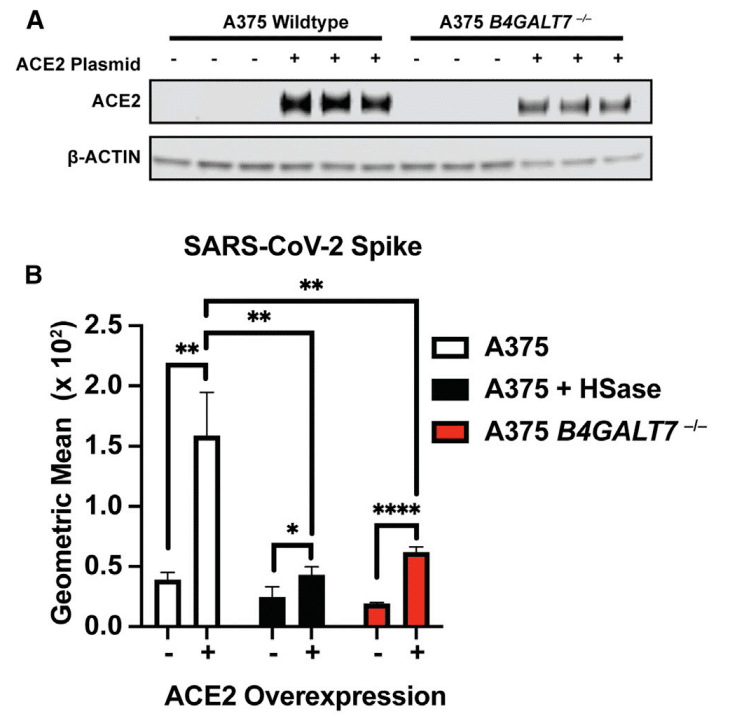
Interestingly, the enhanced binding was HS dependent, as illustrated by the loss of binding of S protein after HSase treatment (Figure 5B).
興味深いことに、HSase 処理後の S タンパク質の結合の喪失によって示されるように、結合の強化は HS に依存していました (図 5B)。
CRISPR-Cas9-mediated deletion of the B4GALT7 gene, which is required for glycosaminoglycan assembly (Figure S5B), also reduced binding of S protein (Figure 5B) despite the overexpression of ACE2 (Figure 5A).
グリコサミノグリカンの組み立てに必要な B4GALT7 遺伝子の CRISPR-Cas9 による削除 (図 S5B) も、ACE2 の過剰発現 (図 5A) にもかかわらず、S タンパク質の結合を減少させました (図 5B)。
To explore the impact of diminished ACE2 expression, we examined S protein binding to A549 cells and in two CRISPR-Cas9 gene-targeted clones, C3 and C6, bearing biallelic mutations in ACE2 (Figure S5C).
ACE2 発現の減少の影響を調べるために、A549 細胞への S タンパク質の結合と、ACE2 に両対立遺伝子変異を持つ 2 つの CRISPR-Cas9 遺伝子標的クローン (C3 および C6) を調べました (図 S5C)。
Binding of S ectodomain protein was greatly reduced in the ACE2−/− clones, and the residual binding was sensitive to HSases (Figure 5C).
ACE2−/− クローンでは S 細胞外ドメイン タンパク質の結合が大幅に減少し、残りの結合は HSase に敏感でした (図 5C)。
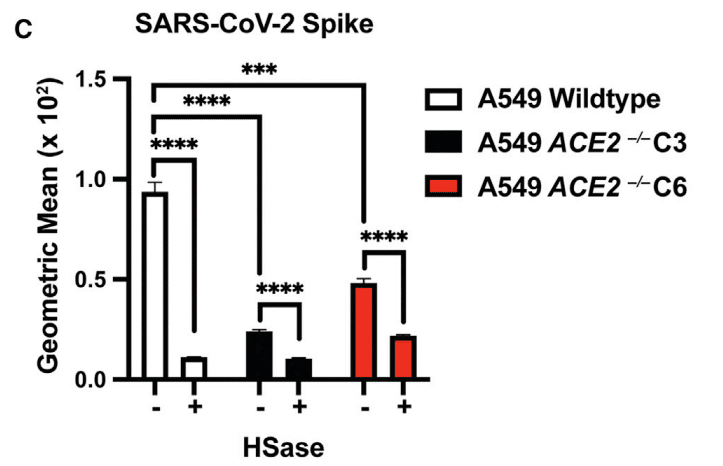
These findings show that binding of S protein on cells requires both HS and ACE2, consistent with the formation of a ternary complex (Figures 2F–2H).
これらの結果は、細胞上の S タンパク質の結合には HS と ACE2 の両方が必要であることを示し、三元複合体の形成と一致しています (図 2F~2H)。
Vesicular Stomatitis Virus Spike Protein Pseudotyped Virus Infection Depends on Heparan Sulfate
水疱性口内炎ウイルススパイクタンパク質擬似ウイルス感染はヘパラン硫酸に依存
Assays using purified components provide biochemical insights into binding, but they do not recapitulate the multivalent presentation of the S protein as it occurs on the virion membrane.
精製された成分を使用したアッセイは、結合に関する生化学的知見を提供しますが、ウイルス粒子膜上で起こる S タンパク質の多価提示を再現するものではありません。
Thus, to extend these studies, pseudotyped vesicular stomatitis virus (VSV) was engineered to express the full-length SARS-CoV-2 S protein and GFP or luciferase to monitor infection.
