結核菌または SARS-COV-2 への曝露後の初期免疫反応: 類似点と相違点
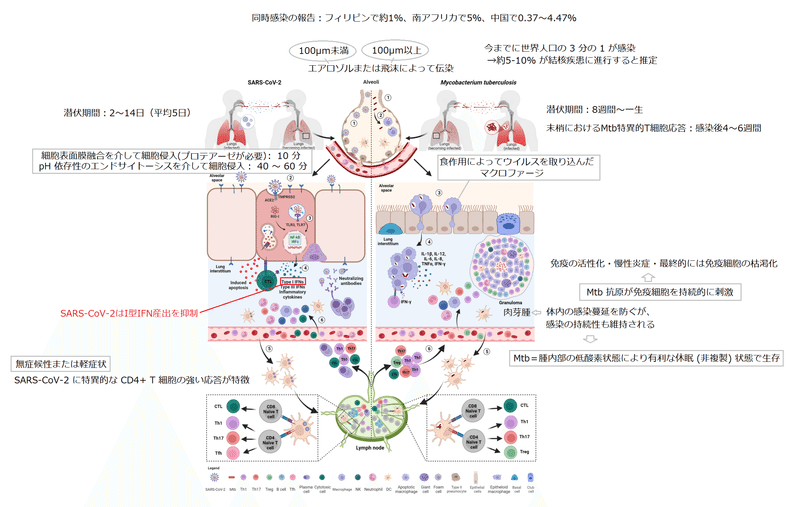
Initial immune response after exposure to Mycobacterium tuberculosis or to SARS-COV-2: similarities and differences
結核菌または SARS-COV-2 への曝露後の初期免疫反応: 類似点と相違点
Tuberculosis (TB), caused by Mycobacterium tuberculosis (Mtb) and Coronavirus disease-2019 (COVID-19), whose etiologic agent is severe acute respiratory syndrome coronavirus-2 (SARS-CoV-2), are currently the two deadliest infectious diseases in humans, which together have caused about more than 11 million deaths worldwide in the past 3 years.
結核菌(Mtb)によって引き起こされる結核(TB)および重症急性呼吸器症候群コロナウイルス-2(SARS-CoV-2)を病原体とするコロナウイルス感染症-2019(COVID-19)は、現在、人類にとって最も致死率の高い 2 つの感染症であり、これらを合わせると過去 3 年間で世界中で約 1,100 万人以上が死亡しています。
TB and COVID-19 share several aspects including the droplet- and aerosol-borne transmissibility, the lungs as primary target, some symptoms, and diagnostic tools.
結核とCOVID-19は、飛沫やエアロゾルによる感染力、主な標的としての肺、いくつかの症状、診断ツールなど、いくつかの側面を共有しています。
However, these two infectious diseases differ in other aspects as their incubation period, immune cells involved, persistence and the immunopathological response.
しかし、これら 2 つの感染症は、潜伏期間、関与する免疫細胞、持続期間、免疫病理学的応答などの他の側面で異なります。
In this review, we highlight the similarities and differences between TB and COVID-19 focusing on the innate and adaptive immune response induced after the exposure to Mtb and SARS-CoV-2 and the pathological pathways linking the two infections.
この総説では、Mtb と SARS-CoV-2 への曝露後に誘発される自然免疫応答と適応免疫応答、および 2 つの感染を結び付ける病理学的経路に焦点を当て、結核と COVID-19 の類似点と相違点に焦点を当てます。
Moreover, we provide a brief overview of the immune response in case of TB-COVID-19 co-infection highlighting the similarities and differences of each individual infection.
さらに、個々の感染症の類似点と相違点を強調しながら、結核とCOVID-19の同時感染の場合の免疫反応の概要を説明します。
A comprehensive understanding of the immune response involved in TB and COVID-19 is of utmost importance for the design of effective therapeutic strategies and vaccines for both diseases.
結核とCOVID-19に関与する免疫応答を包括的に理解することは、両方の疾患に対する効果的な治療戦略とワクチンを設計するために最も重要です。
Introduction
Coronavirus disease-2019 (COVID-19), whose etiologic agent is severe acute respiratory syndrome coronavirus-2 (SARS-CoV-2) and tuberculosis (TB), that is caused by the bacterial pathogen Mycobacterium tuberculosis (Mtb), are the two-leading causes of death from a single infectious agent in humans.
病原体が重症急性呼吸器症候群コロナウイルス-2 (SARS-CoV-2)であるコロナウイルス病-2019 (COVID-19)と、細菌性病原体結核菌(Mtb)によって引き起こされる結核(TB)は、単一の感染性病原体によるヒトの主な死亡原因の 2 つです。
In the past 3 years, SARS-CoV-2 has been responsible for more than 7 million deaths, and Mtb for 4.5 million worldwide.
過去 3 年間で、世界中で SARS-CoV-2 による死者数は 700 万人以上、MTB による死者数は 450 万人を超えています。
SARS-CoV-2 is an enveloped RNA-based single-stranded virus recently emerged belonging to the Betacoronavirus genus.
SARS-CoV-2 は、ベータコロナウイルス属に属し、最近出現したエンベロープ RNA ベースの一本鎖ウイルスです。
The first case of COVID-19 dates back to 2019 in Wuhan, China, and it is thought to be the result of a zoonotic spill-over event that likely occurred from bats and humans and finally caused the global pandemic.
COVID-19の最初の症例は2019年に中国の武漢で発生したもので、おそらくコウモリと人間から発生し、最終的に世界的なパンデミックを引き起こした人獣共通感染症の波及事象の結果であると考えられている。
More than 700 million SARS-CoV-2 infections have been reported worldwide (to date, as of June 2023).
世界中で7億人以上のSARS-CoV-2感染が報告されている(2023年6月現在)。
According to WHO, the largest number of confirmed cases are in Europe, Western Pacific and Americas (Table 1).
WHO によると、確認された感染者数が最も多いのはヨーロッパ、西太平洋、アメリカ大陸です (表 1)。
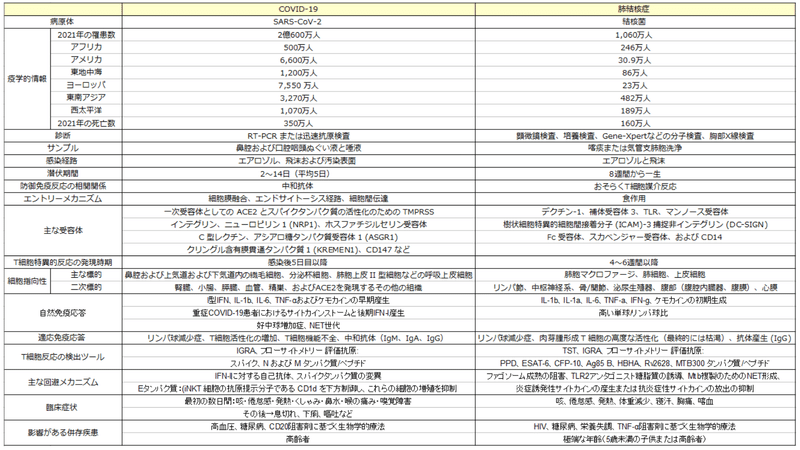
The spread of the virus was probably aided also by the onset of highly mutated forms of SARS-CoV-2, defined as “variants of concern” (VOCs), with enhanced transmission rate and with relatively lower morbidity and mortality compared to the ancestral strain.
ウイルスの蔓延は、おそらく「懸念される変異体」(VOC)として定義される、高度に変異した型の SARS-CoV-2 の発症によっても促進されたと考えられます。VOCは 祖先株と比較して感染率が向上してますが、罹患率と死亡率が比較的低くなっています。
On the contrary, Mtb is an ancient slow growing bacterium that has plagued the human population for thousand years.
一方で、Mtb は、数千年にわたって人類を悩ませてきた古代のゆっくりと増殖する細菌です。
To date, it is estimated that one third of the world population is infected with Mtb, and about 5-10% of the Mtb-exposed and -infected individuals will progress to TB disease.
現在までに、世界人口の 3 分の 1 が Mtb に感染しており、Mtb に曝露および感染した人の約 5 ~ 10% が結核疾患に進行すると推定されています。
In most of them bacilli are detectable in the sputum.
それらのほとんどでは、喀痰から細菌が検出されます。
According to WHO, the largest number of confirmed cases are in Africa and South-Est Asia (Table 1).
WHO によると、確認された感染者数が最も多いのはアフリカと東南アジアです (表 1)。
Although Mtb and SARS-CoV-2 are distinct pathogens, they share several features summarized in Table 1.
Mtb と SARS-CoV-2 は別個の病原体ですが、表 1 にまとめたいくつかの特徴を共有しています。
The main transmission route for both pathogens is via droplets (> 100 µm particles) or aerosols (< 100 µm particles) that are expelled by an ill individual by coughing, sneezing, talking, and breathing.
どちらの病原体も、主な感染経路は、咳、くしゃみ、会話、呼吸によって病気の人の飛沫(粒子が 100 μm 以上)またはエアロゾル(粒子が 100 μm 未満)です。
These particles can travel short distances in the air before being inhaled.
これらの粒子は、吸入される前に空気中を短距離移動する可能性があります。
However, for SARS-CoV-2, the infection can also occur as a result of contact with contaminated surfaces or objects on which virions can persist even for 72 hours.
ただし、SARS-CoV-2 の場合、汚染された表面や物体との接触の結果として感染が発生することもあります。 ビリオンは72時間でも存続することができます。
Regarding Mtb, infection can also occur during autopsies or during the spill of caseus material, i.e. from a scrofula when the cervical tuberculous lymphadenitis drains the material outside.
Mtb に関しては、解剖中や乾酪の物質が流出した際、つまり頸部結核性リンパ節炎により物質が外部に排出された際の陰嚢からの感染も発生する可能性があります。
While SARS-CoV-2 shows a short incubation period (2-14 days) before symptoms onset, in Mtb infection it can range from eight weeks to a lifetime (Table 1 and Figure 1).
SARS-CoV-2 では症状が発現するまでの潜伏期間が短い(2 ~ 14 日)のに対し、Mtb 感染の場合、潜伏期間は 8 週間から生涯にわたる場合があります(表 1 および図 1)。
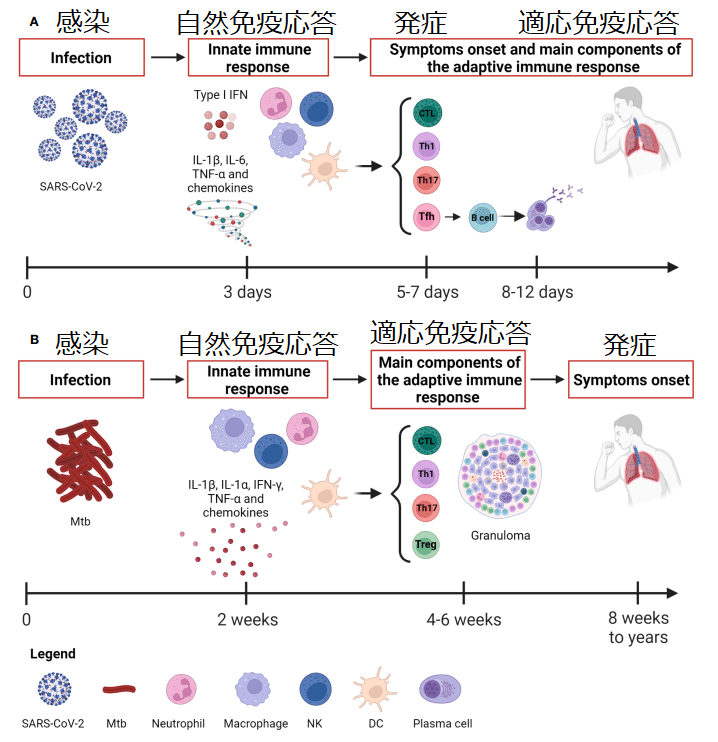
Considering the route of transmission, it is not surprising that both SARS-CoV-2 and Mtb firstly infect the respiratory system causing symptoms such as cough, fatigue and fever.
感染経路を考慮すると、SARS-CoV-2とMtbの両方が最初に呼吸器系に感染し、咳、倦怠感、発熱などの症状を引き起こすことは驚くべきことではありません。
In addition, SARS-CoV-2-infected subjects also experience sneezing, runny nose, sore throat, and anosmia in the first few days followed by shortness of breath, diarrhea, vomiting, etc., whereas in TB patients weight loss, night sweats, chest pain and coughing up of blood were reported.
さらに、SARS-CoV-2に感染した被験者は、最初の数日間はくしゃみ、鼻水、喉の痛み、嗅覚障害を経験し、その後息切れ、下痢、嘔吐などが続きます。 一方、結核患者では、体重減少、寝汗、胸痛、喀血が報告されました。
This similarity in symptoms might make the diagnosis difficult; however, in most cases the COVID-19 symptoms are short-lived compared to those of TB, which has a long incubation with long-lasting symptoms duration.
この症状の類似性により診断が困難になる可能性があります;ただし、ほとんどの場合、COVID-19症状は結核に比べて短期間で済みます。 結核は潜伏期間が長く、症状が長く続く病気です。
Both agents can be detected in respiratory samples such as nasopharyngeal swab or saliva for SARS-CoV-2, and sputum or bronchoalveolar lavage (BAL) for Mtb.
どちらの病原体も、SARS-CoV-2の場合は鼻咽頭ぬぐい液または唾液などの呼吸器サンプル、Mtbの場合は喀痰または気管支肺胞洗浄液(BAL)などで検出できます。
The diagnosis can require different tools.
診断にはさまざまなツールが必要になる場合があります。
For SARS-CoV-2 infection, molecular swab is the first choice in case of suspected symptomatic individuals, contacts of confirmed cases with symptoms and for the screening of health workers.
SARS-CoV-2感染の場合、症状があると疑われる個人、症状のある確定患者との接触者、および医療従事者のスクリーニングの場合、分子綿棒が第一選択となります。
In other contexts, it is recommended to use rapid antigenic tests that are less labor-intensive and costly and can provide results in less than half an hour (Table 1).
他の状況では、労力とコストが少なく、30 分以内に結果が得られる迅速な抗原検査の使用が推奨されます (表 1)。
Regarding Mtb, two main types of tests are used to determine the traditionally called latent infection, now defined “tuberculosis infection”: the tuberculin skin test (TST) and interferon (IFN)-γ release assays (IGRA).
Mtb に関しては、伝統的に潜伏感染と呼ばれ、現在では「結核感染」と定義されている感染を判定するために、ツベルクリン皮膚テスト (TST) とインターフェロン (IFN)-γ 放出アッセイ (IGRA) という 2 つの主要なタイプの検査が使用されます。
For patients with suspected pulmonary TB, the Center for Disease Control (CDC) recommends performing an acid-fast-bacilli smear on three different sputum specimens.
肺結核が疑われる患者に対して、疾病管理センター (CDC) は、3 つの異なる喀痰検体に対して抗酸菌塗抹標本を実施することを推奨しています。
Moreover, Gene-Xpert (Cepheid, Sunnyvale, CA, USA) is a widely accepted diagnostic test for TB detection in direct smear negative cases.
さらに、Gene-Xpert (Cepheid、米国カリフォルニア州サニーベール) は、直接塗抹陰性症例における結核検出用の診断検査として広く受け入れられています。
Notably, SARS-CoV-2 and Mtb-infected individuals show a diverse spectrum of clinical manifestations.
注目すべきことに、SARS-CoV-2およびMtbに感染した人は、多様な臨床症状を示します。
Patients infected with SARS-CoV-2 can experience a clinical outcome ranging from asymptomatic to mild/moderate infection up to severe disease (particularly with Wuhan strain and in those not vaccinated), which can also progress to acute respiratory distress syndrome (ARDS).
SARS-CoV-2に感染した患者は、無症状から軽度/中等度の感染、さらには重篤な疾患(特に武漢株やワクチン接種を受けていない患者)までの臨床転帰を経験する可能性があります。 急性呼吸窮迫症候群(ARDS)に進行する可能性もあります。
Indeed, SARS-CoV-2 can interfere with the host immune system leading to hyperinflammatory state, immune dysregulation, and extensive lung damage.
実際、SARS-CoV-2 は宿主の免疫系に干渉し、過剰炎症状態、免疫調節不全、広範な肺損傷を引き起こす可能性があります。
Differently, Mtb-exposed individuals remain clinically asymptomatic due to the development of an immune response that controls Mtb replication.
これとは異なり、Mtb に曝露された個人は、Mtb の複製を制御する免疫応答の発生により臨床的に無症状のままです。
It has been shown that some individuals heavily exposed to Mtb can clear the infection early before the emergence of the adaptive immune response, can keep a negative score to the TST and IGRA, and therefore do not show any evidence of infection.
Mtb に高度に曝露された一部の個人は、適応免疫応答が出現する前に感染を早期に解消でき、TST および IGRA のマイナススコアを維持できるため、感染の証拠を示さないことが示されています。
The lack of a detectable adaptive immune response in these resistant individuals suggests the key role mediated by the local innate immunity.
これらの耐性を持つ個体には検出可能な適応免疫応答が存在しないことから、局所の自然免疫が重要な役割を果たしていることが示唆されます。
The difficulty of treating and eradicating Mtb is related to the ability of the mycobacteria to survive and replicate within human cells.
Mtb の治療と根絶の難しさは、マイコバクテリアがヒトの細胞内で生存し複製する能力に関係しています。
In both infections, the clinical manifestations may be more severe in presence of comorbidities.
どちらの感染症でも、併存疾患があると臨床症状がより重篤になる可能性があります。
In this regard, they share similar risk factors in terms of comorbidities as advanced age, and diabetes, although they have specific peculiarities as hypertension and biological therapy with CD20 inhibitors for COVID-19 and HIV infection, malnourishment and biological therapy based on TNF-α inhibitors for TB (Table 1).
この点において、彼らは高齢や糖尿病などの併存疾患という点で同様の危険因子を共有しています。ただし、特有の危険因子として、COVID-19およびHIV感染症では高血圧や CD20 阻害剤による生物学的療法、結核では栄養失調やTNF-α阻害剤に基づく生物学的療法があります(表1)。
An effective and timely immune response plays a pivotal role in affecting the clinical course of both COVID-19 and TB.
効果的かつタイムリーな免疫応答は、COVID-19と結核の両方の臨床経過に影響を与える上で極めて重要な役割を果たします。
This review aims to provide an overview of innate and adaptive immune responses induced after the exposure to Mtb and SARS-CoV-2 highlighting the similarities and differences of each individual infection and their crosstalk in TB-COVID-19 co-infection.
このレビューは、Mtb および SARS-CoV-2 への曝露後に誘発される自然免疫応答および適応免疫応答の概要を提供することを目的としており、個々の感染症の類似点と相違点と、結核とCOVID-19の同時感染におけるクロストークを強調しています。
Cell tropism and entry mechanisms
細胞の指向性と侵入メカニズム
Viral entry is the first and pivotal step for the viral life cycle.
ウイルスの侵入は、ウイルスのライフサイクルにとって最初で極めて重要なステップです。
Not surprisingly, blocking virus entry is a primary target of several therapeutic strategies to prevent the subsequent steps and inhibit viral replication and host cell pathology.
