SARS-CoV-2 と SARS-CoV の相対的親和性、複製速度、細胞損傷プロファイリングと COVID-19 の臨床症状、伝染性、実験室研究への影響: 観察研究
Comparative tropism, replication kinetics, and cell damage profiling of SARS-CoV-2 and SARS-CoV with implications for clinical manifestations, transmissibility, and laboratory studies of COVID-19: an observational study
SARS-CoV-2 と SARS-CoV の相対的親和性、複製速度、細胞損傷プロファイリングと COVID-19 の臨床症状、伝染性、実験室研究への影響: 観察研究
Summary
Background
Severe acute respiratory syndrome coronavirus 2 (SARS-CoV-2) was reported from China in January, 2020.
重症急性呼吸器症候群コロナウイルス2(SARS-CoV-2)は、2020年1月に中国から報告されました。
SARS-CoV-2 is efficiently transmitted from person to person and, in 2 months, has caused more than 82 000 laboratory-confirmed cases of coronavirus disease 2019 (COVID-19) and 2800 deaths in 46 countries.
SARS-CoV-2は人から人へと効率的に伝染し、2か月間で46か国で82,000件を超えるコロナウイルス感染症2019(COVID-19)の検査確定例と2,800人の死亡を引き起こしました。
The total number of cases and deaths has surpassed that of the 2003 severe acute respiratory syndrome coronavirus (SARS-CoV).
症例数と死亡者数は、2003年の重症急性呼吸器症候群コロナウイルス(SARS-CoV)の症例数を上回りました。
Although both COVID-19 and severe acute respiratory syndrome (SARS) manifest as pneumonia, COVID-19 is associated with apparently more efficient transmission, fewer cases of diarrhoea, increased mental confusion, and a lower crude fatality rate.
COVID-19と重症急性呼吸器症候群(SARS)はどちらも肺炎として現れますが、COVID-19は明らかにより効率的な伝染、下痢の症例の減少、精神混乱の増加、粗死亡率の低下と関連しています。
However, the underlying virus–host interactive characteristics conferring these observations on transmissibility and clinical manifestations of COVID-19 remain unknown.
しかし、COVID-19の伝染性と臨床症状に関するこれらの観察結果をもたらす根本的なウイルスと宿主の相互作用特性は不明のままです。
Methods
We systematically investigated the cellular susceptibility, species tropism, replication kinetics, and cell damage of SARS-CoV-2 and compared findings with those for SARS-CoV.
SARS-CoV-2 の細胞感受性、種指向性、複製速度、細胞損傷を体系的に調査し、その結果を SARS-CoV の結果と比較しました。
We compared SARS-CoV-2 and SARS-CoV replication in different cell lines with one-way ANOVA.
異なる細胞株における SARS-CoV-2 と SARS-CoV の複製を一元配置分散分析で比較しました。
For the area under the curve comparison between SARS-CoV-2 and SARS-CoV replication in Calu3 (pulmonary) and Caco2 (intestinal) cells, we used Student's t test.
Calu3 (肺) 細胞と Caco2 (腸) 細胞における SARS-CoV-2 と SARS-CoV の複製の曲線下面積の比較には、スチューデント t 検定を使用しました。
We analysed cell damage induced by SARS-CoV-2 and SARS-CoV with one-way ANOVA.
SARS-CoV-2 と SARS-CoV によって引き起こされる細胞損傷を一元配置分散分析で分析しました。
Findings
SARS-CoV-2 infected and replicated to comparable levels in human Caco2 cells and Calu3 cells over a period of 120 h (p=0·52).
SARS-CoV-2 は、120 時間にわたってヒト Caco2 細胞と Calu3 細胞に感染し、同程度に複製しました (p=0.52)。
By contrast, SARS-CoV infected and replicated more efficiently in Caco2 cells than in Calu3 cells under the same multiplicity of infection (p=0·0098).
対照的に、SARS-CoV は、同じ感染多重度では、Caco2 細胞の方が Calu3 細胞よりも効率的に感染し、複製しました (p=0.0098)。
SARS-CoV-2, but not SARS-CoV, replicated modestly in U251 (neuronal) cells (p=0·036).