したがって、これらの研究を拡張するために、擬似型水疱性口内炎ウイルス (VSV) を操作して、感染を監視するために全長 SARS-CoV-2 S タンパク質と GFP またはルシフェラーゼを発現させました。
Vero E6 cells are commonly used in the study of SARS-CoV-2 infection due to their high susceptibility to infection.
Vero E6 細胞は感染に対する感受性が高いため、SARS-CoV-2 感染の研究でよく使用されます。
S protein binding to Vero cells also depends on cellular HS, as binding was sensitive to HSases, heparin, and split-glycol heparin (Figure 6A).
Vero 細胞への S タンパク質の結合も細胞 HS に依存しており、結合は HSase、ヘパリン、およびスプリットグリコールヘパリンに敏感でした (図 6A)。
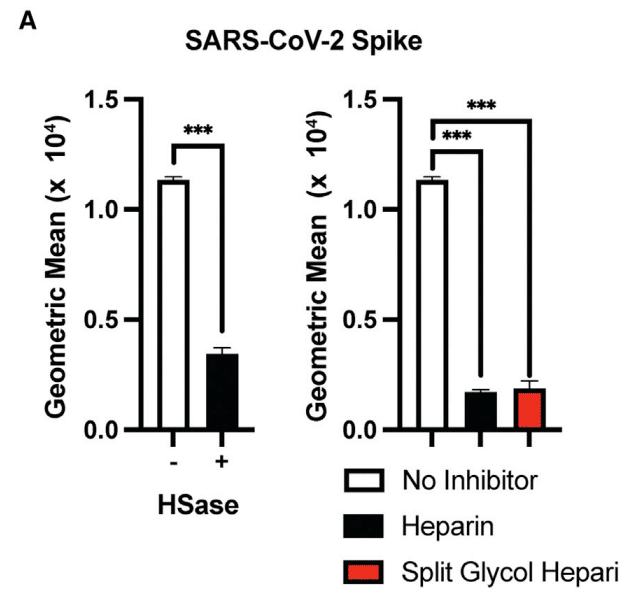
Interestingly, HSase treatment reduced binding to a lesser extent than the level of reduction observed in A549, H1299, and Hep3B cells (Figure 3).
興味深いことに、HSase 処理による結合の減少は、A549、H1299、および Hep3B 細胞で観察された減少レベルよりも低かった (図 3)。
The decrease in sensitivity to HSase may be due to the very high levels of ACE2 expression in comparison to other cells (western blotting, Figure 6B; qRT-PCR, Figure S5A).
HSase に対する感度の低下は、他の細胞と比較して ACE2 の発現レベルが非常に高いためである可能性がある (ウェスタンブロッティング、図 6B、qRT-PCR、図 S5A)。
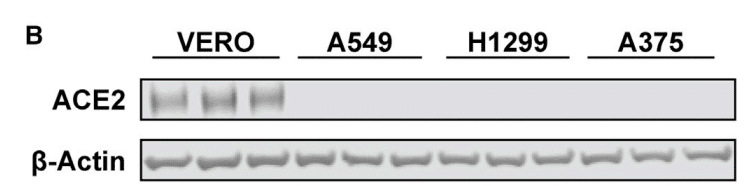
Infection of Vero cells by GFP-expressing VSV S protein pseudotyped virus was readily assessed qualitatively by fluorescence microscopy (inset) and quantitatively by flow cytometry (Figures 6C and 6D).
GFP 発現 VSV S タンパク質擬似型ウイルスによる Vero 細胞の感染は、蛍光顕微鏡 (挿入図) による定性的な評価とフローサイトメトリーによる定量的な評価が容易に可能であった (図 6C および 6D)。
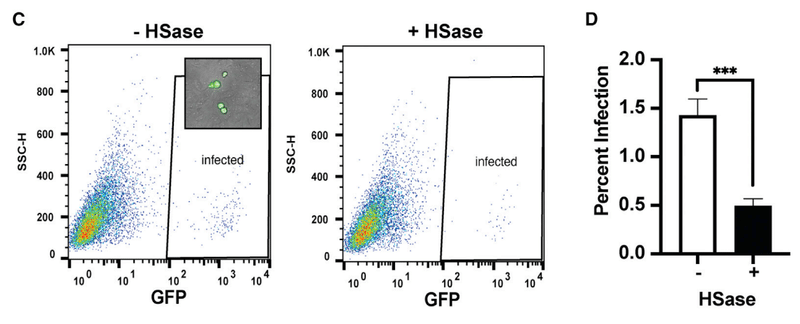
HSase treatment reduced infection ∼3-fold.
HSase 処理により感染が約 3 倍減少しました。
Infection by luciferase-expressing VSV S protein pseudotyped virus provided greater sensitivity, allowing studies with both high and low infection rates (Figures 6E and 6F).