当然のことながら、ウイルスの侵入をブロックすることは、その後の段階を阻止し、ウイルスの複製と宿主細胞の病理を阻害するためのいくつかの治療戦略の主な目標です。
Although both SARS-CoV-2 and Mtb are airborne pathogen entering via droplets, and primarily infect the human respiratory system, they differ by cellular tropism and entry mechanisms.
SARS-CoV-2 と Mtb はどちらも飛沫を介して侵入する空気感染性病原体であり、主に人間の呼吸器系に感染しますが、細胞の指向性と侵入メカニズムが異なります。
SARS-CoV-2 and cell tropism and entry mechanisms
SARS-CoV-2 と細胞の向性および侵入メカニズム
SARS-CoV-2 has a broad spectrum of tropism.
SARS-CoV-2 は広範囲の指向性を持っています。
The human angiotensin-converting enzyme 2 (ACE2) represents the major cellular entry point for the virus, thus the expression of ACE2 defines which tissues can be potentially infected by SARS-CoV-2.
ヒト アンジオテンシン変換酵素 2 (ACE2) は、ウイルスの主要な細胞侵入点を表すため、ACE2 の発現によって、どの組織が SARS-CoV-2 に感染する可能性があるかが決まります。
The epithelial cells such as subset of ciliated cells, secretory goblet cells and alveolar epithelial type II cells within the nasal cavity and the upper and lower respiratory tract, represent the primary targets for the initial infection and spread of SARS-CoV-2.
鼻腔および上気道および下気道内の繊毛細胞のサブセット、分泌杯細胞、肺胞上皮 II 型細胞などの上皮細胞は、SARS-CoV-2 の初期感染と拡散の主な標的となります。
In this regard, the higher amount of viral RNA was found in ciliated and epithelial progenitors.
この点に関して、繊毛および上皮前駆細胞ではより多量のウイルス RNA が見つかりました。
Interestingly, although the human respiratory tract is the main target for the virus due to its airborne transmissibility, ACE2 expression in kidneys and gastrointestinal tract is even higher than the lungs.
興味深いことに、空気感染力があるため、ウイルスの主な標的はヒトの気道であるにもかかわらず、腎臓と胃腸管での ACE2 発現は肺よりもさらに高いことです。
Notably, extrapulmonary organs such as the kidneys, small intestines, pancreas, blood vessels, testes and other tissues can be additional targets for SARS-CoV-2, thus explaining the variety of symptoms associated to the infection.
特に、腎臓、小腸、膵臓、血管、精巣、その他の組織などの肺外臓器が、SARS-CoV-2 の追加の標的となる可能性があります。 したがって、感染に関連するさまざまな症状が説明されます。
SARS-CoV-2 gains access to cells mainly through two possible routes, the plasma membrane fusion and the endocytic pathway.
SARS-CoV-2 は、主に原形質膜融合とエンドサイトーシス経路という 2 つの考えられる経路を通じて細胞に侵入します。
The entry route used by the virus is dependent on the expression of cell surface proteases, which are needed for the activation of the viral protein, and it is primarily mediated by the structural protein spike, a trimeric glycoprotein that binds to the ACE2.
ウイルスが使用する侵入経路は、ウイルスタンパク質の活性化に必要な細胞表面プロテアーゼの発現に依存しており、主に構造タンパク質スパイク(ACE2に結合する三量体糖タンパク質)によって媒介されます。
After binding, spike undergoes a conformational change that allows the proteolytic cleavage before membrane fusion (Figure 2).
結合後、スパイクは構造変化を受け、膜融合前にタンパク質分解による切断が可能になります (図 2)。
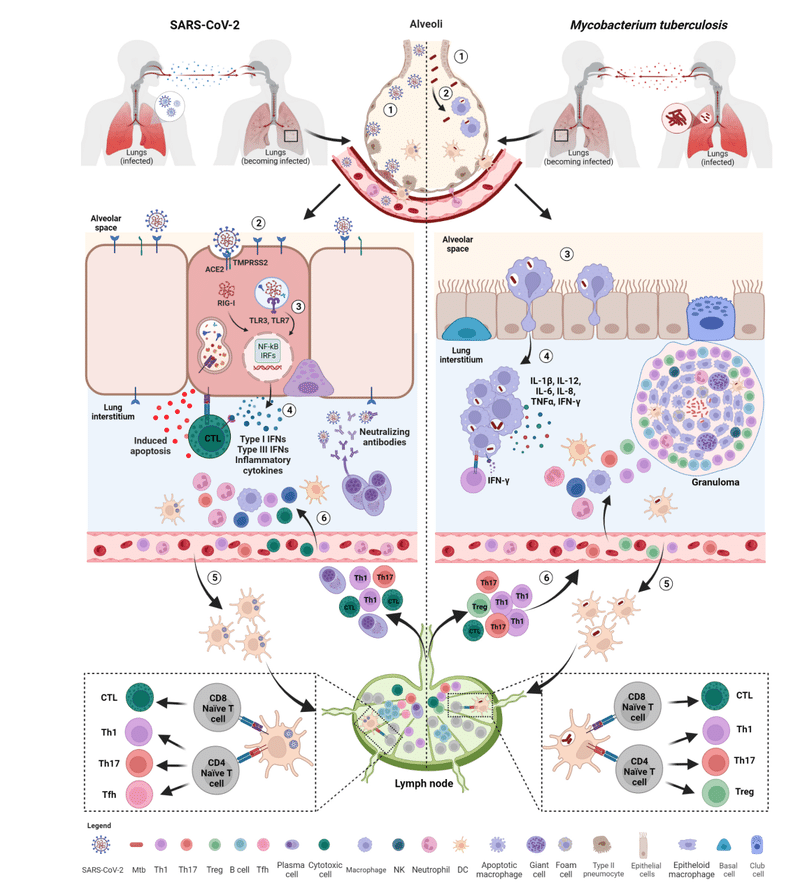
Spike activation can occur either at the cell surface or in endosomes and consists of two different proteolytic events.
スパイク活性化は細胞表面またはエンドソームのいずれかで発生し、2 つの異なるタンパク質分解イベントで構成されます。
The first proteolytic event occurs during spike biosynthesis and it is mediated by the host pro-protein convertase furin that cleaves the polybasic S1/S2 junction generating the two subunits S1 and S2 non-covalently linked and with different roles in the viral entry.
最初のタンパク質分解イベントはスパイク生合成中に発生します。そしてそれは、多塩基性S1/S2接合部を切断する宿主プロタンパク質転換酵素フリンによって媒介され、非共有結合で結合し、ウイルス侵入において異なる役割を持つ2つのサブユニットS1およびS2を生成します。
The amino-terminal S1 subunit includes a receptor-binding domain (RBD) that is involved in the initial recognition of ACE2 receptor, whereas the carboxy-terminal S2 presents highly conserved regions that catalyse the fusion between viral and host cell membranes, crucial to release the viral RNA genome and start the replication in the target cell.
アミノ末端の S1 サブユニットには、ACE2 受容体の初期認識に関与する受容体結合ドメイン (RBD) が含まれています。 一方、カルボキシ末端 S2 には、ウイルスと宿主細胞膜の融合を触媒する高度に保存された領域があり、ウイルス RNA ゲノムを放出して標的細胞内で複製を開始するために重要です。
A further cleavage at the S2’ site is needed to expose the S2’ fragment, a highly hydrophobic fusion peptide that starts the fusion of membranes.
膜の融合を開始する高度に疎水性の融合ペプチドである S2' フラグメントを露出するには、S2' 部位でのさらなる切断が必要です。
Interestingly, TMPRSS2, which is a type 2 transmembrane serine protease (TTSPs) expressed in the human upper and lower respiratory tract, heart, prostate and gastrointestinal tracts, has been shown to prime spikes on cell surface thus allowing the entry via membrane fusion.
興味深いことに、TMPRSS2 はヒトの上気道、下気道、心臓、前立腺、胃腸管で発現する 2 型膜貫通セリンプロテアーゼ (TTSP) であり、細胞表面のスパイクを刺激し、膜融合を介した侵入を可能にすることが示されています。
In the absence or insufficient availability of cell surface proteases, in particular TMPRSS2, SARS-CoV-2 prefers to enter via clathrin-mediated endocytosis.
細胞表面プロテアーゼ、特に TMPRSS2 が存在しないか、利用可能性が不十分な場合、SARS-CoV-2 はクラスリン媒介エンドサイトーシスを介して侵入することを好みます。
In this case, the conformational modifications of the spike occur in the acidic environment of endosomes and its cleavage is mediated by the members of the cathepsin family (e.g. B and L).
この場合、スパイクの構造変化はエンドソームの酸性環境で起こり、その切断はカテプシンファミリーのメンバー(例:B および L)によって媒介されます。
While the virus takes 10 minutes to enter the cells via cell surface membrane fusion, the pH-dependent endocytosis process needs about 40–60 minutes after infection.
ウイルスが細胞表面膜融合を介して細胞に侵入するのに 10 分かかりますが、pH 依存性のエンドサイトーシスのプロセスには感染後約 40 ~ 60 分かかります。
The cleavage of S1/S2 can have an impact on viral fitness and transmission, thus affecting viral infectivity.
S1/S2 の切断はウイルスの適合性と伝播に影響を与える可能性があり、ウイルスの感染力に影響を与えます。
Notably, during the COVID-19 pandemic, several mutations have accumulated in S1 and S2 subunits of the spike causing the emergence of several SARS-CoV-2 VOCs capable of escaping the immune system, while preserving the steps of activation of the spike protein.
注目すべきことに、COVID-19パンデミック中、スパイクタンパク質の活性化のステップを維持しながら、スパイクのS1およびS2サブユニットにいくつかの変異が蓄積し、免疫システムから逃れることができるいくつかのSARS-CoV-2 VOCの出現を引き起こしました。
The different infectivity rate in the epithelial cells of the nose, bronchi, and lung by SARS-CoV-2 VOC is correlated with the different protease expression, subsequent transmissibility, and severity of disease.
SARS-CoV-2 VOC による鼻、気管支、肺の上皮細胞における感染率の違いは、プロテアーゼ発現の違い、その後の感染力、および疾患の重症度と相関しています。
Emerged Omicron subvariants are less dependent on TMPRSS2-mediated spike activation at the plasma membrane, showing a reduced replication of the virus in the lung and intestinal cultures, while a similar replication rate was observed in the nasal epithelia compared to the Delta variant.
出現した Omicron サブバリアントは、細胞膜での TMPRSS2 媒介スパイク活性化への依存性が低く、肺および腸の培養物におけるウイルスの複製が減少していることが示されています。 一方、デルタ変異体と比較して、鼻上皮では同様の複製率が観察されました。
Likely, this modified tropism allowed a major air transmission of the virus, in accordance with the highest rate of spread observed in the latest variants compared with the ancestral one.
おそらく、祖先のものと比較して最新の変異種で観察された最も高い拡散率に従って、この改変された指向性がウイルスの大規模な空気感染を可能にしたと考えられます。
Moreover, the different spike protease tropism resulted in the diminished pathogenesis in the lung.
さらに、異なるスパイクプロテアーゼ指向性により、肺の病因が減少しました。
Besides TMPRSS2, other TTSPs or metalloproteases can mediate SARS-CoV-2 entry.
TMPRSS2 以外にも、他の TTSP またはメタロプロテアーゼが SARS-CoV-2 の侵入を媒介する可能性があります。
For instance, TMPRSS2 and TMPRSS4 promote viral entry into human enterocytes of the proximal digestive tract, and matrix metalloproteases (MMPs), such as ADAM10 and ADAM17, seem to be involved in the cleavage at the S2 site in cells lacking TMPRSS2.
例えば、TMPRSS2 および TMPRSS4 は、近位消化管のヒト腸細胞へのウイルスの侵入を促進し、ADAM10 や ADAM17 などのマトリックスメタロプロテアーゼ (MMP) は、TMPRSS2 を欠く細胞の S2 部位での切断に関与しているようです。
Moreover, coagulation factors, such as factor Xa and thrombin, can directly cleave spike protein at both cleavage sites and thus further contributing to infection at the stage of viral entry.
さらに、第 Xa 因子やトロンビンなどの凝固因子は、両方の切断部位でスパイクタンパク質を直接切断することができるため、ウイルス侵入段階での感染にさらに寄与する可能性があります。
Furthermore, other molecules have been suggested as alternative receptors for the SARS-CoV-2 entry process including integrins, neuropilin 1 (NRP1), phosphatidylserine receptors, the C-type lectins, asialoglycoprotein receptor 1 (ASGR1), Kringle Containing Transmembrane Protein 1 (KREMEN1), and CD147, as reviewed by Jackson and colleagues.
さらに、他の分子がSARS-CoV-2侵入プロセスの代替受容体として示唆されています。Jackson らのレビューによると、インテグリン、ニューロピリン 1 (NRP1)、ホスファチジルセリン受容体、C 型レクチン、アシアロ糖タンパク質受容体 1 (ASGR1)、クリングル含有膜貫通タンパク質 1 (KREMEN1)、および CD147 です。
Notably, SARS-CoV-2 could also infect cells through other mechanisms that allow the virus to escape the immune recognition favoring its spread in the host.
注目すべきことに、SARS-CoV-2は、ウイルスが免疫認識を逃れて宿主内での蔓延を促進する他のメカニズムを通じて細胞に感染する可能性もあります。
In this regard, SARS-CoV-2-infected cells can directly fuse with adjacent cells expressing ACE2 through S1/S2 cleaved SARS-CoV-2 spikes resulting in the formation of multinucleated cells or syncytia.
これに関して、SARS-CoV-2感染細胞は、S1/S2切断されたSARS-CoV-2スパイクを介してACE2を発現する隣接細胞と直接融合することができ、その結果、多核細胞または合胞体が形成される。
The syncytia formation favors a cell-to-cell transmission of the virus without even the need to assemble viral particles or to release the virus in the extracellular environment.
シンシチウムの形成は、ウイルス粒子を組み立てたり細胞外環境にウイルスを放出したりする必要さえなく、ウイルスの細胞間感染を促進します。
SARS-CoV-2-induced multinucleated pneumocytes and syncytia formation is a feature of severe COVID-19 patients, suggesting their involvement in the COVID-19 pathogenesis.
SARS-CoV-2 によって誘発される多核肺細胞と合胞体の形成は、重症の COVID-19 患者の特徴です。 COVID-19の病因に関与していることを示唆しています。
Moreover, these structures might cause direct cytopathic effects to lymphocytes.
さらに、これらの構造はリンパ球に直接的な細胞変性効果を引き起こす可能性があります。
In this regard, Zhang and colleagues reported that lymphocytes could be internalized by syncytia by forming cell-in-cell structures and leading to cell death.
これに関して、Zhangらは、リンパ球が細胞間構造を形成し、細胞死を引き起こすことにより、合胞体によって取り込まれる可能性があると報告した。
Another possible mechanism for viral entry is mediated by extracellular vesicles (EVs) containing particles or viral components well documented in SARS-CoV-2-infected cells.
ウイルス侵入のもう 1 つの考えられるメカニズムは、SARS-CoV-2 感染細胞で十分に実証されている粒子またはウイルス成分を含む細胞外小胞 (EV) によって媒介されるものです。
Regardless of the mechanism and molecules involved in SARS-CoV-2 entry, the virus replicates triggering the host immune response.
SARS-CoV-2 の侵入に関与するメカニズムや分子に関係なく、ウイルスは複製して宿主の免疫応答を引き起こします。
M. tuberculosis and cell tropism and entry mechanisms
結核菌と細胞指向性および侵入メカニズム
As for SARS-CoV-2, the first interactions between bacteria and host occur in the lungs after the inhalation of the aerosolized Mtb.
SARS-CoV-2 と同様に、細菌と宿主の間の最初の相互作用は、エアロゾル化された Mtb の吸入後に肺で起こります。
The size of Mtb droplets (2–5 µm particles) is important to ensure the passage through the upper respiratory tract into the alveolar space, where bacilli primarily encounter pneumocytes, epithelial cells (AEC), and alveolar macrophages (AMs) with anti-bacterial capacities.
Mtb 液滴 (2 ~ 5 μm の粒子) のサイズは、上気道を通って肺胞腔に確実に通過するために重要です。 ここで、桿菌は主に抗菌能力を持つ肺細胞、上皮細胞 (AEC)、および肺胞マクロファージ (AM) に遭遇します。
On the other hand, larger droplets can be stuck in the upper airways or oropharynx probably explaining the onset of the extrapulmonary forms of TB localized in the oropharynx but lacking evidence of concurrent pulmonary disease.
一方で、より大きな飛沫が上気道または中咽頭に付着する可能性があり、おそらく中咽頭に局在する肺外型の結核の発症を説明しますが、肺疾患の併発の証拠はありません。
Once entered into the airways, Mtb is phagocytosed by AMs, which are permissive for infection establishment.
Mtb は気道に入ると、感染の確立を許容する AM によって貪食されます。
In the upper airway, Mtb invades the specialized epithelial cells called microfold cell (M cell) through the binding to the scavenger receptor B1 in both mouse and human tissue.
上気道では、Mtb はマウスとヒトの両方の組織のスカベンジャー受容体 B1 への結合を介して、マイクロフォールド細胞 (M 細胞) と呼ばれる特殊な上皮細胞に侵入します。
Similar to SARS-CoV-2, Mtb can disseminate to other organs including the lymphatics and lymph nodes that are the main sites of extrapulmonary TB.
SARS-CoV-2 と同様に、Mtb は肺外結核の主な部位であるリンパ管やリンパ節などの他の臓器に広がる可能性があります。
Lymphatic endothelial cells, the adipose tissue and the bone marrow have been identified as extrapulmonary niches where Mtb may persist for long time.
リンパ内皮細胞、脂肪組織、骨髄は、Mtb が長期間存続する可能性がある肺外ニッチとして特定されています。
The receptors involved in the Mtb entry into cells have not been fully demonstrated.
Mtb の細胞への侵入に関与する受容体は完全には証明されていません。
Phagocytosis of Mtb by macrophages seems not occur via a single receptor-mediated pathway, but rather it seems to be mediated by multiple receptors including dectin-1, the complement receptor 3, mannose receptor, the dendritic cell-specific intercellular adhesion molecule (ICAM)-3-grabbing nonintegrin (DC-SIGN), Fc receptors, scavenger receptors and CD14.
マクロファージによるMtbの貪食は、単一の受容体媒介経路を介して起こるものではないようです。むしろ、複数の受容体によって媒介されているようです。これには、デクチン-1、補体受容体 3、マンノース受容体、樹状細胞特異的細胞間接着分子 (ICAM)-3 捕捉非インテグリン (DC-SIGN)、Fc 受容体、スカベンジャー受容体、CD14 が含まれます。
Other receptors, such as Toll-like receptors (TLRs) are involved in the recognition of mycobacteria.
トール様受容体 (TLR) などの他の受容体は、マイコバクテリアの認識に関与しています。
To enable their entrance into AMs, mycobacteria exploit a group of pathogen-associated molecular patterns (PAMPs) expressed on its surface, including mycobacterial lipoproteins such as the 19 kDa surface antigen LpqH, which acts as an adhesin playing a crucial role in both host-pathogen interactions and pleiotropic immune regulation through the engagement of the TLR1/TLR2.