SARS-CoV-2 は U251 (神経細胞) 細胞で適度に複製しましたが、SARS-CoV は複製しませんでした (p=0.036)。
For animal species cell tropism, both SARS-CoV and SARS-CoV-2 replicated in non-human primate, cat, rabbit, and pig cells.
動物種の細胞指向性については、SARS-CoV と SARS-CoV-2 はどちらも、ヒト以外の霊長類、ネコ、ウサギ、ブタの細胞で複製しました。
SARS-CoV, but not SARS-CoV-2, infected and replicated in Rhinolophus sinicus bat kidney cells.
SARS-CoV は Rhinolophus sinicus コウモリの腎臓細胞に感染し、複製しましたが、SARS-CoV-2 は複製しませんでした。
SARS-CoV-2 consistently induced significantly delayed and milder levels of cell damage than did SARS-CoV in non-human primate cells (VeroE6, p=0·016; FRhK4, p=0·0004).
SARS-CoV-2は、非ヒト霊長類細胞において、一貫してSARS-CoVよりも有意に遅れて、より軽度の細胞損傷を引き起こした(VeroE6、p = 0.016; FRhK4、p = 0.0004)。
Interpretation
As far as we know, our study presents the first quantitative data for tropism, replication kinetics, and cell damage of SARS-CoV-2.
私たちの知る限り、本研究は、SARS-CoV-2 の向性、複製速度、細胞損傷に関する初の定量的データを提示しています。
These data provide novel insights into the lower incidence of diarrhoea, decreased disease severity, and reduced mortality in patients with COVID-19, with respect to the pathogenesis and high transmissibility of SARS-CoV-2 compared with SARS-CoV.
これらのデータは、SARS-CoV と比較した SARS-CoV-2 の病因と高い伝染性に関して、COVID-19 患者の下痢の発生率の低さ、疾患の重症度の軽減、死亡率の低下に関する新たな洞察を提供します。
Funding
May Tam Mak Mei Yin, The Shaw Foundation Hong Kong, Richard Yu and Carol Yu, Michael Seak-Kan Tong, Respiratory Viral Research Foundation, Hui Ming, Hui Hoy and Chow Sin Lan Charity Fund, Chan Yin Chuen Memorial Charitable Foundation, Marina Man-Wai Lee, The Hong Kong Hainan Commercial Association South China Microbiology Research Fund, The Jessie & George Ho Charitable Foundation, Perfect Shape Medical, The Consultancy Service for Enhancing Laboratory Surveillance of Emerging Infectious Diseases and Research Capability on Antimicrobial Resistance for the Department of Health of the Hong Kong Special Administrative Region Government, The Theme-Based Research Scheme of the Research Grants Council, Sanming Project of Medicine in Shenzhen, and The High Level-Hospital Program, Health Commission of Guangdong Province, China.
Introduction
Coronaviruses are enveloped, positive-sense, single-stranded RNA viruses that can infect a wide range of human and animal species.
コロナウイルスは、エンベロープを持つプラス鎖一本鎖RNAウイルスで、幅広い種類のヒトや動物に感染する可能性があります。
Before December, 2019, six human pathogenic coronaviruses were known.
2019年12月以前には、6種類のヒト病原性コロナウイルスが知られていました。
Severe acute respiratory syndrome coronavirus (SARS-CoV) and Middle East respiratory syndrome coronavirus (MERS-CoV) can cause severe acute atypical pneumonia with extrapulmonary manifestations in both immunocompetent and immunocompromised patients.
重症急性呼吸器症候群コロナウイルス(SARS-CoV)と中東呼吸器症候群コロナウイルス(MERS-CoV)は、免疫能のある患者と免疫不全の患者の両方で、肺外症状を伴う重篤な急性非定型肺炎を引き起こす可能性があります。
Human coronavirus 229E (HCoV-229E), HCoV-NL63, HCoV-OC43, and HCoV-HKU1 usually cause mild and self-limiting upper respiratory tract infections in immunocompetent patients and, occasionally, lower respiratory tract infections in immunocompromised hosts.