ルシフェラーゼ発現VSV Sタンパク質擬似型ウイルスによる感染は感度が高く、感染率が高い場合と低い場合の両方の研究が可能になりました(図6Eおよび6F)。
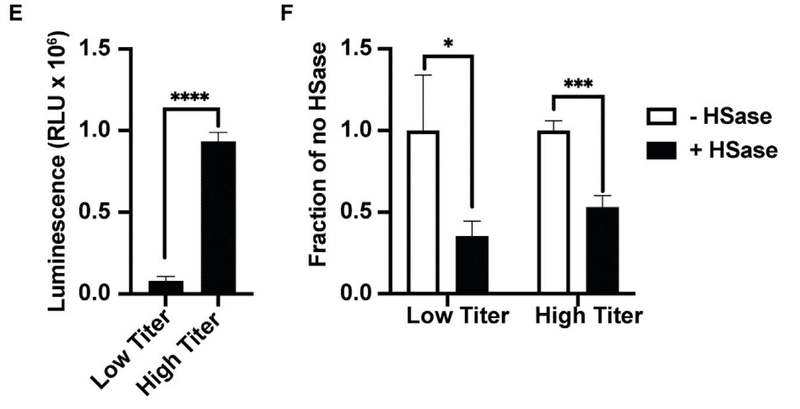
Under either condition, infection was reduced 2- to 3-fold by HSase. Heparin very potently reduced infection more than ∼4-fold at 0.5 μg/mL and higher concentrations (Figure 6G).
どちらの条件でも、HSaseによって感染は2~3倍減少しました。ヘパリンは0.5μg/mL以上の濃度で感染を約4倍以上も非常に強力に減少させました(図6G)。
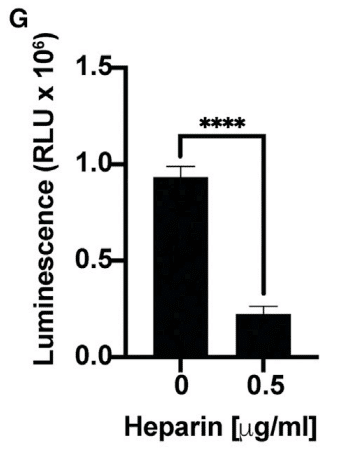
In contrast, studies of SARS-CoV-1 S protein pseudotyped virus showed that HSase-treatment actually increased SARS-CoV-1 infection by more than 2-fold, suggesting that HS might interfere with binding of SARS-CoV-1 in this cell line (Figure 6H).
対照的に、SARS-CoV-1 Sタンパク質擬似型ウイルスの研究では、HSase処理によりSARS-CoV-1感染が実際に2倍以上増加したことが示されており、HSがこの細胞株におけるSARS-CoV-1の結合を妨害する可能性があることを示唆しています(図6H)。
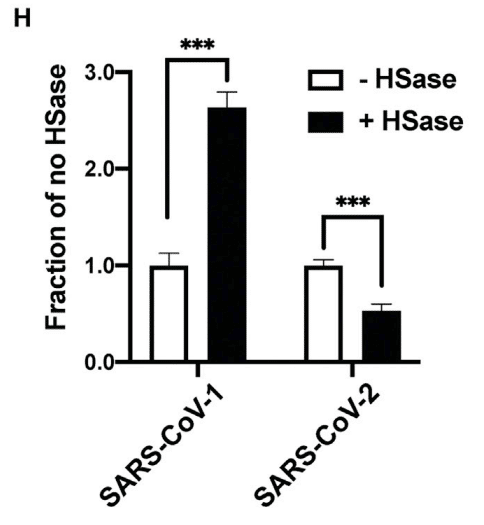
Infection of H1299 and A549 cells by SARS-CoV-2 S pseudotyped virus was too low to obtain accurate measurements, but infection of Hep3B cells could be readily measured (Figure 6I).
SARS-CoV-2 S 擬似型ウイルスによる H1299 細胞および A549 細胞の感染は低すぎて正確な測定はできなかったが、Hep3B 細胞の感染は容易に測定できた (図 6I)。
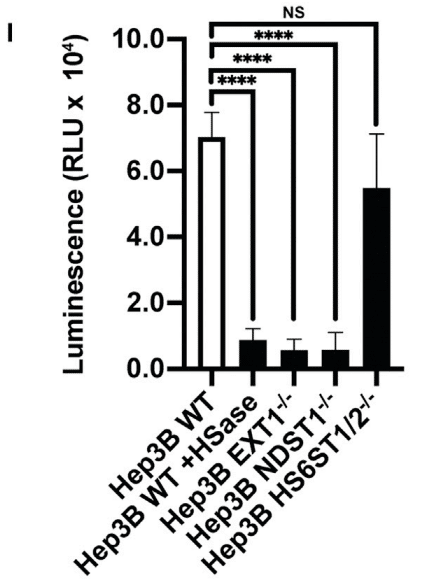
HSase and mutations in EXT1 and NDST1 dramatically reduced infection 6- to 7-fold.
HSase と EXT1 および NDST1 の変異により、感染は 6 ~ 7 倍劇的に減少しました。
Inactivation of the 6-O-sulfotransferases had only a mild effect unlike its strong effect on S protein binding (Figure 3F), possibly due to the high valency conferred by multiple copies of S protein on the pseudovirus envelope.
6-O-硫酸基転移酵素の不活性化は、S タンパク質結合に対する強力な効果とは異なり、軽度の効果しかありませんでした (図 3F)。これは、擬似ウイルスエンベロープ上の S タンパク質の複数のコピーによって付与された高い結合価によるものと考えられます。
Hep3B cells were not susceptible to infection by SARS-CoV-1 S protein pseudotyped virus but was infected by MERS-CoV S protein pseudotyped virus, and infection was independent of HS (Figure S6).