マイコバクテリアは、AM への侵入を可能にするために、19 kDa 表面抗原 LpqH などのマイコバクテリア リポタンパク質を含む、その表面に発現する一群の病原体関連分子パターン (PAMP) を利用します。 これは、宿主と病原体の相互作用と、TLR1/TLR2の関与を介した多面的免疫制御の両方において重要な役割を果たす付着因子として機能します。
The downstream signaling and the phagosomal fate depend on the type of receptor engaged during the phagocytosis.
下流のシグナル伝達とファゴソームの運命は、食作用中に関与する受容体の種類に依存します。
Macrophages containing Mtb then migrate from the air space to the lung interstitium in an IL1-R signaling- and ESX-1 secretion system-dependent manner.
その後、Mtb を含むマクロファージは、IL1-R シグナル伝達系と ESX-1 分泌系に依存して気腔から肺間質へ移動します。
This is the first step preceding the formation of the granuloma, the pathologic hallmark of TB (Figure 2).
これは、結核の病理学的特徴である肉芽腫の形成に先立つ最初のステップです (図 2)。
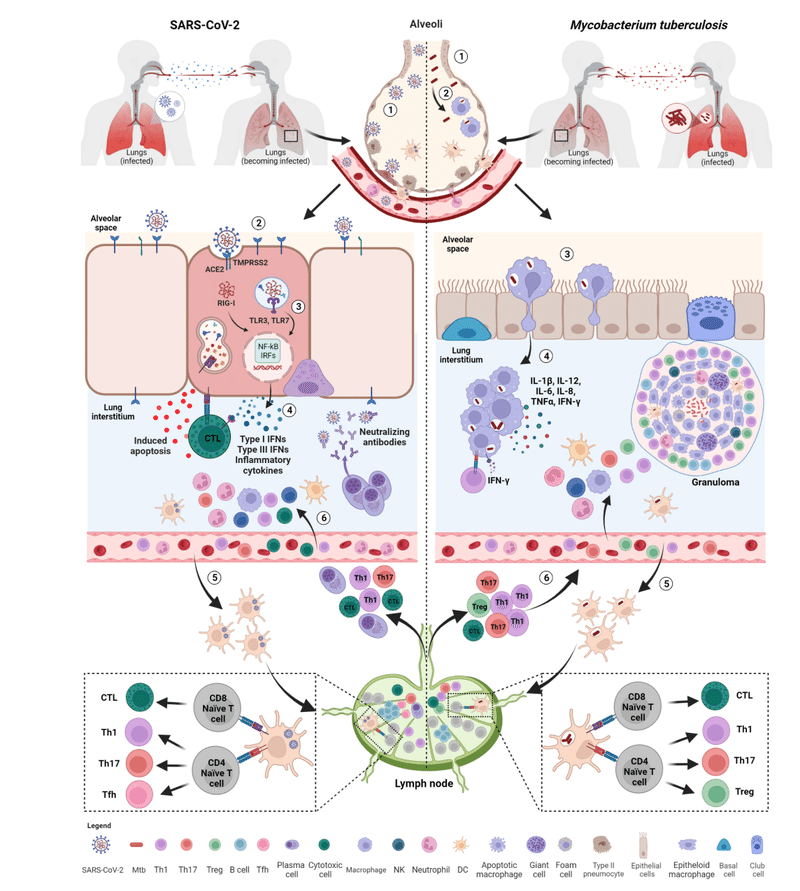
Innate immune response
自然免疫応答
Whereas Mtb causes a slow-progressing infection that might result in the development of TB disease even after many years, the SARS-CoV-2 infection evolves rapidly causing COVID-19 (Figure 1).
Mtb は感染の進行が遅く、何年も経っても結核疾患を発症する可能性があるのに対し、SARS-CoV-2 感染は急速に進化し、COVID-19 を引き起こします (図 1)。
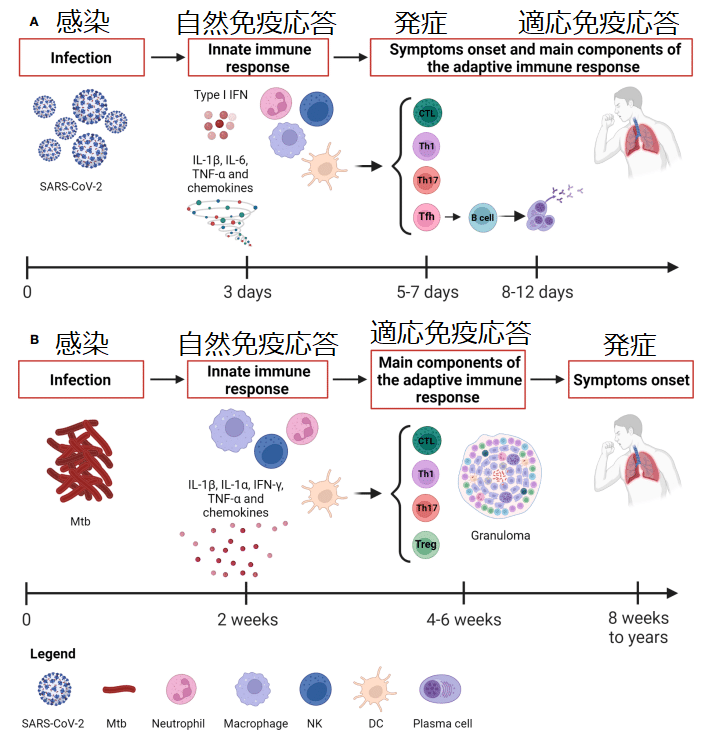
Within the immunological response to Mtb and SARS-CoV-2, both the innate and adaptive responses play an important role.
Mtb および SARS-CoV-2 に対する免疫学的応答では、先天的応答と適応的応答の両方が重要な役割を果たします。
The innate immune response is a nonspecific response that serves as initial defense against pathogens.
自然免疫応答は、病原体に対する最初の防御として機能する非特異的応答です。
It consists of humoral components (cytokines, chemokines, interferons, complement and coagulation-fibrinolysis systems, and naturally occurring antibodies) and cellular components (natural killer cells, macrophages, dendritic cells and other innate lymphocytes).
これは、体液性成分(サイトカイン、ケモカイン、インターフェロン、補体および凝固線溶系、天然抗体)と細胞成分(ナチュラルキラー細胞、マクロファージ、樹状細胞およびその他の自然リンパ球)から構成されます。
Innate immunity aids in controlling the infection, in the identification and eradication of infected cells as well as in the development of the adaptive immunity.
自然免疫は、感染の制御、感染細胞の特定と根絶、および適応免疫の発達に役立ちます。
Innate immune response to SARS-CoV-2
SARS-CoV-2 に対する自然免疫応答
The heterogeneous course of SARS-CoV-2 infection depends on the immune response at the early stages of infection.
SARS-CoV-2 感染の不均一な経過は、感染の初期段階での免疫反応に依存します。
Considering the rapid course of COVID-19, the capability of patients with asymptomatic or mild disease to control the infection is likely due to the innate immune response since the adaptive response occurs days later, with the T cell immunity preceding the B cell response occurring after 2 weeks (Figure 1).
COVID-19の急速な経過を考慮すると、適応反応は数日後に起こるため、無症候性または軽症の患者が感染を制御できるのは、おそらく自然免疫反応によるものと考えられます。T 細胞免疫が 2 週間後のB 細胞応答に先行して起こります (図 1)。
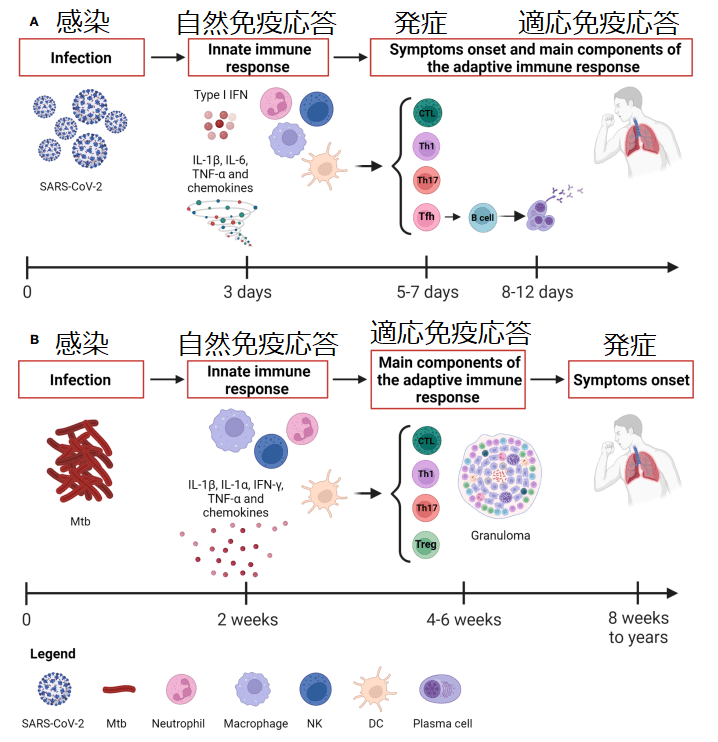
Early on, an effective control of SARS-CoV-2 spread depends on the induction of a robust antiviral response and on the ability of alveolar macrophages to eliminate the virus and the infected cells through phagocytosis.
初期段階で SARS-CoV-2 の蔓延を効果的に制御できるかどうかは、強力な抗ウイルス反応の誘導と、貪食を通じてウイルスと感染細胞を除去する肺胞マクロファージの能力にかかっています。
Immune cells resident within the lung recognize SARS-CoV-2 through several pathogen-recognition receptors (PRRs), such as TLRs (TL3 and TLR7), retinoic acid-inducible gene I (RIG-I)-like receptors (RLRs), nucleotide-binding oligomerization domain (NOD)-like receptors (NLRs) and inflammasomes.
肺内に存在する免疫細胞は、いくつかの病原体認識受容体 (PRR) を通じて SARS-CoV-2 を認識します。 TLR (TL3 および TLR7)、レチノイン酸誘導性遺伝子 I (RIG-I) 様受容体 (RLR)、ヌクレオチド結合オリゴマー化ドメイン (NOD) 様受容体 (NLR)、インフラマソームなどです。
As a result, downstream signaling pathways involving nuclear factor kappa-light-chain-enhancer of activated B cells (NF-kB) and interferon regulatory factors (IRFs) are activated inducing the production of multiple pro-inflammatory cytokines such as IL-1β, IL-6, and TNF-α, several chemokines (CCL20, CXCL1, CXCL2, CXCL3, CXCL5, CXCL6, CXCL8 and CXCL16) and antiviral IFNs resulting in the initial inflammation.
その結果、活性化B細胞の核因子カッパ軽鎖エンハンサー(NF-kB)とインターフェロン調節因子(IRF)が関与する下流のシグナル伝達経路が活性化され、複数の炎症誘発性サイトカインの産生が誘導されます。 IL-1β、IL-6、TNF-α、いくつかのケモカイン(CCL20、CXCL1、CXCL2、CXCL3、CXCL5、CXCL6、CXCL8、CXCL16)などです。 抗ウイルス性 IFN は初期の炎症を引き起こします。
The local innate immune response attracts and activates into the site of infection further innate immune cells such as neutrophils, monocytes, dendritic cells (DCs), natural killer (NK), and innate lymphoid cells aimed to promote viral clearance (Figure 2).
局所的な自然免疫応答は、ウイルス排除の促進を目的とした好中球、単球、樹状細胞 (DC)、ナチュラルキラー (NK)、自然リンパ球などの自然免疫細胞をさらに感染部位に引き付けて活性化します (図 2)。
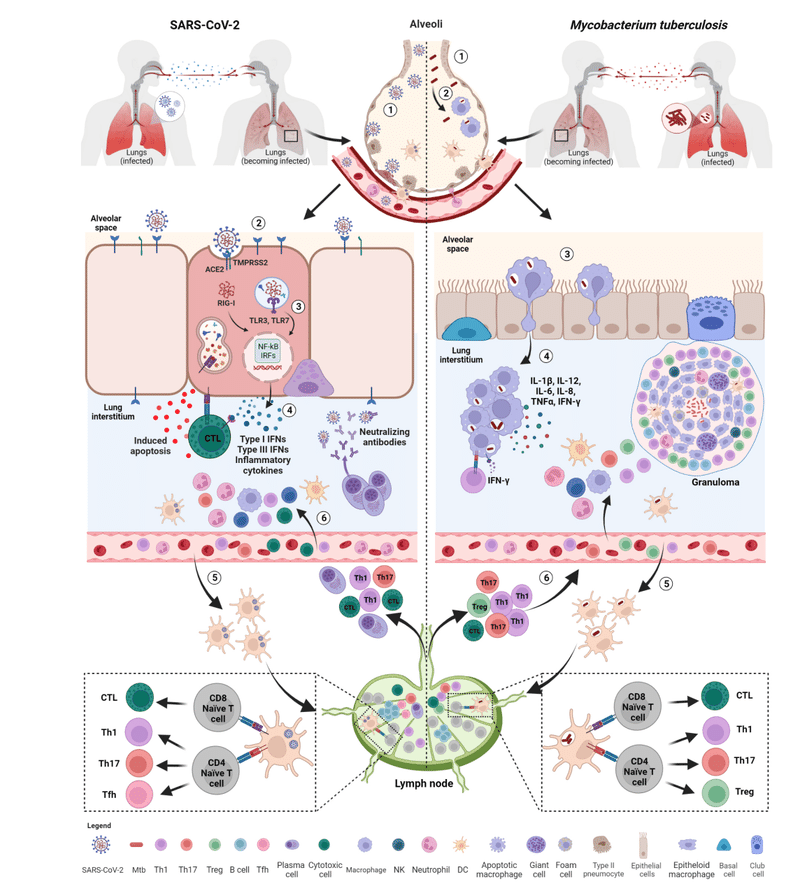
Consequently, the combined action of innate immune cells, cytokines, and chemokines may have an impact on the outcome of SARS-CoV-2 infection.
したがって、自然免疫細胞、サイトカイン、ケモカインの複合作用が SARS-CoV-2 感染の結果に影響を与える可能性があります。
Although SARS-CoV-2 induces a pro-inflammatory state, there are reports of reduced IFN release; in fact, SARS-CoV-2 is more effective at suppressing IFN responses compared to other respiratory viruses.
SARS-CoV-2 は炎症促進状態を誘発しますが、IFN 放出が減少するという報告があります; 実際、SARS-CoV-2 は他の呼吸器ウイルスと比較して、IFN 応答の抑制においてより効果的です。
Type I IFN, which includes IFN-α and IFN-β, represents the primary defensive response against viral infections by the induction of antiviral effector molecules encoded by IFN-stimulated genes (ISGs) and immunomodulatory responses.
IFN-α および IFN-β を含む I 型 IFN は、IFN 刺激遺伝子 (ISG) によってコードされる抗ウイルスエフェクター分子の誘導および免疫調節応答によるウイルス感染に対する主要な防御反応を表します。
In SARS-CoV-2-infected individuals, the presence of a quick type I IFN production soon after infection contributes to protection against critical illness as observed in studies conducted in individuals exposed to COVID-19 cases.
COVID-19に曝露された人を対象に実施された研究で観察されたように、SARS-CoV-2感染者では、感染直後の迅速なI型IFN産生の存在が重篤な疾患に対する防御に寄与しています。
On the contrary, if a strong and rapid antiviral response is lacking, the ongoing infection can lead to an exuberant release of cytokines and chemokines that is amplified by the further infiltration of circulating immune cells, finally provoking the so-called “cytokine storm”, which can be caused by infectious and non-infectious agents, and which in COVID-19 is responsible for the immunopathology associated with its severe presentation.
逆に、強力かつ迅速な抗ウイルス反応が欠如している場合、感染が進行するとサイトカインやケモカインが過剰に放出され、循環免疫細胞のさらなる浸潤によって増幅され、最終的にいわゆる「サイトカインストーム」を引き起こす可能性があります。これは感染性病原体と非感染性病原体によって引き起こされる可能性があり、COVID-19では、その重篤な症状に関連する免疫病理の原因となります。
Based on the evidence, individuals with highly compromised IFN-I response, which means no IFN-β and low IFN-α production and activity due to neutralizing auto-antibodies or inherited errors of type I IFN immunity, do not control the primary SARS-CoV-2 infection and they are more at risk of fatal COVID-19.
証拠に基づいて、IFN-I 反応が高度に低下している個人(中和自己抗体または I 型 IFN 免疫の遺伝的エラーにより、IFN-β が存在せず、IFN-α の産生と活性が低いことを意味)は、SARS-CoV-2 の初感染を制御できず、致死的な COVID-19 のリスクが高くなります。
Moreover, a low number and an impaired functionality of plasmacytoid dendritic cells (pDCs), which are the main IFN producers, have been found in bronchoalveolar lavage fluid (BALF) from severe or critical patients compared to the moderate ones.
さらに、主要な IFN 産生細胞である形質細胞様樹状細胞 (pDC) の数が少なく、機能が損なわれていることが、中等度の患者と比較して、重症または重篤な患者の気管支肺胞洗浄液 (BALF) で見つかりました。
Also, a lower frequency of circulating pDCs was found in samples from SARS-CoV-2-infected individuals than in controls.
また、SARS-CoV-2感染者のサンプルでは、対照よりも循環pDCの頻度が低いことが判明した。
In vitro studies have shown the presence of a huge amount of NF-kB-dependent proinflammatory mediators in BALF (CCL2, CCL3, CCL4, and CXCL10) and in circulation (IP-10, IL-6, and IL-8, IL-1, IFN-γ, IL-17, TNF-α, MCP-1, G-CSF, GM-CSF, IL-1RA, CCL2, CCL3, CCL5, CCL8, CXCL2, CXCL8, CXCL9, and CXCL16).
インビトロ研究では、BALFおよび循環中に大量の NF-kB 依存性炎症誘発性メディエーターが存在することが示されています。BALFにはCCL2・CCL3・CCL4・CXCL10、循環中にはIP-10・ IL-6・IL-8・IL-1・IFN-γ・IL-17・TNF-α・ MCP-1・G-CSF・GM-CSF・IL-1RA・CCL2・CCL3・CCL5・CCL8・CXCL2・CXCL8・CXCL9・CXCL16。
Patients with COVID-19 generally show migration of neutrophils and monocytes into the nasopharyngeal mucosa in response to chemokines released by infected epithelial cells (e.g. CXCL1, CXCL3, CXCL6, CXCL15, CXCL16, and CXCL17).
COVID-19患者は一般に、感染した上皮細胞から放出されるケモカインに反応して、鼻咽頭粘膜への好中球と単球の遊走を示します(例: CXCL1、CXCL3、CXCL6、CXCL15、CXCL16、CXCL17)。
Once reached the lung, neutrophils as phagocytes may exert a protective role in the clearance of the infection by secreting leukotrienes, reactive oxygen species (ROS), and forming neutrophil extracellular traps (NET), which are aggregates of extracellular DNA, histones, microbicidal proteins and proteases aimed to entrap and kill pathogens.