ヒトコロナウイルス229E(HCoV-229E)、HCoV-NL63、HCoV-OC43、およびHCoV-HKU1は、通常、免疫能のある患者では軽度で自然に治まる上気道感染症を引き起こし、免疫不全の宿主では時折、下気道感染症を引き起こします。
On Dec 31, 2019, WHO was informed of a cluster of unexplained cases of pneumonia in Wuhan, Hubei province, China.
2019年12月31日、WHOは中国湖北省武漢市で原因不明の肺炎の症例が集団発生したことを知らされた。
Subsequent investigations identified a novel lineage B betacoronavirus (later named severe acute respiratory syndrome coronavirus 2 [SARS-CoV-2]) with a high degree of genomic similarity with bat coronaviruses.その後の調査で、コウモリコロナウイルスと高いゲノム類似性を持つ新しい系統Bベータコロナウイルス(後に重症急性呼吸器症候群コロナウイルス2 [SARS-CoV-2] と命名)が特定された。
The typical clinical features of SARS-CoV-2 infection, or coronavirus disease 2019 (COVID-19), are similar to severe acute respiratory syndrome (SARS) and include fever, myalgia, dry cough, dyspnoea, fatigue, and radiological evidence of ground-glass lung opacities compatible with atypical pneumonia.
SARS-CoV-2感染症、またはコロナウイルス疾患2019(COVID-19)の典型的な臨床的特徴は重症急性呼吸器症候群(SARS)に似ており、発熱、筋肉痛、乾いた咳、呼吸困難、疲労、非定型肺炎と一致するすりガラス状肺陰影の放射線学的証拠などがある。
However, important clinical differences between COVID-19 and SARS have been increasingly recognised.
しかし、COVID-19とSARSの重要な臨床的違いがますます認識されつつある。
For example, diarrhoea is seen much less frequently in patients with COVID-19 (2·0–10·1%) than in those with SARS (20·1%).
たとえば、COVID-19 患者では下痢が見られる頻度は SARS 患者 (20.1%) に比べてはるかに低い (2.0~10.1%)。
Neurological manifestations such as confusion are infrequently reported in patients with COVID-19 (9·1%) but are almost absent in patients with SARS.
混乱などの神経学的症状は COVID-19 患者ではまれにしか報告されていない (9.1%) が、SARS 患者ではほとんど見られない。
Another apparent difference between SARS-CoV-2 and SARS-CoV is the speed at which the COVID-19 outbreak is expanding.
SARS-CoV-2 と SARS-CoV のもう 1 つの明らかな違いは、COVID-19 の流行が拡大する速度である。
Within just 2 months, the number of laboratory-confirmed COVID-19 cases worldwide has already exceeded the total number of SARS cases by nearly ten times.
わずか 2 か月以内に、世界中で検査で確認された COVID-19 の症例数は、すでに SARS の症例総数の 10 倍近くになっている。
The mean basic reproduction number (R0) of COVID-19 has been estimated to be similar to that of SARS (roughly 3·0).
COVID-19 の平均基本再生産数 (R0) は、SARS のそれと同程度 (およそ 3.0) と推定されている。
However, in the epicentre of outbreak in Hubei province, the R0 of COVID-19 could be as high as 6·49.
しかし、湖北省の発生中心地では、COVID-19のR0は6.49にまで高くなる可能性があります。
Although the COVID-19 pandemic might be related to various geographical and social factors, such as the high population density in Wuhan, the massive population movement before the Spring Festival, and control measures implemented by health authorities during the different phases of the outbreak, it remains unclear whether SARS-CoV-2 possesses viral factors that are associated with higher transmissibility than SARS-CoV.
COVID-19パンデミックは、武漢の人口密度の高さ、春節前の大規模な人口移動、発生のさまざまな段階で保健当局が実施した管理措置など、さまざまな地理的および社会的要因に関連している可能性がありますが、SARS-CoV-2がSARS-CoVよりも高い伝染性に関連するウイルス因子を持っているかどうかは不明です。
As shown by SARS-CoV, MERS-CoV, and other emerging viruses, cell culture models are useful for characterising cell tropism, viral replication kinetics, and virus-induced cell damage profiles of novel viruses.
SARS-CoV、MERS-CoV、その他の新興ウイルスで示されているように、細胞培養モデルは、新規ウイルスの細胞向性、ウイルス複製速度、およびウイルス誘発性細胞損傷プロファイルを特徴付けるのに役立ちます。
To date, these important biological characteristics of infectious SARS-CoV-2 in tissue cell cultures remain incompletely understood.