Hep3B 細胞は、SARS-CoV-1 S タンパク質擬似型ウイルスによる感染には感受性がありませんでしたが、MERS-CoV S タンパク質擬似型ウイルスには感染し、感染は HS とは無関係でした (図 S6)。
Cellular Heparan Sulfate Is Required for Efficient Infection by Authentic SARS-CoV-2 Virus
細胞内のヘパラン硫酸は本物のSARS-CoV-2ウイルスの効率的な感染に必要である
Studies of pseudovirus were then extended to authentic SARS-CoV-2 virus infection using strain USA-WA1/2020.
その後、擬似ウイルスの研究は、株 USA-WA1/2020 を使用した本物の SARS-CoV-2 ウイルス感染にまで拡大されました。
Infection of Vero E6 cells was monitored by double staining of the cells with antibodies against the SARS-CoV-2 nucleocapsid (N) and S proteins (Figure 7A).
Vero E6 細胞の感染は、SARS-CoV-2 ヌクレオカプシド (N) および S タンパク質に対する抗体で細胞を二重染色することによって監視されました (図 7A)。
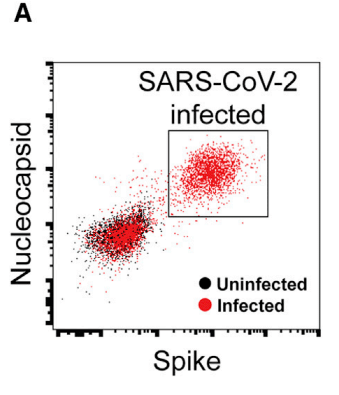
Cells were treated with virus for 1 h and the extent of infection was assayed one day later.
細胞をウイルスで 1 時間処理し、感染の程度を 1 日後に検査しました。
Varying the MOI (0.2–0.5) yielded infection rates that ranged from 15%–50%.
MOI (0.2~0.5) を変化させると、感染率は 15%~50% の範囲になりました。
Treatment of the cells with HSases caused, on average, a ∼5-fold reduction in the percentage of infected cells.
HSase による細胞処理により、感染細胞の割合が平均で約 5 分の 1 に減少しました。
Composite data from five separate experiments done in triplicate are shown in Figure 7B (color coded for individual experiments).
3 回繰り返して行われた 5 つの個別の実験からの複合データを図 7B に示します (個々の実験は色分けされています)。
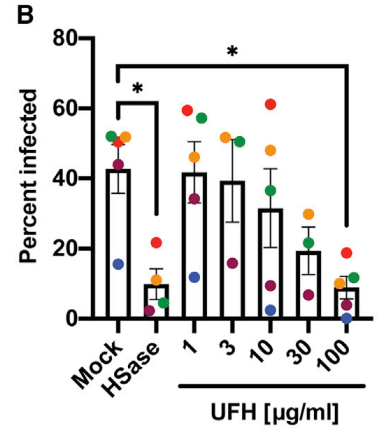
Data normalized to the values obtained in the absence of any treatment (mock) is shown in Figure 7C.
処理なし (モック) で得られた値に正規化されたデータを図 7C に示します。
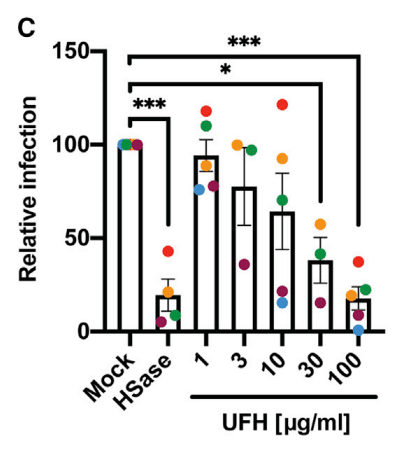
Titration of UFH showed dose-dependent inhibition of infection (Figures 7B and 7C), with cells infected at a lower MOI showing greater sensitivity to heparin inhibition (maroon and blue symbols).
UFH の滴定により、用量依存的な感染阻害が示され (図 7B および 7C)、低い MOI で感染した細胞はヘパリン阻害に対する感受性が高まった (えび茶色と青色の記号)。
To rule out that the treatments caused a decrease in ACE2 expression or a reduction in cell viability, Vero cells were treated with heparin lyases and 100 μg/mL UFH, and ACE2 expression was measured by western blotting and cell viability by CellTiter-Blue (Figures S7A and S7B).
処理によって ACE2 発現が減少したり、細胞生存率が低下したりした可能性を排除するために、Vero 細胞をヘパリンリアーゼと 100 μg/mL UFH で処理し、ACE2 発現をウェスタンブロッティングで、細胞生存率を CellTiter-Blue で測定した (図 S7A および S7B)。
No effect on ACE2 expression or cell viability was observed.
ACE2 発現または細胞生存率への影響は観察されなかった。
These findings further emphasize the potential for using UFH or other non-anticoagulant heparinoids to prevent viral attachment.
これらの知見は、UFH または他の非抗凝固性ヘパリン類似物質を使用してウイルス付着を予防できる可能性をさらに強調している。
These findings were then extended to Hep3B cells and mutants altered in HS biosynthesis using a viral plaque assay.