肺に到達すると、食細胞としての好中球は、ロイコトリエン、活性酸素種(ROS)を分泌し、好中球細胞外トラップ(NET)を形成することにより、感染症の除去において保護的な役割を果たす可能性があります。 NETは、病原体を捕らえて殺すことを目的とした細胞外 DNA、ヒストン、殺菌性タンパク質、プロテアーゼの集合体です。
However, neutrophils are known to be implicated in COVID-19 pathology as hyperinflammation drivers through increased cytokine production and cell degranulation.
しかし、好中球は、サイトカイン産生の増加と細胞脱顆粒を介して過剰炎症を促進する要因として、COVID-19の病理に関与していることが知られている。
Indeed, their extensive and prolonged activation causes an hyperinflammatory environment and cellular infiltrations that may result in the tissue damage observed in the ARDS and increased mortality.
実際、それらの大規模かつ長期にわたる活性化は過剰炎症環境と細胞浸潤を引き起こし、ARDS で観察される組織損傷と死亡率の増加を引き起こす可能性があります。
Indeed, a high neutrophil-to-lymphocyte ratio (NLR), that is a marker of inflammation and infection, and NET DNA complexes have been found in severe COVID-19 compared with mild/moderate cases or healthy controls.
実際、炎症や感染のマーカーである好中球対リンパ球比(NLR)とNET DNA複合体が、軽度/中等度の症例や健康な対照と比較して、重症COVID-19では高いことが認められている。
In addition to NET generation, another source of hyperinflammation associated with COVID-19 is the activation of the NLRP3-inflammasome due to the interaction of the nucleoprotein (N) with NLRP3.
NET の生成に加えて、COVID-19 に関連するもう 1 つの過剰炎症の原因は、核タンパク質 (N) と NLRP3 の相互作用による NLRP3 インフラマソームの活性化です。
In this regard, a study conducted in an ACE2 humanized mouse model of COVID-19 showed that, in response to infection, macrophages activate inflammasomes causing the release of IL-1β and IL-18 and undergo pyroptosis, thus favoring the pathogenesis of acute lung injury.
これに関して、COVID-19のACE2ヒト化マウスモデルで行われた研究では、感染に反応してマクロファージがインフラマソームを活性化し、IL-1βとIL-18の放出を引き起こし、パイロトーシスを起こすことが示された。 したがって、急性肺損傷の発症を促進します。
During SARS-CoV-2 infection, monocytes/macrophages are involved either as virus target or as producer of inflammatory cytokines and undergo phenotypical changes.
SARS-CoV-2 感染中、単球/マクロファージはウイルスの標的として、または炎症性サイトカインの生産者として関与し、表現型の変化を受けます。
Alterations in the phenotype of monocytes consisting of reduced antigenic presentation and dysregulated immune response have been observed.
抗原提示の減少および免疫応答の調節不全からなる単球の表現型の変化が観察されています。
In the peripheral blood of COVID-19 patients there are cell subsets of mixed M1/M2 macrophages secreting IL-6, TNF-α and IL-10 and characterized by higher expression of CD80 and CD86.
COVID-19 患者の末梢血には、IL-6、TNF-α、IL-10 を分泌する混合 M1/M2 マクロファージの細胞サブセットが存在し、CD80 と CD86 の発現が高いことが特徴です。
NK cells are innate lymphocytes that are recruited along with macrophages and neutrophils in the lungs as confirmed by the analysis on BALF samples of COVID-19 patients.
NK細胞は、COVID-19 患者のBALFサンプルの分析で確認されたように、肺内でマクロファージや好中球とともに補充される自然リンパ球である。
NK cells usually exert an antiviral activity through the production of the effector cytokines IFN-γ and TNF-α and limit tissue fibrosis.
NK 細胞は通常、エフェクター サイトカイン IFN-γ および TNF-α の産生を通じて抗ウイルス活性を発揮し、組織の線維化を制限します。
Regarding the protective role of NK cells against infection, Witkowski and colleagues reported that SARS-CoV-2-infected individuals with a higher NK cell number at hospitalization showed a more rapid clearance of viral load.
感染に対するNK細胞の保護的役割に関して、Witkowskiらは、入院時のNK細胞数が多いSARS-CoV-2感染者は、より迅速なウイルス量の除去を示したと報告した。
Although during early stages of infection NK cells may contribute to control viral replication and dissemination, their migration in affected tissue may favor the enhancement of inflammation.
感染の初期段階では、NK 細胞はウイルスの複製と播種の制御に寄与する可能性がありますが、罹患組織内での NK 細胞の移動は炎症の増強に有利に働く可能性があります。
In this context, a reduced peripheral cell count and functional impairment of NK cells with an enhanced expression of the cytolytic proteins perforin and granzyme B have been found in patients with severe COVID-19.
これに関連して、重度 COVID-19患者では、末梢細胞数の減少と、細胞溶解タンパク質であるパーフォリンおよびグランザイムBの発現亢進によるNK細胞の機能障害が見出されている。
CD1d-restricted NKT cells are other types of innate lymphocytes that are involved in antiviral immunity.
CD1d 拘束性 NKT 細胞は、抗ウイルス免疫に関与する他の種類の自然リンパ球です。
To counteract their function, the envelope (E) protein of SARS-CoV-2 reduces the expression of the antigen-presenting molecule CD1d thus inhibiting the activation of innate NKT cells and enhancing SARS-CoV-2 virulence.
それらの機能に対抗するために、SARS-CoV-2 のエンベロープ (E) タンパク質は抗原提示分子 CD1d の発現を減少させ、これにより先天性 NKT 細胞の活性化を阻害し、SARS-CoV-2 の毒性を増強します。
The activation of the innate immune system is essential to mount an effective adaptive immune response.
自然免疫系の活性化は、効果的な適応免疫応答を開始するために不可欠です。
In this regard, DCs, as professional antigen presenting cells (APCs), represent a point of junction between innate and adaptive immune response as they migrate to lymph nodes to activate naïve T lymphocytes.
この点において、プロフェッショナル抗原提示細胞(APC)としての DC は、リンパ節に移動してナイーブ T リンパ球を活性化する際に、自然免疫応答と適応免疫応答の間の接合点を表します。
Innate immune response to M. tuberculosis
結核菌に対する自然免疫応答
The innate immune response to Mtb infection is multifaceted with several different cell types and functions involved.
Mtb 感染に対する自然免疫応答は、いくつかの異なる細胞型と機能が関与していて多面的です。
Upon pattern recognition, a variety of cellular functions, including phagocytosis, autophagy, and apoptosis will be launched by the host to clear or control Mtb.
パターンが認識されると、食作用、オートファジー、アポトーシスなどのさまざまな細胞機能が宿主によって起動され、Mtb を除去または制御します。
In particular, macrophages with antimicrobial mechanisms such as nitric oxide synthesis and antimicrobial peptides such as cathelicidin represent the first defense line against Mtb infection.
特に、一酸化窒素合成などの抗菌機構を持つマクロファージやカテリシジンなどの抗菌ペプチドは、Mtb感染に対する第一の防御線となります。
The investigation of the early events and host responses against Mtb in humans is very challenging and difficult as the progression of infection is generally slow and individuals often do not know the exact time of exposure or infection.
ヒトにおけるMtbに対する初期事象と宿主反応の調査は、感染の進行が一般に遅く、個人が曝露や感染の正確な時期を知らないことが多いため、非常に難解かつ困難である。
Therefore, a validated model that recapitulates TB in human lungs is critical to support TB research.
したがって、ヒトの肺における結核を再現する検証済みのモデルは、結核研究をサポートするために重要です。
In this regard, a number of in vitro systems, spheroids, human airway organoids, and experimental animal models of TB such as zebrafish, mouse, guinea pig, rabbit and rat have provided new insights into the local events that occur during few days and weeks post Mtb infection.
これに関して、多くの in vitro システム、スフェロイド、ヒト気道オルガノイド、およびゼブラフィッシュ、マウス、モルモット、ウサギ、ラットなどの結核実験動物モデルが研究されています。 Mtb 感染後の数日から数週間の間に起こる局地的の出来事についての新たな洞察を提供しました。
In particular, Mtb infection in nonhuman primates closely recapitulates human TB and these models can be used to study the full spectrum of infection outcome and pathology of TB.
特に、非ヒト霊長類におけるMtb感染はヒト結核をよく再現しており、これらのモデルは感染転帰と結核の病理の全範囲を研究するために使用することができます。
Early in infection, the infected cells are activated and start to release some early mediators of inflammation such as TNF-α, IL-1α, IL-1β, IFN-γ and chemo-attractant molecules (e.g. CXCL5, CXCL8), some of which also characterize the early stages of SARS-CoV-2 infection (Figure 2).
感染の初期に、感染細胞は活性化され、TNF-α、IL-1α、IL-1β、IFN-γ、化学誘引分子(CXCL5、CXCL8など)などの炎症の初期メディエーターを放出し始めます。 その一部は、SARS-CoV-2 感染の初期段階の特徴でもあります (図 2)。
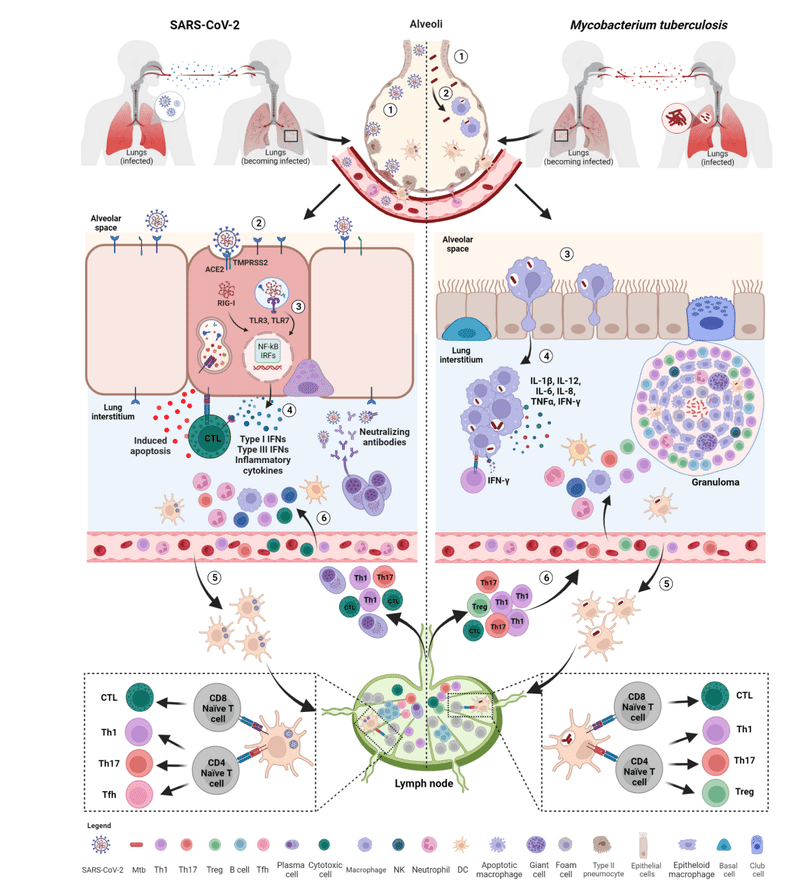
These soluble factors mediate the recruitment to the site of infection of different blood cell types including neutrophils, monocytes, macrophages and DCs, which are necessary for starting early granuloma formation.
これらの可溶性因子は、初期の肉芽腫形成を開始するために必要な、好中球、単球、マクロファージ、DC などのさまざまな種類の血液細胞の感染部位への動員を仲介します。
These innate granulomas include cells that are not yet fully activated, thus favoring the dissemination of mycobacteria from infected macrophages to uninfected cells.
これらの先天性肉芽腫にはまだ完全に活性化されていない細胞が含まれているため、感染したマクロファージから非感染細胞へのマイコバクテリアの伝播を促進します。
Notably, the EVs released from infected cells containing mycobacterial components, including lipoarabinomannan, the Ag85 complex and lipoproteins, have been shown to contribute to the migration of immune cells to the lungs.
特に、リポアラビノマンナン、Ag85複合体、リポタンパク質などのマイコバクテリア成分を含む感染細胞から放出されるEVは、免疫細胞の肺への遊走に寄与することが示されています。
Moreover, EVs can modulate immune response by promoting the release of proinflammatory cytokines and by increasing autophagy and superoxide production.
さらに、EV は炎症誘発性サイトカインの放出を促進し、オートファジーとスーパーオキシドの生成を増加させることによって免疫応答を調節できます。
During the first 10 days post-infection, Mtb almost exclusively resides and replicates inside AMs, suggesting that these cells provide an early niche for Mtb growth.
感染後の最初の 10 日間、Mtb はほぼ独占的に AM 内に存在し複製されます。このことは、これらの細胞が Mtb 増殖の初期ニッチを提供していることを示唆しています。
In a murine model of TB, Mtb was reported to be equally distributed between AMs, DCs and neutrophils 14 days post-aerosol challenge.
結核のマウスモデルでは、エアロゾル攻撃から 14 日後に、Mtb が AM、DC、好中球に均等に分布していることが報告されました。
As already mentioned for SARS-CoV-2 and also known for other infections including Mtb, DCs play a crucial role by transporting bacteria from the site of infection to the draining lymph nodes in order to prime naïve T cells and start an adaptive immune response.
SARS-CoV-2 についてすでに述べたように、また Mtb を含む他の感染症でも知られているように、DC はナイーブ T 細胞を刺激して適応免疫応答を開始するために、細菌を感染部位から所属リンパ節に輸送することで重要な役割を果たします。
An involvement of CCR2+ inflammatory monocytes in the Mtb delivery to pulmonary lymph nodes has also been reported.
肺リンパ節への Mtb 送達における CCR2+ 炎症性単球の関与も報告されています。
Notably, a higher monocyte/lymphocyte ratio is observed in Mtb-infected patients.
注目すべきことに、Mtb感染患者ではより高い単球/リンパ球比が観察されます。
Neutrophils are other professional phagocytes that have been shown to be involved in the early innate immune response against Mtb through a direct antimicrobial activity and chemokines/cytokines production.
好中球は、直接的な抗菌活性およびケモカイン/サイトカインの産生を通じて、Mtb に対する初期の自然免疫応答に関与することが示されている他の専門的な食細胞です。
They readily phagocytose Mtb and can destroy it via ROS, proteases and antimicrobial peptides (AMPs).
それらは容易に Mtb を貪食し、ROS、プロテアーゼ、抗菌ペプチド (AMP) を介してそれを破壊します。
They can also undergo apoptosis and microbe-containing apoptotic neutrophils can be phagocytosed by macrophages and DCs and then transported to the lymph nodes.
これらはアポトーシスを起こすこともあり、微生物を含むアポトーシス好中球はマクロファージや DC によって貪食され、リンパ節に輸送されます。
In addition, mucosal-associated invariant T cells (MAITs) are a group of T cells restricted to a nonclassical molecule MR-1 and not to the classical major histocompatibility complex (MHC) molecules.
さらに、粘膜関連不変 T 細胞 (MAIT) は、古典的な主要組織適合性複合体 (MHC) 分子ではなく、非古典的分子 MR-1 に限定された T 細胞のグループです。
MAITs are also involved in the early responses to Mtb by producing IFN-γ and TNF-α, and showing cytotoxic activity upon recognition of microbe-derived riboflavin metabolites.
MAIT は、IFN-γ および TNF-α を産生し、微生物由来のリボフラビン代謝産物を認識すると細胞傷害活性を示すことにより、Mtb に対する初期応答にも関与しています。
Moreover, there is evidence for a role of NK cells in controlling Mtb infection, by killing the pathogen through antibody-dependent cellular cytotoxicity, directly targeting the Mtb by binding to cell wall components such as mycolic acid, arabinogalactan, peptidoglycan through receptors including TLR-2, NKp44, NKp46, and NK group 2D (NKG2D), promoting the maturation of phagolysosome and phagocytosis by producing cytokines such as IFN-γ and TNF-α and by killing Mtb-infected macrophages through the release of granules (perforin, granulysin, and granzyme).
さらに、Mtb 感染の制御における NK 細胞の役割についての証拠があります。つまり、抗体依存性の細胞毒性を介して病原体を殺す、TLR-2、NKp44、NKp46、NKグループ2D(NKG2D)などの受容体を介してミコール酸、アラビノガラクタン、ペプチドグリカンなどの細胞壁成分に結合することでMtbを直接標的にする、IFN-γやTNF-αなどのサイトカインを産生し、顆粒(パーフォリン、グラニュリシン、グランザイム)の放出を通じてMtb感染マクロファージを死滅させることにより、ファゴリソソームの成熟と食作用を促進することです。
However, it is not well known whether the role of NK cells is as important as that of macrophages or cytokines such as IFN-γ or TNF-α.
しかし、NK細胞の役割がマクロファージやIFN-γやTNF-αなどのサイトカインの役割と同じくらい重要であるかどうかはよくわかっていません。
Nonetheless, Mtb has evolved several strategies to evade the host’s immune system through its unique cell wall structure, intracellular survival, dormancy and the ability to modulate immune response.
それにもかかわらず、Mtb は、その独特の細胞壁構造、細胞内生存、休眠、免疫応答を調節する能力を通じて宿主の免疫系を回避するためのいくつかの戦略を進化させてきました。
Mtb has adapted to survive and replicate in macrophages by inhibiting phagosome maturation and promoting necrosis over apoptosis.
Mtb は、ファゴソームの成熟を阻害し、アポトーシスよりも壊死を促進することにより、マクロファージ内で生存および複製するように適応しています。
Several types of programmed necrosis in response to Mtb infection, such as inflammasome-mediated pyroptosis and NET-associated NETosis have been identified.
インフラマソーム媒介ピロトーシスや NET 関連 NETosis など、Mtb 感染に応答したプログラムされた壊死のいくつかのタイプが特定されています。
However, NETosis may facilitate the interactions between neutrophils and other immune cells rather than killing Mtb directly.
しかし、NETosis は、Mtb を直接殺すのではなく、好中球と他の免疫細胞の間の相互作用を促進する可能性があります。
Moreover, the formation of NETs can be induced via type I IFN signaling to favor MTB replication.
さらに、MTB 複製を促進するために、I 型 IFN シグナル伝達を介して NET の形成を誘導することもできます。
Mtb inhibits also innate immune response by induction of TLR2 antagonist glycolipids.
Mtb は、TLR2 アンタゴニスト糖脂質の誘導によって自然免疫応答も阻害します。
It also modulates the immune response through the release of molecules that suppress the production of proinflammatory cytokines or even by inducing the production of anti-inflammatory cytokines.
また、炎症誘発性サイトカインの産生を抑制する分子の放出や、さらには抗炎症性サイトカインの産生を誘導することによって免疫応答を調節します。
As for SARS-CoV-2, the control of Mtb infection requires a timely innate response as well as an effective adaptive response.
SARS-CoV-2 と同様に、Mtb 感染の制御には、効果的な適応反応だけでなく、タイムリーな先天的反応も必要です。
Adaptive immune response
適応免疫応答
The adaptive immune response comprises antibody and cell-mediated responses and takes approximately 2 to 3 weeks before we can measure it.