現在まで、組織細胞培養における感染性SARS-CoV-2のこれらの重要な生物学的特性は、完全には理解されていません。
In this study, we analysed the differential susceptibilities of 25 cell lines derived from different human organ tissues and non-human animal species and compared findings in SARS-CoV-2 and SARS-CoV.
この研究では、さまざまなヒト臓器組織およびヒト以外の動物種に由来する25の細胞株の異なる感受性を分析し、SARS-CoV-2とSARS-CoVの所見を比較しました。
Our findings could provide potential explanations at tissue culture level for noted differences in clinical manifestations and transmission characteristics between SARS-CoV-2 and SARS-CoV, as well as information for the rational design of diagnostics and research methods for COVID-19.
私たちの所見は、SARS-CoV-2とSARS-CoVの臨床症状と感染特性の顕著な違いについて組織培養レベルでの潜在的な説明を提供し、COVID-19の診断と研究方法の合理的な設計に関する情報も提供します。
Methods
Viruses and biosafety
We isolated SARS-CoV-2 HKU-001a from a nasopharyngeal aspirate specimen taken from a patient from Hong Kong with laboratory-confirmed COVID-19.
香港のCOVID-19感染が確認された患者から採取した鼻咽頭吸引検体からSARS-CoV-2 HKU-001aを分離しました。
We inoculated the nasopharyngeal aspirate specimen on VeroE6 (non-human primate kidney) cells, with and without 0·5 μg/mL trypsin.
鼻咽頭吸引検体を、0.5μg/mLトリプシンの有無にかかわらず、VeroE6(非ヒト霊長類腎臓)細胞に接種しました。
We monitored the inoculated cells daily for cytopathic effects by light microscopy, and we collected cell supernatant daily for quantitative RT-PCR to assess the viral load.
接種した細胞を光学顕微鏡で毎日観察し、細胞上清を毎日採取して定量RT-PCRを行い、ウイルス量を評価しました。
Substantial cytopathic effects were seen at 72 h postinoculation (hpi), and we confirmed positive SARS-CoV-2 replication by quantitative RT-PCR using specific primers and probes against SARS-CoV-2 (panel).
接種後72時間(hpi)にかなりの細胞変性効果が見られ、SARS-CoV-2に対する特異的プライマーとプローブを使用した定量RT-PCRにより、SARS-CoV-2の複製が陽性であることを確認しました(パネル)。
We did whole-genome sequencing of the SARS-CoV-2 isolate using an Oxford Nanopore MinION device (Oxford Nanopore Technologies, Oxford, UK) supplemented by Sanger sequencing, as previously described.
我々は、前述のように、オックスフォードナノポアMinIONデバイス(オックスフォードナノポアテクノロジーズ、英国オックスフォード)を使用して、サンガーシーケンシングを補完し、SARS-CoV-2分離株の全ゲノムシーケンシングを行った。
Sequence comparisons between SARS-CoV-2 HKU-001a and clinical isolates are shown in the appendix (pp 1–2).
SARS-CoV-2 HKU-001a と臨床分離株の配列比較は付録 (1~2 ページ) に示されています。
SARS-CoV was a clinical isolate archived at the Department of Microbiology, The University of Hong Kong.
SARS-CoV は香港大学微生物学部に保管されている臨床分離株です。
Both SARS-CoV-2 HKU-001a (GenBank accession number MT230904) and SARS-CoV GZ50 (AY304495) were propagated and the titre ascertained in VeroE6 cells with plaque assays.
SARS-CoV-2 HKU-001a (GenBank 登録番号 MT230904) と SARS-CoV GZ50 (AY304495) の両方が増殖され、プラークアッセイで VeroE6 細胞で力価が確認されました。
Both viruses were passaged three times before being used for experiments.
両方のウイルスは実験に使用する前に 3 回継代されました。
All experiments entailing live SARS-CoV-2 and SARS-CoV followed the approved standard operating procedures of our biosafety level 3 facility.