これらの発見は、ウイルスプラークアッセイを使用して、Hep3B細胞とHS生合成が変化した変異体にまで拡張されました。
Virus was added to wild-type, NDST1−/−, and HS6ST1/2−/− cells for 2 h, the virus was removed, and after 2 days incubation, a serial dilution of the conditioned culture medium was added to monolayers of Vero E6 cells.
ウイルスを野生型、NDST1−/−、およびHS6ST1/2−/−細胞に2時間添加し、ウイルスを除去し、2日間培養した後、調整培養培地の連続希釈物をVero E6細胞の単層に添加しました。
The number of plaques was then quantitated by staining and visualization.
次に、プラークの数を染色と可視化によって定量しました。
As a control, culture medium from infected Vero E6 cells was tested, which showed robust viral titers.
対照として、感染したVero E6細胞の培養培地をテストしたところ、強力なウイルス力価が示されました。
Hep3B cells also supported viral replication, but to a lesser extent than Vero cells.
Hep3B細胞もウイルス複製をサポートしましたが、Vero細胞ほどではありませんでした。
Inactivation of NDST1 in Hep3B cells abolished virus production, whereas inactivation of HS6ST1/2−/− reduced infection more mildly, ∼3-fold (Figure 7D).
Hep3B細胞でNDST1を不活性化するとウイルス産生が消失しましたが、HS6ST1/2−/−を不活性化すると感染がより穏やかに、約3倍減少しました(図7D)。
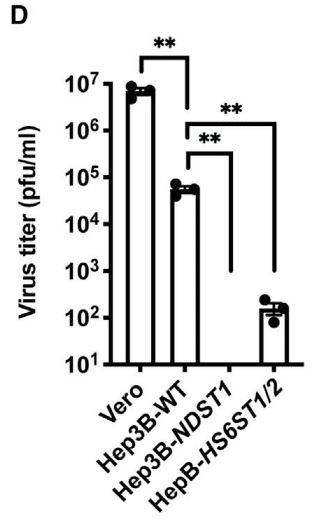
Finally, to explore the role of cellular HS in SARS-CoV-2 infection of primary human bronchial epithelial cells, cells were grown at an air-liquid interface and infected with authentic SARS-CoV-2 virus with and without prior HSase treatment or addition of UFH.
最後に、初代ヒト気管支上皮細胞の SARS-CoV-2 感染における細胞 HS の役割を調べるために、細胞を気液界面で増殖させ、HSase 処理の有無や UFH の添加の有無にかかわらず、本物の SARS-CoV-2 ウイルスに感染させました。
Infection was assessed by flow cytometry using antibodies against the viral N protein (Figure 7E).
感染は、ウイルス N タンパク質に対する抗体を使用したフローサイトメトリーによって評価されました (図 7E)。
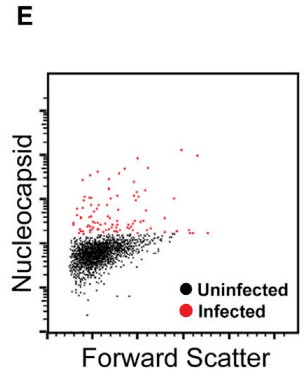
Composite data from three separate experiments each done in triplicate are shown in Figure 7F (again color coded for individual experiments).
それぞれ 3 回ずつ行われた 3 つの別々の実験からの複合データを図 7F に示します (ここでも個々の実験ごとに色分けされています)。
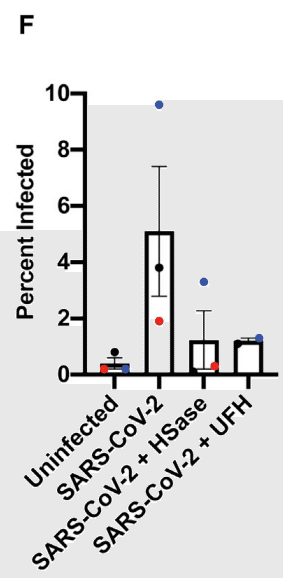
Data normalized to the values obtained in the absence of any treatment (uninfected) is shown in Figure 7G.
処理なし (感染なし) で得られた値に正規化されたデータを図 7G に示します。
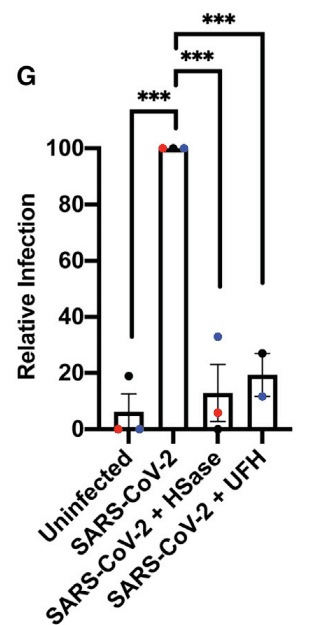
Treatment with HSase and UFH reduced infection more than 5-fold, but it had no effect on cell viability (Figure S7C).
HSase と UFH による処理により、感染は 5 倍以上減少しましたが、細胞生存率には影響がありませんでした (図 S7C)。
These findings demonstrate the requirement of cellular HS in mediating infection of authentic human bronchial epithelial cells by SARS-CoV-2.