適応免疫応答は抗体と細胞媒介性の応答で構成されており、測定できるようになるまでに約 2 ~ 3 週間かかります。
It is involved in the specific recognition of pathogens and in the establishment of the immunological memory.
それは病原体の特異的認識と免疫学的記憶の確立に関与しています。
Notwithstanding the importance of innate responses, a coordinated cellular immunity is crucial for disease control in both SARS-CoV-2 and Mtb infection.
自然反応の重要性にもかかわらず、SARS-CoV-2 と Mtb 感染の両方における疾患制御には、調整された細胞免疫が重要です。
T cell response to SARS-CoV-2
SARS-CoV-2 に対する T 細胞の応答
In the majority of cases, SARS-CoV-2 infection induces adaptive antigen-specific responses, viral clearance and immunological memory finally resulting in an asymptomatic or mild disease.
ほとんどの場合、SARS-CoV-2 感染は、適応的な抗原特異的反応、ウイルス除去、免疫学的記憶を誘導し、最終的には無症候性または軽度の疾患をもたらします。
However, a failure of the first line defense mechanisms, particularly of innate IFN, may act as triggering factor for viral proliferation and immune dysregulation.
しかし、第一線の防御機構、特に先天性 IFN の不全は、ウイルスの増殖や免疫調節異常の引き金として作用する可能性があります。
Indeed, the delayed/ineffective adaptive responses and exaggerated inflammatory response can promote immunopathogenesis of COVID-19, particularly ARDS.
実際、遅延/無効な適応反応と過剰な炎症反応は、 COVID-19、特にARDSの免疫病因を促進する可能性があります。
Several lines of evidence from both human studies and animal model systems have shown that an effective T cell response is required to control and eradicate SARS-CoV-2 infection by releasing cytokines and other anti-inflammatory factors.
ヒト研究と動物モデル系の両方から得られたいくつかの証拠は、サイトカインやその他の抗炎症因子を放出することにより、SARS-CoV-2感染を制御し根絶するには効果的なT細胞応答が必要であることを示している。
During the infection, subepithelial DCs present SARS-CoV-2-specific peptides through MHC class I and II molecules on the cell surface, thus promoting the activation of CD8+ and CD4+ T cells, respectively, which migrate to the lung after antigen exposure.
感染中、上皮下DCは細胞表面のMHCクラスIおよびII分子を介してSARS-CoV-2特異的ペプチドを提示し、抗原曝露後に肺に遊走するそれぞれCD8+ T細胞とCD4+ T細胞の活性化を促進します。
Indeed, the lung is characterized by the presence of tissue-resident T cells with a memory phenotype (CD69+, CD103+/-, CD45RA,CCR7-) originated from the priming of naïve T cells.
実際、肺は、ナイーブ T 細胞のプライミングに由来する記憶表現型 (CD69+、CD103+/-、CD45RA、CCR7-) を持つ組織常在 T 細胞の存在を特徴としています。
Interestingly, an involvement of EVs in the regulation of antigen presentation and T cell activation has also been reported.
興味深いことに、抗原提示および T 細胞活性化の調節における EV の関与も報告されています。
While CD8+ T cells recognize and kill the infected cells, CD4+ T cells contribute to activate B cells for antibody secretion and CD8+ T cells to exert the cytotoxic activity, and to produce cytokines that favor immune cell migration at the site of infection.
CD8+ T細胞は感染細胞を認識して殺すのに対し、CD4+ T細胞は抗体分泌のためにB細胞を活性化し、CD8+ T細胞を活性化して細胞傷害活性を発揮し、感染部位での免疫細胞の遊走を促進するサイトカインの産生に寄与します。
Initial studies conducted by Grifoni and colleagues, and subsequently confirmed by others showed CD4+ and CD8+ viral specific T cell responses in most infected individuals mainly against spike antigen, although present also against other structural (nucleocapsid and membrane proteins) and non-structural SARS-CoV-2 antigens.
Grifoni らによって実施され、その後他の研究者によって確認された初期研究では、ほとんどの感染者において主にスパイク抗原に対する CD4+ および CD8+ ウイルス特異的 T 細胞反応が示されました。 ただし、他の構造抗原(ヌクレオカプシドおよび膜タンパク質)および非構造 SARS-CoV-2 抗原に対しても存在します。
Since spike protein has been identified as the most immunogenic antigen, it has been employed for many of the currently used SARS-CoV-2 vaccines.
スパイクタンパク質は最も免疫原性の高い抗原として特定されているため、現在使用されている SARS-CoV-2 ワクチンの多くに使用されています。
Unlike Mtb infection, the early development of antigen-specific T cell responses is generally observed within 7 days after the onset of COVID-19 symptoms, peaks at 14 days and may be detectable even if SARS-CoV-2 specific antibodies are lacking (Figure 1).
Mtb感染とは異なり、抗原特異的T細胞反応の初期発現は通常、COVID-19症状発現後7日以内に観察され、14日でピークに達し、SARS-CoV-2特異的抗体が欠如している場合でも検出できる可能性がある(図1)。
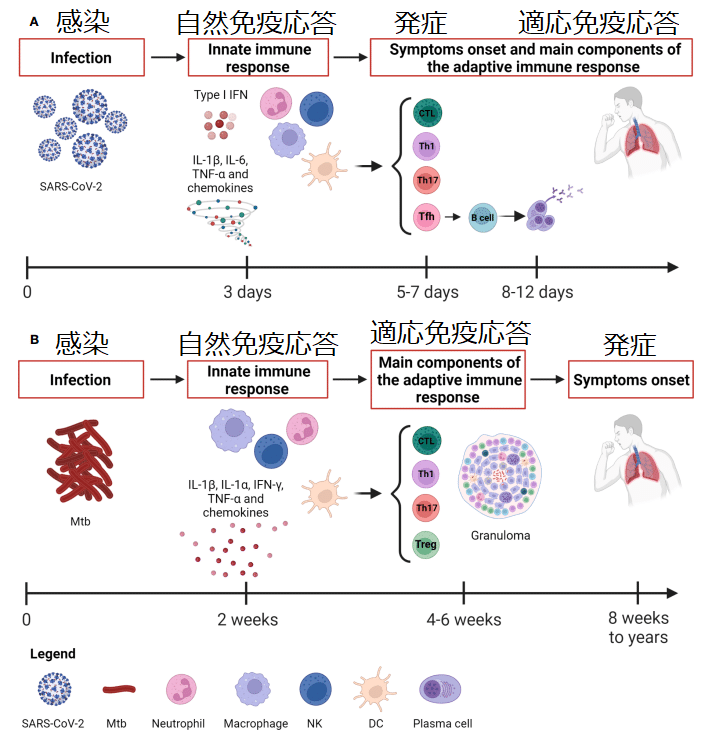
Several studies have shown that asymptomatic or pauci-symptomatic individuals are characterized by a strong SARS-CoV-2-specific CD4+ T cell response.
いくつかの研究では、無症候性または症状が少ない人は、SARS-CoV-2 に特異的な CD4+ T 細胞の強い応答を特徴とすることが示されています。
Surprisingly, CD4 +T cell responses were also observed in 40% to 60% of unexposed individuals likely because of the cross-recognition between SARS-CoV-2 and other “common cold” coronaviruses.
驚くべきことに、CD4 +T 細胞反応は、おそらく SARS-CoV-2 と他の「風邪」コロナウイルスの間の相互認識のため、未曝露の個人の 40% ~ 60% にも観察されました。
T cell activity has been associated with a less disease severity.
T 細胞の活性は疾患の重症度の軽減と関連しています。
The critical role played by T cells in the protection against the severe disease has been highlighted also with the occurrence of different VOCs with an increased ability to escape neutralizing antibodies.
重篤な疾患に対する防御において T 細胞が果たす重要な役割は、中和抗体から逃れる能力が増加したさまざまな VOC の発生によっても強調されています。
Indeed, the spike-specific T cell response induced by both vaccination and natural infection seems to be not affected by the amino acid mutations that characterize the VOCs, including Omicron, in healthy subjects and in the vulnerable populations.
実際、ワクチン接種と自然感染の両方によって誘導されるスパイク特異的 T 細胞応答は、健常者および脆弱な集団において、オミクロンを含む VOC を特徴付けるアミノ酸変異の影響を受けないようです。
Indeed, the availability of thousands of SARS-CoV-2 epitopes that may be recognized by T cells makes unlikely that the virus may successfully escape the T cell response by mutating the epitopes.
実際、T 細胞によって認識される可能性のある何千もの SARS-CoV-2 エピトープが利用可能であるため、ウイルスがエピトープを変異させることによって T 細胞応答をうまく回避できる可能性は低くなります。
SARS-CoV-2 infection mainly support the differentiation of CD4+ T lymphocytes toward T helper 1 (Th1), T helper 17 (Th17) and T follicular helper (Tfh) cells (Figure 2).
SARS-CoV-2 感染は主に、CD4+ T リンパ球の T ヘルパー 1 (Th1)、T ヘルパー 17 (Th17)、および T 濾胞ヘルパー (Tfh) 細胞への分化をサポートします (図 2)。
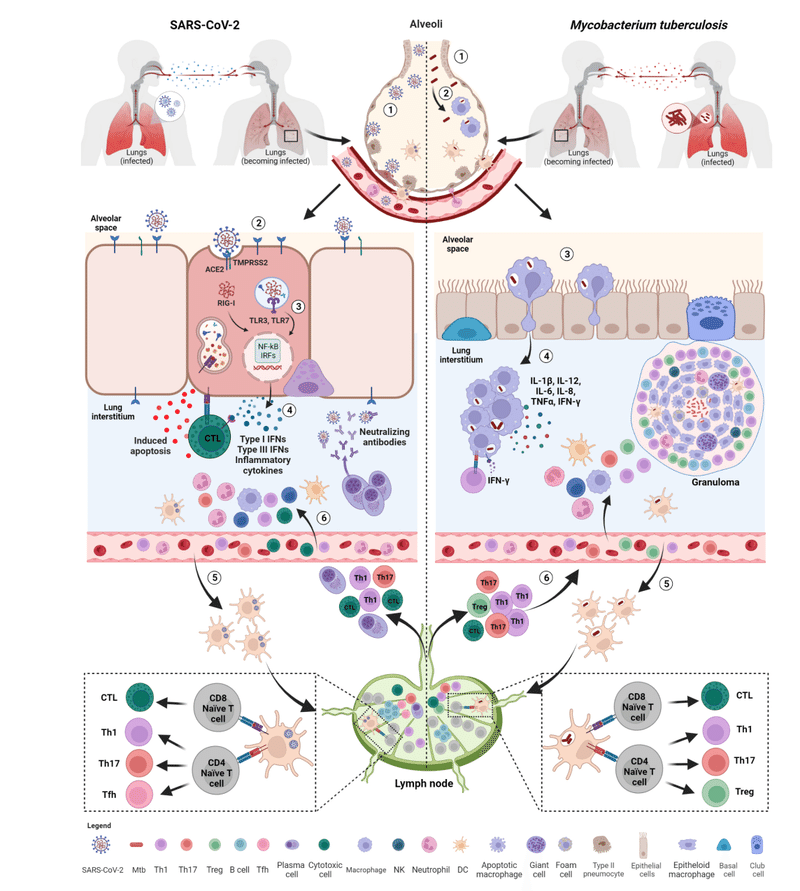
An appropriate Th1 immune response is necessary for protection against COVID-19, as an early and rapid expansion of IFN-γ-secreting SARS-CoV-2-specific T cells was detected over the course of acute infection and was associated with viral clearance and mild disease.
COVID-19に対する防御には、適切な Th1 免疫応答が必要です。 IFN-γを分泌するSARS-CoV-2特異的T細胞の早期かつ急速な増殖が急性感染の過程で検出され、ウイルス排除と軽度の疾患に関連していたためです。
Chauss and colleagues showed that asymptomatic SARS-CoV-2-infected individuals present in the BALF CD4+ T cells switched from a predominantly pro-inflammatory Th1 phenotype toward an IFN-γ and IL-10-producing phenotype that enable them the viral control without causing pathology.
Chaussらは次のことを示しました。つまり、無症候性SARS-CoV-2感染者のBALF CD4+ T細胞は、主に炎症促進性のTh1表現型からIFN-γおよびIL-10産生表現型に切り替わり、病状を引き起こすことなくウイルスを制御できるようになった。
The mechanism behind the switching phenotype is triggered by cell-intrinsic complement that orchestrates an autocrine/paracrine autoregulatory vitamin D (VitD) loop to initiate Th1 shutdown.
表現型の切り替えの背後にあるメカニズムは、オートクリン/パラクリン自動調節ビタミン D (VitD) ループを調整して Th1 シャットダウンを開始する細胞固有の補体によって引き起こされます。
During this process, Vitamin D induces epigenetic changes in the CD4+ T cells and recruits transcriptional factors, including c-JUN, STAT3 and BACH2 finally resulting in the switch off of Th1 programs and in the IL-10 induction.
このプロセス中に、ビタミン D は CD4+ T 細胞にエピジェネティックな変化を誘導し、c-JUN、STAT3、BACH2 などの転写因子を動員し、最終的に Th1 プログラムのスイッチをオフにして IL-10 誘導を引き起こします。
In patients with severe COVID-19 these regulatory processes are lacking and thus exacerbated Th1 cytokine profiles are prevail.
重度の COVID-19 患者では、これらの調節プロセスが欠如しているため、Th1 サイトカイン プロファイルの悪化が蔓延しています。
The lack of a fine-tuned Th1 immune response can cause an exacerbated reaction that precedes cytokine storm promoting the differentiation of Th2 cells that are related to a poor prognosis.
微調整された Th1 免疫応答の欠如は、予後不良に関連する Th2 細胞の分化を促進するサイトカインストームに先立つ反応の悪化を引き起こす可能性があります。
In this regard, Gil-Etayo and colleagues observed in COVID-19 patients a significant reduction in the percentage of Th1 and Th17 cells whereas a higher frequency of activated Th2 cells.
これに関して、Gil-Etayoらは、COVID-19患者において、Th1細胞とTh17細胞の割合が大幅に減少しているのに対し、活性化されたTh2細胞の頻度が高いことを観察した。
Moreover, a higher number of senescent Th2 cells together with higher levels of IL-15 were observed in patients with a fatal outcome.
さらに、致死的な結果をもたらした患者では、より多くの数の老化 Th2 細胞とより高いレベルの IL-15 が観察されました。
In addition, Th17 cells are strongly activated in severe COVID-19, thus favoring cell-mediated immunopathology through the production of IL-17 and GM-CSF.
さらに、Th17 細胞は重篤な COVID-19 において強く活性化されるため、IL-17 および GM-CSF の産生を通じて細胞性免疫病理が促進されます。
IL-17 released by Th17 cells induces the activation of monocytes/macrophages, DCs, and neutrophils which, in turn, increases the release of cytokines (IL-1, IL-6, IL-8, IL-21, TNF-α, and MCP-1), thus promoting the cytokine storm.
Th17 細胞によって放出される IL-17 は、単球/マクロファージ、DC、および好中球の活性化を誘導し、それによってサイトカイン(IL-1、IL-6、IL-8、IL-21、TNF-α、MCP-1)の放出が増加します。したがって、サイトカインストームが促進されます。
It has been reported that the polarization of CD4+ T cells toward Th17 instead of Th1 can be promoted by neutrophils as well as by the up-regulation of pro-inflammatory cytokines IL-1β, IL-6 and IL-23.
CD4+ T 細胞の Th1 ではなく Th17 への分極は、好中球によって、また炎症誘発性サイトカイン IL-1β、IL-6、および IL-23 の上方制御によって促進される可能性があることが報告されています。
Tfh cells are localized within the germinal centers of the secondary lymphoid organs and they are primarily involved in the activation and proliferation of B cells, and the production of high affinity antibodies as well as in the assistance of CD8+ T cell functions.
Tfh 細胞は二次リンパ器官の胚中心内に局在しており、主に B 細胞の活性化と増殖、高親和性抗体の産生、および CD8+ T 細胞機能の補助に関与しています。
In rhesus macaques CD8+ T cells are crucial for viral clearance especially when a reduced humoral response is present.
アカゲザルでは、特に体液性反応が低下している場合、CD8+ T 細胞がウイルスの除去に重要です。
In this regard, a weak CD8+ T cell response has been associated with a poor prognosis.
これに関して、弱い CD8+ T 細胞応答は予後不良と関連しています。
Indeed, a delayed or lacking CD8+ T cell response was found in patients with severe or fatal outcomes probably due to the inability of T cells to rapidly limit viral replication.
実際、おそらくT細胞がウイルス複製を迅速に制限できないため、重篤または致命的な転帰を示す患者ではCD8+ T細胞応答の遅延または欠如が見られました。
Besides CD4+ and CD8+ T cells, regulatory T cells (Treg) have been shown to play a critical role in SARS-CoV-2 infection, particularly as regulators of the inflammatory response.
CD4+ および CD8+ T 細胞に加えて、制御性 T 細胞 (Treg) が SARS-CoV-2 感染において、特に炎症反応の調節因子として重要な役割を果たすことが示されています。
Perturbations in Treg phenotype, such as the reduced expression of Foxp3 and cytokines including IL-10 and TGF-β, have been associated with disease severity.
Foxp3 や IL-10 や TGF-β などのサイトカインの発現低下などの Treg 表現型の乱れは、疾患の重症度と関連しています。
Quantitative and/or functional deficiency of T cells is associated with pathological processes responsible for tissue damage.
T 細胞の量的および/または機能的欠損は、組織損傷の原因となる病理学的プロセスに関連しています。
Indeed, a characteristic hallmark of severe COVID-19 is the peripheral lymphopenia accompanied by a reduced count of monocytes, eosinophils, basophils, but not neutrophils.
実際、重症COVID-19の特徴的な特徴は、単球、好酸球、好塩基球の数の減少を伴うが、好中球の数の減少を伴わない末梢リンパ球減少症である。
Possible explanations for T cell depletion is the SARS-CoV-2 infection of T cells through the binding of the spike protein to the CD147 or CD26 expressed on cell surface, their recruitment to infected site, or their apoptosis via Fas/Fas ligand or TNF.
T細胞枯渇の考えられる説明は、T細胞のSARS-CoV-2感染です。細胞表面に発現するCD147またはCD26へのスパイクタンパク質の結合、感染部位へのそれらの動員、またはFas/FasリガンドまたはTNFを介したアポトーシスを介して行われます。
Furthermore, increased levels of IL-6, IL-10 and TNF-α may contribute to lymphopenia.
さらに、IL-6、IL-10、および TNF-α のレベルの増加は、リンパ球減少症の一因となる可能性があります。
The prolonged peripheral lymphocytopenia increases the risk of secondary bacterial infections.
末梢リンパ球減少症が長期化すると、二次細菌感染のリスクが高まります。
Also, an immunosuppression following hyperinflammation in COVID-19 disease has been described, in particular NLRs and TLRs were shown to be associated to immunosuppression.