生きた SARS-CoV-2 と SARS-CoV を含むすべての実験は、バイオセーフティ レベル 3 施設の承認された標準操作手順に従いました。
Phylogenetic analysis of SARS-CoV-2 and SARS-CoV
Three nucleotide sequences of SARS-CoV-2 (GenBank accession numbers MN938384, MN975262, and MT230904) and two nucleotide sequences of SARS-CoV (AY278491 and AY304495) were first aligned using MUSCLE.
まず、SARS-CoV-2 の 3 つのヌクレオチド配列 (GenBank アクセッション番号 MN938384、MN975262、MT230904) と SARS-CoV の 2 つのヌクレオチド配列 (AY278491 と AY304495) を MUSCLE を使用してアラインメントしました。
We used aligned sequences for the determination of best model and for the phylogenetic analysis using MEGA X.
アラインメントした配列は、最適なモデルの決定と MEGA X を使用した系統解析に使用しました。
Cell culture and virus infection
We inoculated 25 cell lines derived from different tissues or organs and host species (table) with SARS-CoV-2 or SARS-CoV at a multiplicity of infection (MOI) of 0·1 for 2 h at 37°C.
我々は、異なる組織または臓器および宿主種(表)から得られた 25 の細胞株に、感染多重度(MOI)0.1 で SARS-CoV-2 または SARS-CoV を 37°C で 2 時間接種しました。
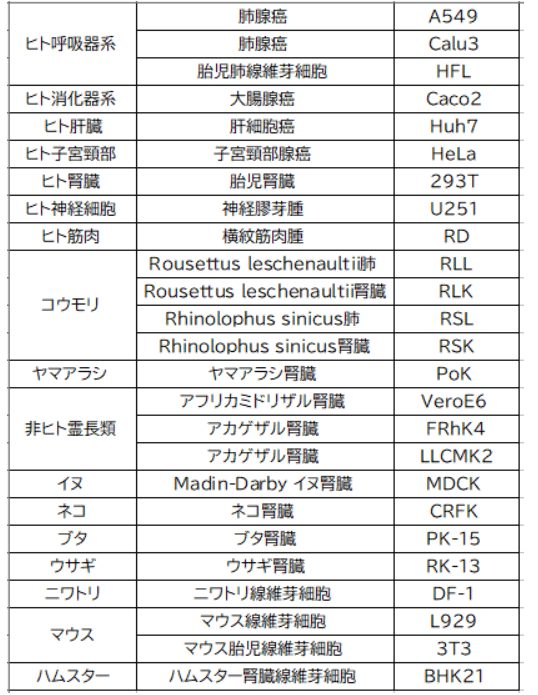
Depending on the cell type, we maintained cells in minimum essential medium (Gibco, Waltham, MA, USA), Dulbecco's modified Eagle's medium (DMEM; Gibco), or DMEM/F12 (Gibco), according to suppliers' instructions.
細胞の種類に応じて、最小必須培地(Gibco、米国マサチューセッツ州ウォルサム)、ダルベッコ改変イーグル培地(DMEM、Gibco)、またはDMEM/F12(Gibco)で細胞を維持した。 サプライヤーの指示に従っています。
The cell lines we used are routinely tested for mycoplasma and are maintained mycoplasma-free.
使用した細胞株は、定期的にマイコプラズマ検査を受けており、マイコプラズマフリーで維持されている。
RNA extraction and quantitative RT-PCR
We harvested supernatant samples from infected cells at 2 hpi, 24 hpi, 72 hpi, and 120 hpi for quantitative RT-PCR analysis of virus replication, as previously described.
前述のとおり、感染細胞から 2 hpi、24 hpi、72 hpi、120 hpi の上清サンプルを採取し、ウイルス複製の定量的 RT-PCR 分析を行いました。
Briefly, we lysed 100 μL of viral supernatant then extracted total RNA with QIAamp viral RNA mini kit (Qiagen, Hilden, Germany).
簡単に説明すると、ウイルス上清 100 μL を溶解し、QIAamp ウイルス RNA ミニ キット (Qiagen、ドイツ、ヒルデン) で全 RNA を抽出しました。
We used real-time quantitative RT-PCR to quantify SARS-CoV-2 and SARS-CoV replication, using QuantiNova Probe RT-PCR kit (Qiagen) with a LightCycler 480 Real-Time PCR System (Roche, Basel, Switzerland).