これらの結果は、SARS-CoV-2 による本物のヒト気管支上皮細胞の感染を媒介する上で細胞 HS が必要であることを示しています。
Discussion
In this report, we provide compelling evidence that HS is a necessary host attachment factor that promotes SARS-CoV-2 infection of various target cells.
本レポートでは、HS が SARS-CoV-2 のさまざまな標的細胞への感染を促進する必須の宿主付着因子であるという説得力のある証拠を示します。
The RBD of the SARS-CoV-2 S protein binds to heparin/HS, most likely through a docking site composed of positively charged amino acid residues aligned in a subdomain of the RBD that is separate from the site involved in ACE2 binding (Figure 1).
SARS-CoV-2 S タンパク質の RBD は、おそらく ACE2 結合に関与する部位とは別の RBD のサブドメインに整列した正電荷アミノ酸残基で構成されるドッキング部位を介してヘパリン/HS に結合します (図 1)。
Competition studies, enzymatic removal of HS, and genetic studies confirm that the S protein, whether presented as a recombinant protein (Figures 2, 3, 4, and 5) in a pseudovirus (Figure 6) or in authentic SARS-CoV-2 virions (Figure 7), binds to cell surface HS in a cooperative manner with ACE2 receptors.
競合研究、HS の酵素除去、および遺伝学的研究により、S タンパク質は、擬似ウイルス (図 6) 内の組み換えタンパク質 (図 2、3、4、および 5) として存在するか、または本物の SARS-CoV-2 ビリオン (図 7) に存在するかにかかわらず、ACE2 受容体と協調して細胞表面の HS に結合することが確認されています。
Mechanistically, binding of heparin/HS to S trimers enhances binding to ACE2, likely increasing multivalent interactions with the target cell.
メカニズム的には、ヘパリン/HS が S 三量体に結合すると ACE2 への結合が強化され、標的細胞との多価相互作用が増加する可能性があります。
These data provide crucial insights into the pathogenic mechanism of SARS-CoV-2 infection and suggest HS-S protein complexes as a novel therapeutic target to prevent infection.
これらのデータは、SARS-CoV-2 感染の病原性メカニズムに関する重要な洞察を提供し、HS-S タンパク質複合体が感染を防ぐための新しい治療ターゲットであることを示唆しています。
The glycocalyx is the first point of contact for all pathogens that infect animal cells, and thus it is not surprising that many viruses exploit glycans, such as HS, as attachment factors.
グリコカリックスは動物細胞に感染するすべての病原体にとって最初の接触点であるため、多くのウイルスが HS などのグリカンを付着因子として利用するのは当然のことです。
For example, the initial interaction of herpes simplex virus with cells involves binding to HS chains on one or more HS proteoglycans through the interactions with the viral glycoproteins gB and gC.
たとえば、単純ヘルペスウイルスと細胞の最初の相互作用には、ウイルス糖タンパク質 gB および gC との相互作用を通じて、1 つ以上の HS プロテオグリカン上の HS 鎖への結合が含まれます。
Viral entry requires the interaction of a specific structure in HS with a third viral glycoprotein, gD, working in concert with membrane proteins related to TNF/NGF receptors.
ウイルスの侵入には、HS の特定の構造と、TNF/NGF 受容体に関連する膜タンパク質と連携して働く 3 番目のウイルス糖タンパク質 gD との相互作用が必要です。
Similarly, the human immunodeficiency virus binds to HS by way of the V3 loop of the viral glycoprotein gp120, but infection requires the chemokine receptor CCR5.
同様に、ヒト免疫不全ウイルスはウイルス糖タンパク質 gp120 の V3 ループを介して HS に結合しますが、感染にはケモカイン受容体 CCR5 が必要です。
Other coronaviruses also utilize HS; for example, NL63 (HCoV-NL63) binds HS via the viral S protein in addition to ACE2.
他のコロナウイルスも HS を利用します。たとえば、NL63 (HCoV-NL63) は、ACE2 に加えてウイルス S タンパク質を介して HS に結合します。
In these examples, initial tethering of virions to the host cell plasma membrane appears to be mediated by HS, but infection requires transfer to a proteinaceous receptor.
これらの例では、ウイルス粒子の宿主細胞の細胞膜への最初の係留は HS によって媒介されているように見えますが、感染にはタンパク質受容体への移動が必要です。
The data presented here show that SARS-CoV-2 requires HS in addition to ACE2.
ここで提示されたデータは、SARS-CoV-2 には ACE2 に加えて HS が必要であることを示しています。
We imagine a model in which cell surface HS acts as a “collector” of the virus and a mediator of the RBD-ACE2 interaction, making viral infection more efficient.
細胞表面の HS がウイルスの「コレクター」として機能し、RBD-ACE2 相互作用のメディエーターとして機能して、ウイルス感染をより効率的にするというモデルを想像します。
HS varies in structure across cell types and tissues, as well as with gender and age.
HS の構造は、細胞の種類や組織によって異なり、性別や年齢によっても異なります。
Variation in competition by HS from different tissues supports this conclusion and raises the possibility that HS contributes to the tissue tropism and the susceptibility of different patient populations, in addition to levels of expression of ACE2.
異なる組織からの HS の競合の変動は、この結論を裏付けており、ACE2 の発現レベルに加えて、HS が組織向性やさまざまな患者集団の感受性に寄与している可能性を示唆しています。
Coronaviruses can utilize a diverse set of glycoconjugates as attachment factors.