また、COVID-19 疾患における過剰炎症後の免疫抑制についても報告されており、特に NLR と TLR が免疫抑制に関連していることが示されています。
A reduced number of peripheral Treg cells has also been observed in severe cases of COVID-19, likely leading to the development of lung pathology.
COVID-19の重症例では末梢Treg細胞の数の減少も観察されており、肺病変の発症につながる可能性が高い。
As COVID-19 progresses, a different T cell functionality has also been observed.
COVID-19が進行するにつれて、異なる T 細胞の機能も観察されています。
Early during the acute phase of SARS-CoV-2 infection, T lymphocytes are characterized by a highly activated cytotoxic phenotype, whereas in convalescent individuals they show a polyfunctional and memory phenotype.
SARS-CoV-2 感染の急性期の初期には、T リンパ球は高度に活性化された細胞傷害性表現型を特徴としますが、回復期の個人では多機能性および記憶表現型を示します。
CD8+ T cells expressing markers of exhaustion such as PD-1+ TIM3+ increase over the infection and this scenario seems to be related to IL-10 blood levels.
PD-1+ TIM3+ などの疲労マーカーを発現する CD8+ T 細胞は感染症を超えて増加し、このシナリオは IL-10 血中濃度に関連していると思われます。
The hyper-activation of T cells along with the dysfunctionality of DCs and Tregs may increase the overwhelming alveoli inflammation and cytokine storm in COVID-19.
DC および Treg の機能不全に伴う T 細胞の過剰な活性化により、COVID-19における圧倒的な肺胞炎症とサイトカインストームが増加する可能性があります。
In light of what is reported in literature, an efficient T cell response is fundamental for viral clearance.
文献で報告されていることを考慮すると、効率的な T 細胞応答がウイルス除去の基本です。
Antibody response to SARS-CoV-2
SARS-CoV-2 に対する抗体反応
The antibody response usually appears by 1-2 weeks later than SARS-CoV-2 specific T cell response that is detectable 5-6 days post-infection (Figure 1).
抗体応答は通常、感染後 5 ~ 6 日で検出可能な SARS-CoV-2 特異的 T 細胞応答より 1 ~ 2 週間遅れて現れます (図 1)。
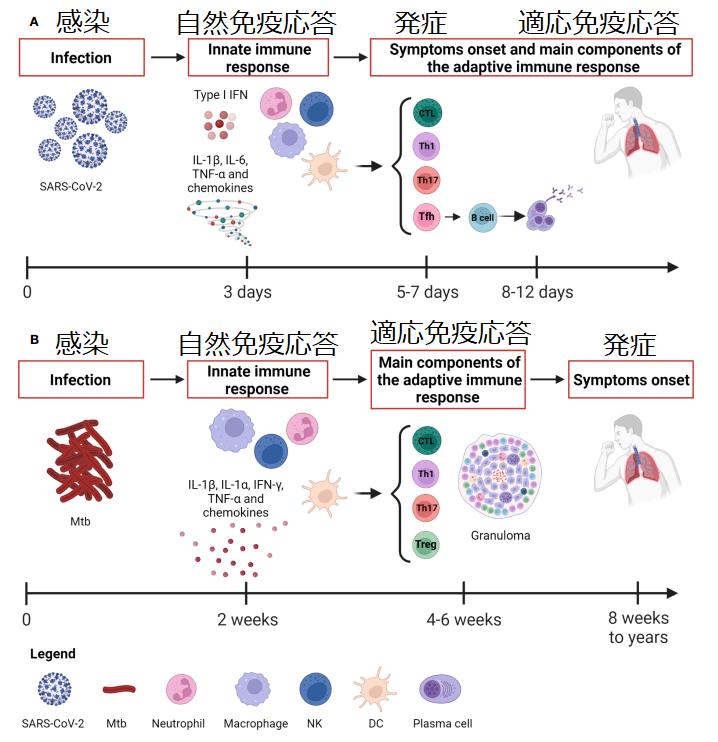
Within few days post-infection, B cells are rapidly activated in extrafollicular foci to differentiate in short-lived plasma cells that predominantly produce IgM antibodies but also IgG or IgA-switched to initially stem viral infection, while waiting for the production of antibodies with higher affinity.
感染後数日以内に、B 細胞は濾胞外病巣で急速に活性化され、短命の形質細胞に分化します。 主に IgM 抗体を産生しますが、より高い親和性を持つ抗体の産生を待つ間、最初にウイルス感染を阻止するために IgG または IgA に切り替えられる抗体も産生します。
The first IgM, IgA and IgG are measurable in the sera between 8 and 12 days after symptom onset.
最初の IgM、IgA、および IgG は、症状発症後 8 ~ 12 日間の血清中で測定可能です。
Subsequently, within the germinal centers in the secondary lymphoid organs, antigen-specific B cells undergo somatic hypermutation and isotype-switching resulting in the production of high-affinity IgG antibodies that mainly recognize nucleocapsid and spike proteins.
続いて、二次リンパ器官の胚中心内で、抗原特異的 B 細胞が体細胞超変異とアイソタイプスイッチングを受け、主にヌクレオカプシドとスパイクタンパク質を認識する高親和性 IgG 抗体が産生されます。
Cross-sectional and longitudinal studies showed that Enzyme-linked immunosorbent assay (ELISA) titers and neutralizing antibodies are detectable around 14 days after symptom onset, peak in 3 to 4 weeks, and decline subsequently causing a reduction of protection and increasing the risk of SARS-CoV-2 re-infection.
横断的および縦断的研究により、酵素結合免疫吸着検定法(ELISA)力価と中和抗体は症状発現後約 14 日で検出可能となり、3 ~ 4 週間でピークに達することが示されました。 その後減少し、防御力の低下を引き起こし、SARS-CoV-2 再感染のリスクを高めます。
However, it has been observed that anti-RBD antibodies, neutralizing activity and RBD-specific memory B cells are mostly stable between 6 and 12 months after infection, likely owing to the presence of a long-lived plasma cell compartment located in the bone marrow.
ただし、抗 RBD 抗体、中和活性、および RBD 特異的メモリー B 細胞は、感染後 6 ~ 12 か月の間はほとんど安定していることが観察されています。 おそらく骨髄に長命の形質細胞区画が存在するためと考えられます。
The protective role of the antibodies is limited to those specific for the viral spike protein because they neutralize the virus by hindering the binding between spike and ACE2 receptor and thus blocking its entry, and by promoting effector functions via the binding to the complement and Fc receptors.
抗体の保護的役割は、ウイルスのスパイクタンパク質に特異的なものに限定されています。なぜなら、それらはスパイクとACE2受容体の間の結合を妨げてウイルスの侵入をブロックし、補体とFc受容体への結合を介してエフェクター機能を促進することによってウイルスを中和するからです。
In the case of neutralizing antibodies, the engagement of Fc receptors can potentiate neutralization.
中和抗体の場合、Fc 受容体の結合により中和が強化される可能性があります。
Non-neutralizing antibodies may promote antibody-dependent cellular cytotoxicity (ADCC) and antibody-dependent cellular phagocytosis (ADCP).
非中和抗体は、抗体依存性細胞傷害性 (ADCC) および抗体依存性細胞食作用 (ADCP) を促進する可能性があります。
In this regard, high ADCC activities are detected mainly in hospitalized patients and showed a kinetic similar to antibody titers with a peak at 2-4 weeks post-infection followed by a gradual decline.
これに関して、高い ADCC 活性は主に入院患者で検出され、感染後 2 ~ 4 週間でピークに達し、その後徐々に低下する抗体力価と同様の動態を示しました。
Most of the antibodies are directed against epitopes localized in the receptor-binding motif (RBM) within the RBD of spike, whereas a minority is directed against the N-terminal domain (NTD).
ほとんどの抗体はスパイクの RBD 内の受容体結合モチーフ (RBM) に局在するエピトープに対して向けられていますが、少数は N 末端ドメイン (NTD) に対して向けられています。
Anti-NTD antibodies have less neutralizing activity than anti-RBD antibodies and they may act by interfering with the conformational changes necessary for fusion or binding to receptors such as transmembrane lectins DC-SIGN, L-SIGN and SIGLEC1.
抗 NTD 抗体は抗 RBD 抗体よりも中和活性が低く、膜貫通レクチン DC-SIGN、L-SIGN、SIGLEC1 などの受容体との融合または結合に必要な構造変化を妨げることによって作用する可能性があります。
The antibody response, either qualitative and quantitative, is dependent on the amount of the antigen and on the activity of the germinal centers.
抗体反応は、定性的および定量的に、抗原の量と胚中心の活性に依存します。
In this regard, patients with severe COVID-19 show higher titers of total and neutralizing antibodies than mild or asymptomatic patients, likely due to the stronger antigen response.
この点において、重度COVID-19患者は、軽度または無症状の患者よりも高い総抗体および中和抗体の力価を示しますが、これはおそらく抗原反応が強いためと考えられます。
On the other hand, individuals undergoing B-cell depleting therapies, such as anti-CD20, show an impaired antibody response that is associated with a more severe course of COVID-19.
一方で、抗CD20などのB細胞除去療法を受けている人は、COVID-19のより重篤な経過に関連する抗体反応の低下を示します。
While circulating antibodies may help to control viral dissemination within the host, mucosal antibodies such as the dimeric form of IgA that is secreted in the upper respiratory tract, play an important role in preventing the transmission of SARS-CoV-2, present a stronger neutralizing activity than circulating antibodies, and contribute to protection against re-infection.
循環する抗体は宿主内でのウイルスの蔓延を制御するのに役立つ可能性がありますが、上気道で分泌される二量体型 IgA などの粘膜抗体は、 SARS-CoV-2の感染予防に重要な役割を果たし、循環抗体よりも強力な中和活性を示し、再感染に対する防御に貢献します。
Indeed, SARS-CoV-2 specific IgA have been found in saliva samples collected from infected individuals.
実際、SARS-CoV-2 特異的 IgA は、感染者から採取された唾液サンプルから検出されています。
During SARS-CoV-2 infection, also autoantibodies targeting self-antigens, including type I IFN, were identified in some COVID-19 patients, particularly in those with a severe disease that are characterized by a reduced IFN production, as mentioned above.
SARS-CoV-2感染中に、一部のCOVID-19患者では、I型IFNを含む自己抗原を標的とする自己抗体も同定された。 特に、上述したように、IFN産生の低下を特徴とする重篤な疾患を患っている患者においてはそうである。
COVID-19 patients are also characterized by changes in B-cell subpopulations.
COVID-19患者は、B 細胞亜集団の変化によっても特徴付けられます。
In particular, increased number of proliferating, metabolically hyperactive plasma blasts and reduction of memory B cells have been found in patients with severe disease, whereas they disappeared with convalescence.
特に、増殖し代謝亢進している血漿芽細胞の数の増加と記憶B細胞の減少が重症患者で見出されているが、これらは回復とともに消失します。
Nonetheless, SARS-CoV-2 has evolved different strategies to escape the immune response.
それにもかかわらず、SARS-CoV-2 は免疫反応から逃れるためにさまざまな戦略を進化させてきました。
Unlike bacteria such as Mtb, RNA viruses are usually characterized by high mutation rates.
Mtb などの細菌とは異なり、RNA ウイルスは通常、高い突然変異率を特徴とします。
SARS-CoV-2 exploits this ability to accumulate mutations in the spike protein in order to avoid the immune recognition by neutralizing antibodies and to increase its transmissibility.
SARS-CoV-2は、中和抗体による免疫認識を回避し、感染力を高めるために、この能力を利用してスパイクタンパク質に変異を蓄積します。
In particular, the emerging VOCs has accumulated mutations mainly located in the RBM, in part due to the pressure exerted by the host immune system.
特に、新たに出現した VOC は、宿主の免疫系による圧力の影響もあり、主に RBM に変異を蓄積しています。
It has been proposed that the concurrent onset of multiple mutations in the spike protein might occur during the prolonged infection in immunocompromised patients resulting in the emergence of variant strains.
免疫不全患者の長期感染中にスパイクタンパク質の複数の変異が同時に発生し、その結果、変異株が出現する可能性があることが提案されています。
These mutations increased affinity of the virus for the ACE2 receptor and improved its ability to evade the neutralizing antibody response induced by natural infection or following vaccination with the spike protein derived from the ancestral strain.
これらの変異により、ACE2受容体に対するウイルスの親和性が高まり、自然感染や祖先株由来のスパイクタンパク質によるワクチン接種後に誘発される中和抗体反応を回避する能力が向上しました。
Altogether, the humoral response has been shown to play a crucial role in the host immune protection against SARS-CoV-2 together with the T cell response.
まとめると、体液性応答は、T 細胞応答とともに、SARS-CoV-2 に対する宿主免疫防御において重要な役割を果たすことが示されています。
T cell response to M. tuberculosis
結核菌に対する T 細胞の反応
The infected monocytes, macrophages and DCs are thought to be key elements leading to Mtb dissemination and granuloma formation.
感染した単球、マクロファージ、および DC は、Mtb の播種と肉芽腫の形成につながる重要な要素であると考えられています。
The infected professional antigen-presenting DCs travel to the lung draining lymph nodes where priming of naïve CD4+ and CD8+ T cells is initiated.
感染したプロフェッショナル抗原提示 DC は肺に流入するリンパ節に移動し、そこでナイーブ CD4+ および CD8+ T 細胞のプライミングが開始されます。
Priming is a critical step for the initiation of the adaptive immunity that is crucial to hinder bacilli dissemination and control the infection.
プライミングは、桿菌の蔓延を妨げ、感染を制御するために重要な適応免疫を開始するための重要なステップです。
However, the adaptive (T cell) response takes longer to appear in infected hosts because Mtb or its antigens are transported late into the lymph nodes for T cell priming.
ただし、Mtb またはその抗原が T 細胞プライミングのためにリンパ節に輸送されるのが遅いため、感染した宿主では適応 (T 細胞) 応答が現れるまでに時間がかかります。
In mice this occurs within 2-3 weeks post-infection, but in humans and non-human primates Mtb-specific T cell response in the periphery, measured as a response to TST, or IGRA, is usually not detectable until 4–6 weeks post-infection.
マウスの場合、これは感染後 2 ~ 3 週間以内に起こります。 しかし、ヒトおよびヒト以外の霊長類では、TSTまたはIGRAに対する応答として測定される末梢におけるMtb特異的T細胞応答は、通常、感染後4~6週間まで検出できません。
It was found that Mtb-infected DCs in the lymph node are capable to release soluble and intact Mtb antigens that can be caught by uninfected DCs and efficiently presented to naïve CD4+ T cells to optimize CD4+ T cell priming and to initiate the adaptive immune response.
リンパ節内のMtb感染DCは、未感染DCによって捕捉され、ナイーブCD4+ T細胞に効率的に提示され、CD4+ T細胞のプライミングを最適化し、適応免疫応答を開始することができる可溶性かつ無傷のMtb抗原を放出することができることが判明しました。
Surprisingly, the capacity of Mtb-infected DCs in activation and proliferation of naïve Mtb-specific CD4+ T cells in the murine lymph node was found to be impaired likely due to lower MHC class II-peptide presentation by these infected APCs.
驚くべきことに、マウスリンパ節におけるナイーブMtb特異的CD4+ T細胞の活性化および増殖におけるMtb感染DCの能力が損なわれていることが判明した。 おそらく、これらの感染した APC による MHC クラス II ペプチド提示の低下が原因と考えられます。
The primed T (and likely B) lymphocytes can then move to the site of infection and contribute to the formation of the organized granuloma that consists of modified macrophages as epithelioid cells and multinucleated giant cells accompanied by neutrophils and DCs in the center, infiltrated immune cells including granulocytes, antigen-specific T cells and few B cells in the periphery, with variable degrees of fibrosis or central caseous necrosis (Figure 2).
その後、プライムされた T リンパ球は感染部位に移動し、修飾されたマクロファージからなる組織化された肉芽腫の形成に寄与します。 中心部には類上皮細胞および多核巨細胞が好中球および樹状細胞を伴い、末梢部には顆粒球、抗原特異的T細胞および少数のB細胞を含む浸潤免疫細胞が存在し、さまざまな程度の線維化または中心乾酪壊死を伴います(図2)。
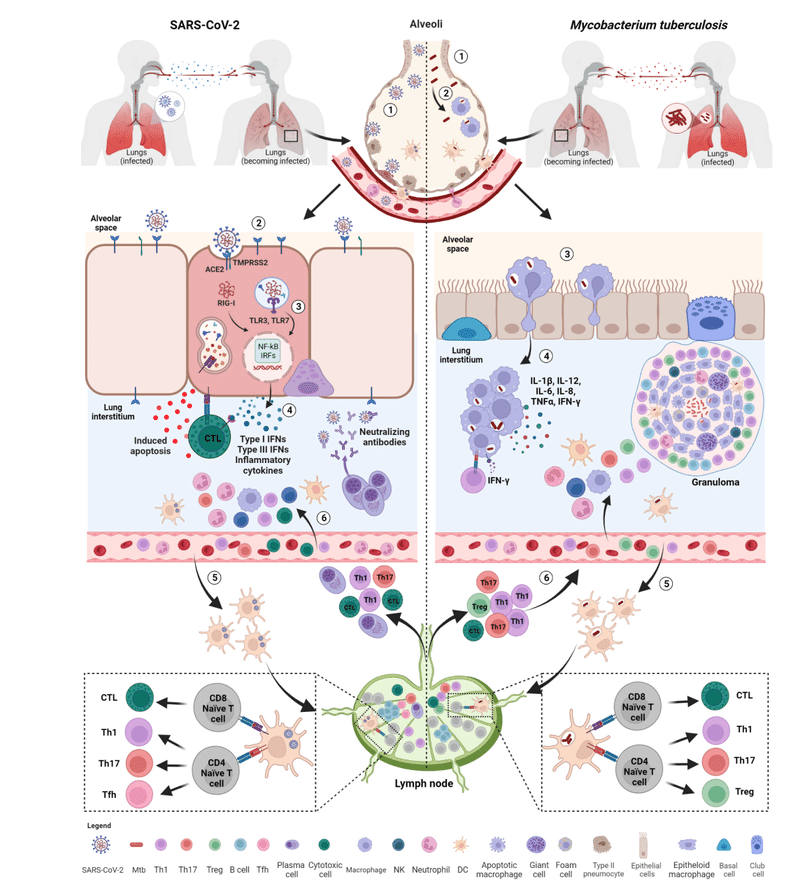
Although the mechanisms driving protection and pathology within the granuloma microenvironments are still poorly understood, such mechanisms can be very important for the prognosis, and outcome of the disease.
肉芽腫微小環境内の保護と病理を推進するメカニズムはまだほとんど理解されていませんが、そのようなメカニズムは病気の予後および転帰にとって非常に重要である可能性があります。
Notably, granuloma structure and function protect the host from the dissemination of the infection, but it is also a way to facilitate the persistency of the infection.
特に、肉芽腫の構造と機能は宿主を感染の蔓延から保護しますが、感染の持続を促進する方法でもあります。
In fact, sterilizing immunity following Mtb infection is rare and even in the presence of a robust adaptive immune response to Mtb, the nature of the granulomas as well as the immune escape mechanisms of Mtb can restrict the host immune response to reliably eliminate the infection.