SARS-CoV-2 および SARS-CoV の複製を定量化するために、QuantiNova Probe RT-PCR キット (Qiagen) と LightCycler 480 リアルタイム PCR システム (Roche、スイス、バーゼル) を使用してリアルタイム定量 RT-PCR を行いました。
Each 20 μL reaction mixture contained 10 μL of 2 × QuantiNova Probe RT-PCR Master Mix (Qiagen), 1·2 μL of RNase-free water, 0·2 μL of QuantiNova Probe RT-Mix (Qiagen), 1·6 μL each of 10 μmol/L forward and reverse primer, 0·4 μL of 10 μmol/L probe, and 5 μL of extracted RNA as template.
各 20 μL の反応混合物には、2 × QuantiNova Probe RT-PCR マスター ミックス (Qiagen) 10 μL、RNase フリー水 1.2 μL、QuantiNova Probe RT-Mix (Qiagen) 0.2 μL、10 μmol/L フォワード プライマーとリバース プライマー各 1.6 μL、10 μmol/L プローブ 0.4 μL、およびテンプレートとして抽出した RNA 5 μL が含まれていました。
We incubated the reactions at 45°C for 10 min for reverse transcription, 95°C for 5 min for denaturation, followed by 45 cycles of 95°C for 5 s and 55°C for 30 s.
反応物を 45°C で 10 分間インキュベートして逆転写し、95°C で 5 分間インキュベートして変性させ、その後 95°C で 5 秒間、55°C で 30 秒間を 45 サイクル繰り返しました。
We detected and measured the signal in each cycle after the annealing step.
アニーリング ステップの後、各サイクルでシグナルを検出して測定しました。
The cycling profile ended with a cooling step at 40°C for 30 s.
サイクリング プロファイルは、40°C で 30 秒間の冷却ステップで終了しました。
Primers and probe sequences were against the RNA-dependent RNA polymerase and helicase gene region of SARS-CoV-2 and SARS-CoV (panel).
プライマーとプローブ配列は、SARS-CoV-2 および SARS-CoV の RNA 依存性 RNA ポリメラーゼおよびヘリカーゼ遺伝子領域に対するものでした (パネル)。
Generation and titration of immune serum
To prepare antibodies against the nucleocapsid protein (NP) of SARS-CoV-2, we mixed 100 μg of purified SARS-CoV-2-NP recombinant protein with an equal volume of complete Freund's adjuvant (Sigma-Aldrich, St Louis, MO, USA) and injected the mixture subcutaneously into 4–6-week-old New Zealand white rabbits.
SARS-CoV-2 のヌクレオカプシドタンパク質 (NP) に対する抗体を調製するために、精製された SARS-CoV-2-NP 組み換えタンパク質 100 μg を等量の完全フロイントアジュバント (Sigma-Aldrich、米国ミズーリ州セントルイス) と混合し、その混合物を 4~6 週齢のニュージーランド白ウサギの皮下注射しました。
We used incomplete Freund's adjuvant (Sigma-Aldrich) for three subsequent injections at 14-day intervals. We obtained serum samples after the third injection.
その後 14 日間隔で 3 回の注射に不完全フロイントアジュバント (Sigma-Aldrich) を使用しました。3 回目の注射後に血清サンプルを採取しました。
For titration of the immune serum, we coated 96-well immunoplates (Nunc Immuno modules, Nunc, Denmark) with 100 μL per well (0·1 μg per well) of SARS-CoV-2-NP in 0·05 mol/L NaHCO3 (pH 9·6) overnight at 4°C, followed by incubation with a blocking reagent.
免疫血清の滴定のために、96 ウェル免疫プレート (Nunc Immuno モジュール、Nunc、デンマーク) を、0.05 mol/L NaHCO3 (pH 9.6) 中の SARS-CoV-2-NP をウェルあたり 100 μL (ウェルあたり 0.1 μg) でコーティングし、4°C で一晩放置した後、ブロッキング試薬でインキュベートしました。
After blocking, we added to the wells 100 μL serial-diluted immunised rabbit SARS-CoV-2-NP or SARS-CoV-NP serum (starting from a dilution of 1/1000) and incubated the plates at 37°C for 1 h.