コロナウイルスは、さまざまな複合糖質を付着因子として利用できます。
Human coronavirus OC43 (HCoV-OC43) and bovine coronavirus (BCoV) bind to 5-N-acetyl-9-O-acetylneuraminic acid, middle east respiratory syndrome virus (MERS-CoV) binds to 5-N-acetyl-neuraminic acid, and guinea fowl coronavirus binds biantennary di-N-acetyllactosamine or sialic acid-capped glycans.
ヒトコロナウイルス OC43 (HCoV-OC43) とウシコロナウイルス (BCoV) は 5-N-アセチル-9-O-アセチルノイラミン酸に結合し、中東呼吸器症候群ウイルス (MERS-CoV) は 5-N-アセチルノイラミン酸に結合し、ホロホロチョウコロナウイルスは二分岐ジ-N-アセチルラクトサミンまたはシアリン酸キャップドグリカンに結合します。
Whether SARS-CoV-2 S protein binds to sialic acid remains unclear.
SARS-CoV-2 S タンパク質がシアリン酸に結合するかどうかは不明です。
Mapping the binding site for sialic acids in other coronavirus S proteins has proved elusive, but modeling studies suggest a location distinct from the HS-binding site shown in Figure 1.
他のコロナウイルスの S タンパク質におけるシアリン酸の結合部位のマッピングは困難であることが判明していますが、モデリング研究では、図 1 に示す HS 結合部位とは異なる場所が示唆されています。
The S protein in murine coronavirus contains both a hemagglutinin domain for binding and an esterase domain that cleaves sialic acids that aids in the liberation of bound virions.
マウスコロナウイルスの S タンパク質には、結合用のヘマグルチニンドメインと、結合したウイルス粒子の解放を助けるシアリン酸を切断するエステラーゼドメインの両方が含まれています。
Whether SARS-CoV-2 S protein, another viral envelope protein, or a host protein contributes to HS-degrading activity to aid in the release of newly made virions is unknown.
SARS-CoV-2 の S タンパク質、別のウイルスエンベロープタンパク質、または宿主タンパク質が、新しく作られたウイルス粒子の放出を助ける HS 分解活性に寄与するかどうかは不明です。
The repertoire of proteins in organisms that bind to HS make up the so called “HS interactome” and consists of a variety of different HSBPs.
HS に結合する生物のタンパク質のレパートリーは、いわゆる「HS インタラクトーム」を構成し、さまざまな HSBP で構成されています。
Unlike lectins that have a common fold that helps define the glycan-binding site, HSBPs do not exhibit a conserved motif that allows accurate predictions of binding sites based on primary sequence.
グリカン結合部位を定義するのに役立つ共通の折り畳みを持つレクチンとは異なり、HSBP には、一次配列に基づいて結合部位を正確に予測できる保存されたモチーフがありません。
Instead, the capacity to bind heparin appears to have emerged through convergent evolution by juxtaposition of several positively charged amino acid residues arranged to accommodate the negatively charged sulfate and carboxyl groups present in the polysaccharide, and hydrophobic and H-bonding interactions stabilize the association.
代わりに、ヘパリンに結合する能力は、多糖類に存在する負に帯電した硫酸基とカルボキシル基に対応するように配置されたいくつかの正に帯電したアミノ酸残基の並置による収斂進化を通じて出現したようであり、疎水性および水素結合相互作用が結合を安定化します。
The RBDs from the SARS-CoV-1 and SARS-CoV-2 S proteins are highly similar in structure (Figure 1G), but the electropositive surface in the SARS-CoV-1 S RBD is not as pronounced in the SARS-CoV-2 S RBD (Figure 1H).
SARS-CoV-1 および SARS-CoV-2 S タンパク質の RBD は構造が非常に類似していますが (図 1G)、SARS-CoV-1 S RBD の電気陽性表面は SARS-CoV-2 S RBD ではそれほど顕著ではありません (図 1H)。
In accordance with this observation, recombinant RBD protein from SARS-CoV-2 showed significantly higher binding to heparin-BSA, compared to RBD from SARS-CoV-1 (Figure 2B).
この観察結果によると、SARS-CoV-2 の組み換え RBD タンパク質は、SARS-CoV-1 の RBD と比較して、ヘパリン BSA への結合が著しく高いことが示されました (図 2B)。
A priori, we predicted that the evolution of the HS-binding site in the SARS-CoV-2 S protein might have occurred by the addition of arginine and lysine residues to its ancestor, SARS-CoV-1.
事前に、SARS-CoV-2 S タンパク質の HS 結合部位の進化は、その祖先である SARS-CoV-1 にアルギニンとリジン残基が追加されたことによって起こった可能性があると予測しました。
Instead, we observed that four of the six predicted positively charged residues that make up the heparin-binding site are present in SARS-CoV-1, as well as most of the other amino acid residues predicted to interact with heparin (Figure 1).
代わりに、ヘパリン結合部位を構成する 6 つの予測された正電荷残基のうち 4 つが SARS-CoV-1 に存在すること、およびヘパリンと相互作用すると予測される他のほとんどのアミノ酸残基が存在することを観察しました (図 1)。
SARS-CoV-1 has been shown to interact with cellular HS in addition to its entry receptors ACE2 and transmembrane protease, serine 2 (TMPRSS2).