実際、Mtb 感染後の滅菌免疫はまれであり、Mtb に対する強力な適応免疫応答が存在する場合でも、肉芽腫の性質と Mtb の免疫回避機構により宿主の感染を確実に排除するための免疫応答が制限されます。
This leads to develop a controlled infection, traditionally called latent infection, in most infected individuals.
これにより、ほとんどの感染者は、伝統的に潜伏感染と呼ばれる制御された感染を発症します。
Mtb can survive in a dormant (non-replicating) state favored by hypoxic conditions inside solid granulomas that makes it difficult to be detected by the immune system.
Mtb は、免疫系による検出が困難な固形肉芽腫内部の低酸素状態により有利な休眠 (非複製) 状態で生存できます。
Within the granuloma, Mtb antigens persistently stimulate immune cells leading to immune activation, chronic inflammation, and finally cell exhaustion.
肉芽腫内では、Mtb 抗原が免疫細胞を持続的に刺激し、免疫の活性化、慢性炎症、そして最終的には細胞の枯渇を引き起こします。
Different T cell types and functions can exert a beneficial or even detrimental role.
さまざまな T 細胞の種類と機能が、有益な役割を果たしたり、有害な役割を果たしたりすることがあります。
The peripheral localization of T cells restricts their access to the central core of the granuloma, where Mtb-infected macrophages reside, and this can limit the interactions between macrophages and lymphocytes.
T細胞の末梢局在化により、Mtb感染マクロファージが存在する肉芽腫の中心核へのアクセスが制限され、これによりマクロファージとリンパ球間の相互作用が制限される可能性があります。
Moreover, a Mtb-induced immunosuppressive environment has been indicated in the granuloma in which IL-10 impairs Th1 activity and lysis of infected macrophages.
さらに、肉芽腫ではMtb誘導性の免疫抑制環境が示されており、IL-10はTh1活性と感染したマクロファージの溶解を損ないます。
The important role for T-cell immunity and particularly IFN-γ-producing Th1 in controlling Mtb infection has been demonstrated in humans and animal models.
Mtb 感染の制御における T 細胞免疫、特に IFN-γ 産生 Th1 の重要な役割は、ヒトおよび動物モデルで実証されています。
IFN-γ is a key factor involved in CD4+ T cell-mediated protection by increasing autophagy and promoting phagosome maturation in macrophages inducing the production of antimicrobial peptides, and limits the accumulation of non-protective CD4+ T cells in the lung vasculature.
IFN-γは、オートファジーを増加させ、抗菌ペプチドの産生を誘導するマクロファージのファゴソーム成熟を促進することにより、CD4+ T細胞を介した防御に関与する重要な因子であり、肺血管系における非防御的なCD4+ T細胞の蓄積を制限します。
In humans, HIV infection appears to be an important risk factor for TB disease progression likely due to CD4+ T cell depletion.
ヒトでは、HIV 感染はおそらく CD4+ T 細胞の枯渇による結核疾患進行の重要な危険因子であると考えられます。
Also, depletion of CD4+ T cells in cynomolgus macaques with acute Mtb infection leads to exacerbated disease in most animals.
また、急性Mtb感染を伴うカニクイザルのCD4+ T細胞の枯渇は、ほとんどの動物で疾患の悪化につながります。
Moreover, TB disease increases HIV replication, in vivo and in vitro through a mechanism of immune activation.
さらに、結核疾患は、免疫活性化のメカニズムを通じて、インビボおよびインビトロで HIV 複製を増加させます。
The activation and proliferation of antigen-specific naïve CD4+ T cell subsets strongly depends on the cytokine milieu released by APCs.
抗原特異的なナイーブ CD4+ T 細胞サブセットの活性化と増殖は、APC によって放出されるサイトカイン環境に強く依存します。
Particularly, macrophages are the main source of IL-1β, IL-6, IL-18, TNF-α, IL-10, and TGF-β, while DCs are the main producers of IL-12, IL-23, IL-27 and IFN-β.
特に、マクロファージは、IL-1β、IL-6、IL-18、TNF-α、IL-10、および TGF-β の主な供給源です。 一方、DC は IL-12、IL-23、IL-27、および IFN-β の主な生産者です。
For instance, IL-12 produced by DCs differentiates naïve CD4+ T cells to Th1 which promote activation of the cell-mediated immunity needed to counteract intracellular pathogens.
例えば、DCによって産生されるIL-12は、ナイーブCD4+ T細胞をTh1に分化させ、細胞内病原体に対抗するために必要な細胞性免疫の活性化を促進します。
These cells secrete pro-inflammatory cytokines such as IL-2, IFN-γ and TNF-α to activate macrophages and cytotoxic CD8+ T cells.
これらの細胞は、IL-2、IFN-γ、TNF-αなどの炎症誘発性サイトカインを分泌して、マクロファージや細胞傷害性CD8+ T細胞を活性化します。
TNF-α is known to be necessary for the formation of a well-organized granuloma and host protection, as confirmed by the higher risk of developing TB disease and disseminated infection in subjects who underwent anti-TNF-α treatment.
TNF-αは、抗TNF-α治療を受けた被験者における結核疾患および播種性感染症の発症リスクが高いことからも確認されるように、よく組織化された肉芽腫の形成と宿主保護に必要であることが知られています。
Activated cytotoxic CD8+ T cells and macrophages kill and eliminate pathogens and infected host cells by cytotoxic effector molecules such as perforin, granzymes and granulysin and by death receptor/ligand ligation.
活性化された細胞傷害性 CD8+ T 細胞およびマクロファージは、パーフォリン、グランザイム、グラニュライシンなどの細胞傷害性エフェクター分子およびデスレセプター/リガンドのライゲーションによって、病原体および感染した宿主細胞を殺し、排除します。
Furthermore, IL-23 produced by DCs drives differentiation and functionality of Th17 cells that produce IL-17 which is a cytokine involved in neutrophil recruitment.
さらに、DCによって産生されるIL-23は、好中球の動員に関与するサイトカインであるIL-17を産生するTh17細胞の分化と機能を促進します。
IL-17 signaling appears to be essential for recruiting neutrophils to the site of infection early after Mtb infection in murine models, but a dysregulated production of this cytokine was also found to be associated with immunopathology driven by excess neutrophil recruitment and inflammation.
IL-17シグナル伝達は、マウスモデルにおけるMtb感染後の早期に感染部位に好中球を動員するために不可欠であると思われるが、このサイトカインの調節不全な産生は、過剰な好中球の動員と炎症によって引き起こされる免疫病理と関連していることも判明しました。
Although inflammation is required for an effective immune response against harmful pathogens, the balance between pro- and anti-inflammatory cytokines is critical to control the disease and lung damage during Mtb infection.
有害な病原体に対する効果的な免疫反応には炎症が必要ですが、Mtb 感染時の疾患や肺損傷を制御するには、炎症促進性サイトカインと抗炎症性サイトカインのバランスが重要です。
Anti-inflammatory cytokines such as IL-4, IL-5, IL-13 released by Th2 cells and IL-10 and TGF-β by regulatory T cells are needed to suppress inflammation during immune response.
免疫応答中の炎症を抑制するには、Th2細胞によって放出されるIL-4、IL-5、IL-13、制御性T細胞によって放出されるIL-10およびTGF-βなどの抗炎症性サイトカインが必要です。
However, these cells may promote long-term persistence of Mtb by favoring active immunosuppression rather than the expected tissue repair response.
しかし、これらの細胞は、予想される組織修復反応ではなく能動的な免疫抑制を優先することにより、Mtb の長期持続を促進する可能性があります。
In patients with TB disease, TST positive, in vitro PPD stimulation induced the production of IL-10, IFN-γ, and cell proliferation, whereas in those TST-negative PPD induced IL-10 but not IFN-γ release, without cell proliferation.
結核疾患患者では、TST陽性のin vitro PPD刺激によりIL-10、IFN-γの産生および細胞増殖が誘導されたが、TST陰性のPPDではIL-10の放出は誘導されたが、細胞増殖はなくIFN-γの放出は誘導されなかった。
Altogether, a better understanding of the dynamically balanced immune response is fundamental for therapeutic strategies and subsequently for vaccine development.
総合すると、動的にバランスがとれた免疫応答をより深く理解することは、治療戦略、ひいてはワクチン開発の基礎となります。
The role of B cells and antibodies in TB
結核におけるB細胞と抗体の役割
Although Mtb infection induces strong antibody responses, the role of antibodies and B cells in TB has not been fully elucidated.
Mtb 感染は強力な抗体反応を誘導しますが、結核における抗体と B 細胞の役割は完全には解明されていません。
Previous studies on B cell depletion have failed to definitively establish a role for these cells or antibodies in Mtb infection and control, although recent studies have demonstrated potentially protective roles of antibodies in humans and non-human primates (NHPs) after intravenous bacille Calmette-Guérin (BCG) vaccination.
B細胞の枯渇に関するこれまでの研究では、Mtbの感染と制御におけるこれらの細胞または抗体の役割を明確に確立することができませんでした。 しかし、最近の研究では、カルメット・ゲラン桿菌(BCG)の静脈内ワクチン接種後のヒトおよび非ヒト霊長類(NHP)における抗体の潜在的な保護的役割が実証されています。
It has been shown that TB disease is associated with decreased B cell count and function compared with individuals who are infected with Mtb but without any clinical symptoms, suggesting that TB patients may be less able to develop successful antibody responses against Mtb.
結核疾患は、Mtb に感染しているが臨床症状を示さない人に比べて、B 細胞の数と機能の減少に関連していることが示されており、結核患者は Mtb に対する抗体反応をうまく発現させる能力が低い可能性があることが示唆されています。
Moreover, distinct glycosylation patterns on the Fc part of the antibodies, and isotype skewing to less potently immune-activating variants like IgG4 have been considered for this altered functional response.
さらに、抗体の Fc 部分の異なるグリコシル化パターン、および IgG4 のような強力ではない免疫活性化バリアントへのアイソタイプの偏りが、この機能的応答の変化について考慮されています。
Surprisingly, heavily Mtb-exposed individuals who “resisted” to infection showed higher antibody functionality compared to those with TB infection, indicating an important role of antibodies in early protective immunity.
驚くべきことに、Mtb に重度に曝露され、感染に「抵抗」した人は、結核感染者と比較してより高い抗体機能を示し、初期の防御免疫における抗体の重要な役割を示しました。
Studies have shown that the interaction of Mtb with macrophages can be affected by antibodies in a variety of ways.
研究により、Mtb とマクロファージの相互作用は、抗体によってさまざまな方法で影響を受ける可能性があることが示されています。
For instance, bacterial opsonization may alter vesicular trafficking and macrophage signaling.
たとえば、細菌のオプソニン作用は小胞輸送やマクロファージのシグナル伝達を変化させる可能性があります。
Moreover, the binding of antibodies to Fc receptors (activator or inhibitory) on macrophages can modulate their function.
さらに、マクロファージ上の Fc 受容体 (活性化因子または阻害因子) への抗体の結合は、その機能を調節する可能性があります。
Together, data suggest that B cells and antibodies may play an important role in protective immunity against mycobacterial infections; however, the diversity of antibody functions, the heterogeneity of the humoral immune response to Mtb, as well as the complexity of the interactions between B cells and other immune cells have been indicated as the major challenges to understand the impact of the humoral immune system in the immune protection at each stage of Mtb infection.
これらのデータを総合すると、B 細胞と抗体がマイコバクテリア感染に対する防御免疫において重要な役割を果たしている可能性があることが示唆されています;ただし、抗体機能の多様性、Mtb に対する体液性免疫応答の不均一性、 また、B 細胞と他の免疫細胞間の相互作用の複雑さも、Mtb 感染の各段階での免疫防御における体液性免疫系の影響を理解するための大きな課題として指摘されています。
M. tuberculosis and SARS-CoV-2 co-infection
結核菌と SARS-CoV-2 の同時感染
Information on TB-COVID-19 co-infection in humans is still limited.
ヒトにおける結核とCOVID-19の同時感染に関する情報はまだ限られています。
Co-infection was reported around 1% in the Philippines, 5% in South Africa, and between 0.37% and 4.47% in China.
同時感染はフィリピンで約1%、南アフリカで5%、中国で0.37~4.47%と報告された。
Recent works suggest that TB-COVID-19 co-infection is associated with elevated risk of unfavorable clinical outcome, with a longer time to recovery, treatment failure, loss to follow-up rates, and higher rates of mortality compared to patients with COVID-19 alone.
最近の研究では、結核とCOVID-19の同時感染は好ましくない臨床転帰のリスク上昇と関連していることが示唆されています。 新型コロナウイルス感染症単独の患者と比べて、回復までの時間が長く、治療の失敗、追跡調査の失敗、死亡率が高い。
However, mechanistic studies are needed to understand the interactions during Mtb and SARS-CoV-2 dual infections, their effect on the host immune response and clinical outcomes.
ただし、MtbとSARS-CoV-2の二重感染時の相互作用、宿主の免疫応答および臨床転帰に対するそれらの影響を理解するには、メカニズムの研究が必要です。
Understanding the early events and pathophysiology of TB-COVID-19 co-infection is warranted to find better ways to manage such cases, particularly in the high TB endemic areas.
結核とCOVID-19の同時感染の初期の出来事と病態生理学を理解することは、特に結核が流行している地域において、そのような症例を管理するより良い方法を見つけるために必要です。
The dysregulated immune response induced by each pathogen can lead to an unbalanced inflammatory response, which can promote the progression and worsening of both diseases.
各病原体によって誘発される免疫応答の調節不全は、不均衡な炎症反応を引き起こす可能性があり、それが両方の疾患の進行と悪化を促進する可能性があります。
To date, the immune response for each pathogen has been well studied, whereas the impact of Mtb and SARS-CoV-2 co-infection on the innate and adaptive immune response, their crosstalk and cumulative impact on disease outcome in humans still need to be delineated.
現在までに、各病原体に対する免疫応答は十分に研究されています。 一方、Mtb と SARS-CoV-2 の同時感染が自然免疫応答および適応免疫応答に及ぼす影響、それらのクロストーク、およびヒトの疾患転帰に対する累積的影響については、まだ詳しく説明する必要があります。
In fact, the studies available have mostly focused on the clinical features of co-infected patients, characterizing a marked lymphopenia and increased levels of some markers of inflammation, such as C-reactive protein (CRP), D-dimer, ferritin, and describing the lung tissue damages.
実際、利用可能な研究は主に、重度感染した患者の臨床的特徴に焦点を当てており、顕著なリンパ球減少と一部の炎症マーカーのレベルの上昇を特徴づけています。 C反応性タンパク質(CRP)、Dダイマー、フェリチンなど、肺組織の損傷について説明します。
There are few published studies either in vitro, ex-vivo using human samples from co-infected individuals or animal models evaluating the immune response and immunopathology in the context of co-infection (Table 2).
共感染者からのヒトサンプルまたは動物モデルを使用して、共感染の状況における免疫応答および免疫病理学を評価する、インビトロ、エクスビボのいずれかでの発表された研究はほとんどありません(表2)。
In vitro studies were recently performed by Sheerin and colleagues using a single-cell RNA-seq (scRNA-seq) approach to analyze the results from a co-infection performed using a whole blood platform (24 or 96 hours) from healthy adults.
最近、Sheerinらによって、健康な成人からの全血プラットフォーム(24時間または96時間)を使用して実行された同時感染の結果を分析するために、単一細胞RNA-seq(scRNA-seq)アプローチを使用したインビトロ研究が実施されました。
The authors characterized different and overlapping immunological responses generated by SARS-CoV-2 (ancestral strain) and Mtb (lineage 4 laboratory strain H37Rv) when a single infection or co-infection occurs.
著者らは、単一感染または同時感染が発生した場合に、SARS-CoV-2(祖先株)およびMtb(系統4の実験株H37Rv)によって生成される、異なる重複する免疫学的反応を特徴付けました。
Based on marker gene expression, they identified 13 distinct clusters of cells showing diverse proportions of monocytes, T cells and neutrophils between different conditions and timepoints.
彼らは、マーカー遺伝子の発現に基づいて、異なる条件および時点間で単球、T 細胞、好中球の割合が異なる 13 個の異なる細胞クラスターを特定しました。
The co-infected condition showed the major immune activation effect early (24h) post-infection with 238 immunological pathways uniquely enriched, including IFN-γ and TNF production, while 182 shared pathways were overlapping at 96h post-infection among different conditions.
同時感染状態では、IFN-γ および TNF 産生を含む 238 の免疫学的経路が独自に濃縮され、感染後早期 (24 時間) に主要な免疫活性化効果が示されました。 一方、感染後96時間の時点では、異なる条件間で182の共有経路が重複していた。
In contrast to SARS-CoV-2-only infection that caused extensive cell death by 96h post-infection, Mtb-only and co-infected conditions maintained monocyte, T cell and NK cell signatures, and negative regulation of the signaling of extrinsic apoptosis.
感染後 96 時間までに広範な細胞死を引き起こした SARS-CoV-2 のみの感染とは対照的に、Mtb のみおよび同時感染した条件では、単球、T 細胞および NK 細胞のサイン、および外因性アポトーシスのシグナル伝達の負の制御が維持されました。
Interesting animal studies evaluating the impact of aerosol Mtb and SARS-CoV-2 co-infection in transgenic (K18-hACE2) C57BL/6 mice showed that pre-infection with Mtb resulted in lower SARS-CoV-2 viral loads at the lung tissue level, likely mediated by the heightened immune microenvironment of the lungs.
トランスジェニック (K18-hACE2) C57BL/6 マウスにおけるエアロゾル Mtb と SARS-CoV-2 の同時感染の影響を評価した興味深い動物研究では、次のことが示されました。 Mtb による前感染により、肺組織レベルでの SARS-CoV-2 ウイルス量が低下しましたが、これはおそらく肺の免疫微小環境の高まりによって媒介されたと考えられます。
In addition, after SARS-CoV-2 superinfection, increased bacterial loads in Mtb-infected tissues and decreased histiocytic inflammation were found.
さらに、SARS-CoV-2 重感染後、Mtb 感染組織の細菌量の増加と組織球性炎症の減少が見られました。
Moreover, SARS-CoV-2 caused a decreasing trend in type 1 (IFN-γ and TNF-α) and an increasing trend in type 2 (IL-4 and IL-13) cytokine transcript levels in Mtb-infected mice.
さらに、SARS-CoV-2は、Mtb感染マウスにおいて1型(IFN-γおよびTNF-α)サイトカイン転写レベルの減少傾向と2型(IL-4およびIL-13)サイトカイン転写レベルの増加傾向を引き起こした。
These findings, which are usually associated with disseminated Mtb infection, suggest that SARS-CoV-2 may have a deleterious effect on TB outcome through the immune dysregulation, potentially resulting in granuloma collapse and the subsequent Mtb dissemination.