SARS-CoV-1 は、そのエントリー受容体 ACE2 および膜貫通プロテアーゼ、セリン 2 (TMPRSS2) に加えて、細胞 HS と相互作用することが示されています。
Our analysis suggests that the putative heparin-binding site in SARS-CoV-2 S may mediate an enhanced interaction with heparin compared to SARS-CoV-1 and that this change evolved through as few as two amino acid substitutions, Thr444Lys and Glu354Asn.
私たちの分析は、SARS-CoV-2 S の推定ヘパリン結合部位が、SARS-CoV-1 と比較してヘパリンとの相互作用を強化している可能性があり、この変化はわずか 2 つのアミノ酸置換 (Thr444Lys と Glu354Asn) によって進化したことを示唆しています。
Further studies are underway to define the amino acid residues in the combining site for heparin/HS to test this hypothesis.
この仮説を検証するために、ヘパリン/HS の結合部位のアミノ酸残基を定義するさらなる研究が進行中です。
The ability of heparin and HS to compete for binding of the SARS-CoV-2 S protein to cell surface HS and the inhibitory activity of heparin toward infection of pseudovirus and authentic SARS-CoV-2 illustrates the therapeutic potential of agents that target the virus-HS interaction to control infection and transmission of SARS-CoV-2.
ヘパリンとHSがSARS-CoV-2 Sタンパク質の細胞表面HSへの結合を競合する能力と、ヘパリンの擬似ウイルスおよび本物のSARS-CoV-2の感染に対する阻害活性は、ウイルス-HS相互作用を標的とする薬剤がSARS-CoV-2の感染と伝染を制御する治療の可能性を示しています。
There is precedent for targeting protein-glycan interactions as therapeutic agents.
治療薬としてタンパク質-グリカン相互作用を標的とする前例があります。
For example, Tamiflu targets influenza neuraminidase, thus reducing viral transmission, and sialylated human milk oligosaccharides can block sialic-acid-dependent rotavirus attachment and subsequent infection in infants.
たとえば、タミフルはインフルエンザノイラミニダーゼを標的とするため、ウイルスの伝染を減らし、シアリル化母乳オリゴ糖は乳児におけるシアリン酸依存性ロタウイルスの付着とそれに続く感染を阻止できます。
COVID-19 patients typically suffer from thrombotic complications ranging from vascular micro-thromboses, venous thromboembolic disease, and stroke, and often receive UFH or low molecular weight heparin.
COVID-19患者は通常、血管微小血栓症、静脈血栓塞栓症、脳卒中などの血栓性合併症を患っており、UFHまたは低分子量ヘパリンを投与されることがよくあります。
The findings presented here and elsewhere suggest that both of these agents can block viral infection.
ここで示した知見や他の文献から、これらの薬剤は両方ともウイルス感染を阻止できることが示唆されている。
Effective anticoagulation is achieved with plasma levels of heparin of 0.3–0.7 units/mL.
効果的な抗凝固作用は、ヘパリン血漿濃度が 0.3~0.7 単位/mL のときに達成される。
This concentration is equivalent to 1.6–4 μg/mL heparin (assuming the activity of UFH is 180 units/mg).
この濃度は、ヘパリン 1.6~4 μg/mL に相当します (UFH の活性が 180 単位/mg であると仮定)。
Although this is sufficient to block S protein binding to cells (Figure 4), it would not be expected to prevent viral infection, but it should attenuate infection depending on the viral load (Figure 7).
これは S タンパク質の細胞への結合を阻止するのに十分ですが (図 4)、ウイルス感染を防ぐことは期待できませんが、ウイルス量に応じて感染を弱めるはずです (図 7)。
The anticoagulant activity of heparin, which is typically absent in HS, is not critical for its antiviral activity based on the observation that MST-derived heparin and split-glycol heparin are nearly as potent as therapeutic heparin (Figures 4 and 6).
HS には通常存在しないヘパリンの抗凝固作用は、MST 由来ヘパリンとスプリットグリコールヘパリンは治療用ヘパリンとほぼ同等の効力があるという観察に基づくと、抗ウイルス作用にとって重要ではありません (図 4 および 6)。
Additional studies are needed to address the potential overlap in the dose response profiles for heparin as an anticoagulant and antiviral agent and the utility of non-anticoagulant heparins.
抗凝固剤および抗ウイルス剤としてのヘパリンの用量反応プロファイルの潜在的な重複と、非抗凝固性ヘパリンの有用性に対処するには、追加の研究が必要です。
Antibodies directed to HS or the binding site in the RBD might also prove useful for attenuating infection.
HSまたはRBDの結合部位を標的とした抗体も、感染を弱めるのに役立つ可能性があります。
In conclusion, this work revealed HS as a novel attachment factor for SARS-CoV-2 and suggests the possibility of using HS mimetics, HS degrading lyases, and metabolic inhibitors of HS biosynthesis for the development of therapy to combat COVID-19.
結論として、本研究はHSがSARS-CoV-2の新たな付着因子であることが明らかとなり、HS模倣物、HS分解リアーゼ、およびHS生合成の代謝阻害剤をCOVID-19と戦う治療法の開発に使用できる可能性を示唆している。
以下省略。
この記事が気に入ったらサポートをしてみませんか?