これらの所見は通常、播種性Mtb感染に関連しており、SARS-CoV-2が免疫調節異常を通じて結核の転帰に悪影響を及ぼし、肉芽腫の崩壊とそれに続くMtbの播種を引き起こす可能性があることを示唆している。
Using two concomitant murine models of COVID-19 (SARS-CoV-2 infection of K18-hACE2 mice and mouse-adapted SARS-CoV-2 [MACoV2] infection of C57BL/6 mice) it was shown that chronically Mtb H37Rv-infected mice were resistant to the pathological consequences of secondary SARS-CoV-2 infection, and SARS-CoV-2 infection did not affect Mtb burdens.
COVID-19 の 2 つの同時マウスモデルの使用 (K18-hACE2 マウスの SARS-CoV-2 感染および C57BL/6 マウスのマウス適応 SARS-CoV-2 [MACoV2] 感染)すると、 慢性的にMtb H37Rvに感染したマウスは、SARS-CoV-2の二次感染による病理学的結果に耐性があり、SARS-CoV-2感染はMtb負荷に影響を及ぼさないことが示された。
Single-cell RNA sequencing of the lungs of the co-infected animals showed that resistance could be due to T and B cells expansion upon viral challenge.
同時感染した動物の肺の単細胞 RNA 配列決定により、耐性の原因がウイルス攻撃時の T 細胞および B 細胞の増殖による可能性があることが示されました。
Interestingly, lower lung protein levels of IFN-γ, IL-6 and IL-1β as well as mRNA levels of IFN-γ and TNF-α and higher levels of IL-10 were found in co-infection than in Mtb-monoinfection at the 30 days post-infection, similar to Hildebrand and colleagues.
興味深いことに、同時感染では感染後30日で、Mtb単独感染よりも肺タンパク質のIFN-γ、IL-6、IL-1βのレベルが低く、IFN-γとTNF-αのmRNAレベルが低く、IL-10のレベルが高いことが判明した。ヒルデブランドらと同様です。
Regarding the evaluation of the immune responses in co-infected humans, two studies have demonstrated that Mtb infection can modulate humoral (antibody) and cytokine responses to SARS-CoV-2 infection and vice versa in investigations conducted in TB endemic countries.
同時感染したヒトにおける免疫反応の評価に関しては、 結核流行国で行われた調査では、Mtb感染がSARS-CoV-2感染に対する体液性(抗体)反応とサイトカイン反応を調節する可能性があり、その逆も同様であることが2つの研究で実証されています。
Rajamanickam and colleagues demonstrated that individuals seropositive (IgG+) for SARS-CoV-2 infection and with TB infection (TBI+/SARS-CoV-2 IgG+) were characterized by higher levels of specific antibodies (IgM, IgG and IgA) and neutralizing antibodies against SARS-CoV-2 compared to individuals with only SARS-CoV-2 infection.
Rajamanickamらは次のことを実証しました。つまり、SARS-CoV-2 感染症および結核感染症(TBI+/SARS-CoV-2 IgG+)の血清陽性(IgG+)の個人は、SARS-CoV-2のみに感染している個人と比較して、SARS-CoV-2に対する特異的抗体(IgM、IgG、およびIgA)および中和抗体のレベルが高いことを特徴としていました。
Moreover, elevated plasma levels of proinflammatory cytokine/chemokine responses including IFN-γ, IL-2, TNF-α, IL-1α, IL-1β, IFN-α, IFN-β, IL-6, IL-12, IL-17, GM-CSF, CCL3, CXCL10 and anti-inflammatory cytokines such as IL-4, IL-10, IL-25 and IL-33 were found in TBI+/SARS-CoV-2 IgG+ subjects.
さらに、TBI+/SARS-CoV-2 IgG+ 被験者では、炎症誘発性サイトカイン/ケモカイン反応の血漿レベルの上昇が見られました。IFN-γ、IL-2、TNF-α、IL-1α、IL-1β、IFN-α、IFN-β、IL-6、IL-12、IL-17、GM-CSF、CCL3、CXCL10、IL-4、IL-10、IL-25、IL-33などの抗炎症性サイトカインを含みます。
These results show that Mtb infection can modulate the immune responses in asymptomatic SARS-CoV-2-infected individuals.
これらの結果は、Mtb 感染が無症候性の SARS-CoV-2 感染者の免疫応答を調節できることを示しています。
In an additional study, it was shown that TBI+/SARS-CoV-2 IgG+ individuals have higher baseline and Mtb-induced (but not mitogen) levels of several pro- and anti-inflammatory cytokines/chemokines including IFN-γ, IL-2, TNF-α, IL-17A, IL-1β, IL-6, IL-12, CCL1, CXCL1, CXCL9, CXCL10, IL-4, IL-13 and reduced levels of IL-5 and IL-10 compared to TBI-/SARS-CoV-2 IgG+ individuals.
追加の研究では、TBI+/SARS-CoV-2 IgG+ の人は、いくつかの炎症誘発性および抗炎症性サイトカイン/ケモカインのベースラインおよび Mtb 誘発性 (マイトジェンではない) レベルがTBI-/SARS-CoV-2 IgG+ の個人との比較して高いことが示されました。IFN-γ、IL-2、TNF-α、IL-17A、IL-1β、IL-6、IL-12、CCL1、CXCL1、CXCL9、CXCL10、IL-4、IL-13を含みます。IL-5とIL-10のレベルは低下しました。
These findings suggest modulating effects of SARS-CoV-2 infection on the immune responses of individuals with Mtb infection.
これらの発見は、Mtb 感染者の免疫応答に対する SARS-CoV-2 感染の調節効果を示唆しています。
However, these results were obtained in TB-infected individuals with only asymptomatic SARS-CoV-2 infection and the influence of each pathogen on the disease severity and the outcome of each infection were not evaluated.
ただし、これらの結果は、無症候性の SARS-CoV-2 感染のみを有する結核感染者で得られたものであり、各感染症の重症度および転帰に対する各病原体の影響は評価されていません。
Differently, clinical outcome was assessed in a case of multidrug-resistant (MDR)/TB-COVID-19 co-infected patient affected by bilateral cavitary pulmonary TB, that subsequently developed COVID-19-associated pneumonia which led to a fatal outcome.
別の方法では、多剤耐性(MDR)/結核とCOVID-19が重複感染し、両側肺空洞性結核に罹患し、その後COVID-19関連肺炎を発症して致死的な転帰に至った患者の症例で臨床転帰が評価された。
Death was probably due to the immuno-suppressed state of the patient, as shown by the low lymphocyte count and by the lack of response to Mtb antigens and mitogen.
リンパ球数の低下や、Mtb 抗原およびマイトジェンに対する反応の欠如によって示されるように、死亡はおそらく患者の免疫抑制状態によるものと考えられます。
In addition, a cohort of TB-COVID-19 co-infected patients with different severity of COVID-19 showed a reduced ability to mount a specific immune response to SARS-CoV-2 stimulation compared to patients with TBI and COVID-19 (TBI-COVID-19) or with COVID-19 only.
さらに、さまざまな重症度COVID-19のTB-COVID-19同時感染患者のコホートでは、TBI-COVID-19の患者、またはCOVID-19のみの患者と比較して、SARS-CoV-2刺激に対する特異的免疫反応を開始する能力の低下を示しました。
In particular, in TB-COVID-19 co-infected patients TNF-α, MIP-1β, and IL-9 showed significant elevated levels compared to COVID-19 only, and TNF-α had the highest discriminant power.
特に、結核とOVID-19の同時感染患者では、OVID-19のみと比較して、TNF-α、MIP-1β、IL-9のレベルが有意に上昇しており、TNF-αの判別力が最も高かった。
Moreover, TNF-α, IL-1β, IL-17A, IL-5, FGF-basic, and GM-CSF were increased in co-infected compared to patients with TB-only.
さらに、TNF-α、IL-1β、IL-17A、IL-5、FGF-basic、およびGM-CSFは、結核のみの患者と比較して、同時感染患者では増加していました。
Importantly, co-infection was associated with an impairment of SARS-CoV-2-specific and a reduced Mtb-specific immune response.
重要なのは、同時感染はSARS-CoV-2特異的免疫反応の障害とMtb特異的免疫反応の低下と関連していたということである。
In agreement with these results, Riou and colleagues demonstrated in TB-COVID-19 co-infection impaired SARS-CoV-2-specific and Mtb-specific CD4+ T cells with reduced polyfunctional cell potentials, proliferation cell capacity, and augmented cell activation markers.
これらの結果と一致して、Riouらは結核COVID-19の同時感染において、SARS-CoV-2特異的およびMtb特異的CD4+ T細胞が多機能細胞電位、増殖細胞能力の低下、および細胞活性化マーカーの増加を伴って障害されることを実証した。
However, the frequency of SARS-CoV-2 specific CD8+ T cell response to peptides spanning the M, N and S sequences in TB-COVID-19 co-infected patients was found to be comparable with patients with COVID-19 only.
しかし、TB-COVID-19 重複感染患者における M、N、S 配列にわたるペプチドに対する SARS-CoV-2 特異的 CD8+ T 細胞応答の頻度は、COVID-19 のみの患者と同等であることが判明しました。
Furthermore, several recent case studies have raised concerns regarding the Mtb reactivation in TB-infected subjects following SARS-CoV-2 co-infection.
さらに、最近のいくつかの事例研究では、SARS-CoV-2 の同時感染後の結核感染者における Mtb の再活性化に関する懸念が生じています。
These reports suggest that since the control of both Mtb and SARS-CoV-2 replication depends on cellular immunity, it is possible that the immune dysregulation caused by SARS-CoV-2 or the immunomodulatory therapies used for COVID-19 treatment may increase the risk for TB reactivation.
これらの報告は次のことを示唆しています。つまり、Mtb と SARS-CoV-2 の複製の制御は細胞性免疫に依存しているため、SARS-CoV-2 または COVID-19 治療に使用される免疫調節療法によって引き起こされる免疫調節不全が結核再活性化のリスクを高める可能性があります。
Both SARS-CoV-2 and Mtb have immunomodulating potentials to change the outcome of the course of each disease in co-infected patients: SARS-CoV-2 may cause immunosuppression and cytokine storm, which can contribute to the Mtb reactivation and lung tissue damage; Mtb may cause T-cell exhaustion and uncontrolled release of proinflammatory cytokines resulting in lung damage, thus potentially contributing to the susceptibility to SARS-CoV-2 infection and to a more severe COVID-19.
SARS-CoV-2 と Mtb は両方とも、重複感染した患者の各疾患の経過を変える免疫調節の可能性を持っています:SARS-CoV-2 は免疫抑制やサイトカインストームを引き起こす可能性があり、Mtb の再活性化や肺組織の損傷に寄与する可能性があります;Mtb は T 細胞の枯渇と炎症誘発性サイトカインの制御されない放出を引き起こし、肺損傷を引き起こす可能性があり、SARS-CoV-2 感染症やより重篤な COVID-19 への感受性に寄与する可能性があります。
In TB-infected individuals, T cells are responsible for Mtb control via the granuloma formation.
結核感染者では、T 細胞が肉芽腫形成を介して Mtb 制御を担っています。
Co-infection with SARS-CoV-2 in these individuals may negatively affect immune regulation in the granuloma leading to Mtb reactivation.
これらの個人における SARS-CoV-2 の同時感染は、肉芽腫の免疫調節に悪影響を及ぼし、Mtb の再活性化を引き起こす可能性があります。
This alteration of the immune system has been reported using a large-scale meta-analysis of transcriptomic data showing that some immune genes are enriched in COVID-19 and TB diseases.
この免疫系の変化は、一部の免疫遺伝子がCOVID-19 や結核疾患で豊富であることを示すトランスクリプトーム データの大規模メタ分析を使用して報告されています。
The findings from case reports indicate the presence of similarities in the immunopathogenesis of the two diseases, which may exacerbate disease severity during co-infection.
症例報告から得られた知見は、この 2 つの疾患の免疫病因に類似性が存在することを示しており、それが重感染時に疾患の重症度を悪化させる可能性があります。
Subclinical and clinical TB disease may increase the risk of severe COVID-19 disease and also SARS-CoV-2 co-infection may induce the progression to TB disease, as reported above.
上で報告されているように、無症候性および臨床的結核疾患は重篤な COVID-19 疾患のリスクを高める可能性があり、また SARS-CoV-2 の同時感染も結核疾患への進行を誘発する可能性があります。
In this regard, IFN-I which is strongly induced by viral infection may be detrimental in the context of Mtb by inhibiting B cell responses, inducing the release of immunosuppressive molecules or reducing the macrophagic activation induced by IFN-γ, Also, the hyperinflammatory milieu caused by Mtb may raise the risk of severe COVID-19 and vice versa.
この点に関して、ウイルス感染によって強く誘導される IFN-I は、B 細胞応答を阻害することにより、Mtb に関して有害である可能性があります。 免疫抑制分子の放出を誘導したり、IFN-γ によって誘導されるマクロファージの活性化を低下させたりします。また、Mtb によって引き起こされる過剰炎症環境は、重篤な COVID-19 のリスクを高める可能性があり、またその逆も同様です。
Mtb spread or reactivation might be favored by inflammatory molecules released from the SARS-CoV-2-induced necroptosis, whereas the apoptosis might mitigate it.
Mtb の拡散または再活性化は、SARS-CoV-2 誘発性ネクロトーシスから放出される炎症性分子によって促進される可能性がありますが、アポトーシスはそれを軽減する可能性があります。
Moreover, while COVID-19 therapies targeting pro-inflammatory cytokines may limit the acute immunopathology, they may also repress the responses needed to control Mtb containment.
さらに、炎症誘発性サイトカインを標的としたCOVID-19治療法は、急性免疫病理を制限する可能性がある一方で、Mtb封じ込めを制御するために必要な反応を抑制する可能性もあります。
Altogether, these studies suggest that co-infection alters the capacity of the host to respond to and control Mtb and/or SARS-CoV-2, indicating the need for further investigation of the underlying immunological pathways.
まとめると、これらの研究は、同時感染によって宿主のMtbおよび/またはSARS-CoV-2への応答および制御能力が変化することを示唆しており、根底にある免疫学的経路をさらに調査する必要があることを示しています。
Final remarks
SARS-CoV-2 and Mtb are currently the two deadliest infectious diseases in humans.
SARS-CoV-2 と Mtb は現在、人間にとって最も致死率の高い 2 つの感染症です。
While the route of infection and the target organ are similar, the time to disease manifestation and the pathways driving immunopathology differ significantly (Figure 3).
感染経路と標的臓器は類似していますが、疾患発現までの時間と免疫病理を引き起こす経路は大きく異なります (図 3)。
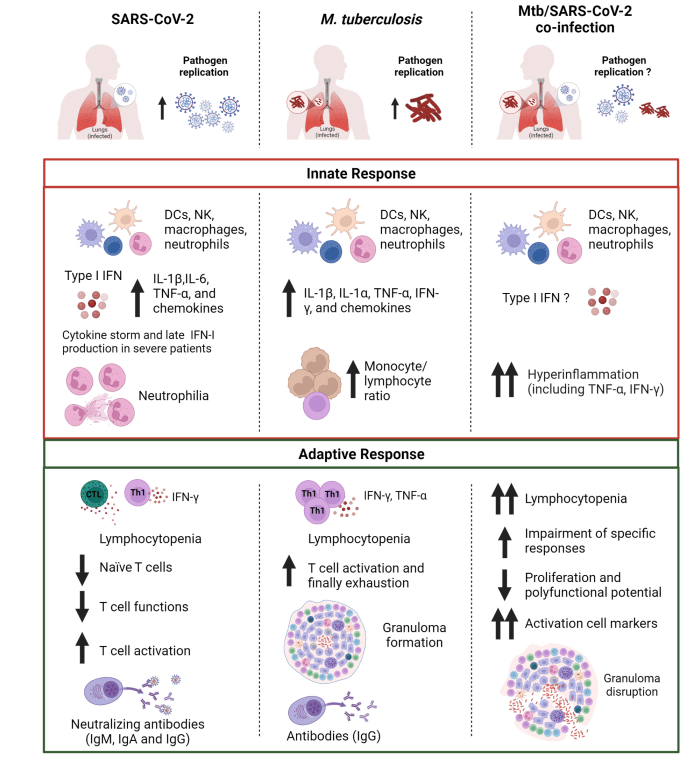
Evidence reported here show that both innate and adaptive immune response are critical components for the protection against SARS-CoV-2 and Mtb.
ここで報告された証拠は、自然免疫応答と適応免疫応答の両方が、SARS-CoV-2 および Mtb に対する防御にとって重要な要素であることを示しています。
The immune response to both SARS-CoV-2 and Mtb is complex and multifaceted, and there are still many aspects that are not well understood.
SARS-CoV-2 と Mtb の両方に対する免疫応答は複雑かつ多面的であり、まだ十分に理解されていない側面が多くあります。
However, it is known that an appropriate activation of the innate immunity in the early stages of infection followed by adaptive immunity is necessary to curb the pathogen dissemination in the host.
しかし、宿主内での病原体の蔓延を抑制するには、感染の初期段階での自然免疫の適切な活性化とその後の適応免疫の活性化が必要であることが知られている。
The comparison of these two pathogens highlights how the innate immune response induced after exposure to SARS-CoV-2 or Mtb share the production of some pro-inflammatory cytokines including IL-1β and TNF-α.
これら 2 つの病原体の比較は、SARS-CoV-2 または Mtb への曝露後に誘発される自然免疫応答が、IL-1β や TNF-α などのいくつかの炎症促進性サイトカインの産生をどのように共有しているかを浮き彫りにします。
Similar results were found in Mtb/SARS-CoV-2 co-infection.
Mtb/SARS-CoV-2 同時感染でも同様の結果が見られました。
For SARS-CoV-2 infection, the early and robust IFN-I production as well as neutralizing antibodies have an outmost importance for guarantee an efficient control of viral spread and to determine the clinical outcome of COVID-19.
SARS-CoV-2 感染症の場合、早期かつ強力な IFN-I 産生と中和抗体が、ウイルス拡散の効率的な制御を保証し、COVID-19 の臨床転帰を決定するために最も重要です。
On the other hand, in Mtb infection a central role is played by the alveolar macrophages and the cytokines they release as TNF-α and IL-1β.
一方、Mtb 感染では、肺胞マクロファージと、それらが TNF-α および IL-1β として放出するサイトカインが中心的な役割を果たします。
Although the infections caused by the individual pathogens have been intensively studied, there are still many unanswered questions about the influence of these pathogens on each other, the immune response, and clinical outcome in the context of co-infection.
個々の病原体によって引き起こされる感染症は集中的に研究されていますが、これらの病原体相互の影響、免疫応答、および同時感染の状況における臨床転帰については、まだ多くの未解決の疑問が残っています。
Recent data has raised concerns regarding the Mtb reactivation following SARS-CoV-2 infection likely due to immune dysregulation caused by SARS-CoV-2 or immunomodulatory COVID-19 therapies.
最近のデータにより、SARS-CoV-2 感染後の Mtb 再活性化は、おそらく SARS-CoV-2 または免疫調節性の COVID-19 療法によって引き起こされる免疫調節不全が原因であるとの懸念が生じています。
Further clinical and scientific research is needed to better understand the interaction and outcome of the co-infection.
同時感染の相互作用と結果をより深く理解するには、さらなる臨床的および科学的研究が必要です。
以下省略。
この記事が気に入ったらサポートをしてみませんか?