ブロッキング後、ウェルに段階希釈した免疫ウサギ SARS-CoV-2-NP または SARS-CoV-NP 血清 (1/1000 希釈から開始) を 100 μL 加え、プレートを 37°C で 1 時間インキュベートしました。
After the plates were washed, we added horseradish peroxidase-labelled goat anti-rabbit antibody (Invitrogen, Carlsbad, CA, USA) at 100 μL per well, and we incubated the plates for 30 mins at 37°C.
プレートを洗浄した後、ホースラディッシュペルオキシダーゼ標識ヤギ抗ウサギ抗体(Invitrogen、米国カリフォルニア州カールスバッド)をウェルあたり100μL加え、プレートを37°Cで30分間インキュベートしました。
After incubation, we washed the wells then added 3,3',5,5'-tetramethylbenzidine solution (Invitrogen).
インキュベーション後、ウェルを洗浄し、3,3',5,5'-テトラメチルベンジジン溶液(Invitrogen)を加えました。
After 10 min of reaction time, we stopped the reactions in the wells with 0·3 N sulphuric acid.
10分間の反応時間後、0.3N硫酸でウェル内の反応を停止しました。
We read the optical density at 450 nm with a plate reader (Perkin Elmer, Waltham, MA, USA).
プレートリーダー(Perkin Elmer、米国マサチューセッツ州ウォルサム)で450nmの光学密度を読み取りました。
Immunostaining and confocal microscopy
To detect antigen expression in SARS-CoV-2-infected cells, we used an in-house rabbit antiserum against SARS-CoV-2-NP.
SARS-CoV-2 に感染した細胞における抗原発現を検出するために、SARS-CoV-2-NP に対する社内ウサギ抗血清を使用しました。
We labelled cell nuclei with the DAPI nucleic acid stain (ThermoFisher Scientific, Waltham, MA, USA).
細胞核を DAPI 核酸染色 (ThermoFisher Scientific、米国マサチューセッツ州ウォルサム) で標識しました。
The secondary antibody was Alexa Fluor (ThermoFisher Scientific). Mounting was done with the Diamond Prolong Antifade mountant (ThermoFisher Scientific).
二次抗体は Alexa Fluor (ThermoFisher Scientific) でした。マウントは Diamond Prolong Antifade マウント剤 (ThermoFisher Scientific) で行いました。
We acquired images with confocal microscopy using the Carl Zeiss LSM780 system (Zeiss, Oberkochen, Germany) with the 40 × oil immersion objective, as previously described.
前述のように、Carl Zeiss LSM780 システム (Zeiss、ドイツオーバーコッヘン) と 40 × 油浸対物レンズを使用して共焦点顕微鏡で画像を取得しました。
Cell viability assays and imaging of cytopathic effect
To ascertain cell damage on SARS-CoV-2 and SARS-CoV infection, we quantified cell viability with the CellTiterGlo assay (Promega, Madison, WI, USA).
SARS-CoV-2 および SARS-CoV 感染による細胞損傷を確認するために、CellTiterGlo アッセイ (Promega、米国ウィスコンシン州マディソン) で細胞生存率を定量化しました。
We lysed cells together with culture supernatant at a ratio of 1:1 (volume) with the CellTiter-Glo reagent and incubated this mixture at room temperature for 10 min, then we measured the luminescence signal with the Vector X3 multilabel plate reader (Perkin Elmer).
細胞を培養上清とともに CellTiter-Glo 試薬で 1:1 (容量) の割合で溶解し、この混合物を室温で 10 分間インキュベートした後、Vector X3 マルチラベル プレート リーダー (Perkin Elmer) で発光信号を測定しました。
We obtained images of cellular cytopathic effect on SARS-CoV-2 and SARS-CoV infection at 72 hpi with a Nikon Ts2R-FL inverted microscope (Nikon, Tokyo, Japan).
Nikon Ts2R-FL 倒立顕微鏡 (Nikon、東京、日本) を使用して、感染後 72 hpi で SARS-CoV-2 および SARS-CoV 感染に対する細胞変性効果の画像を取得しました。
Recombinant human ACE2 blocking assay
We preincubated SARS-CoV-2 or SARS-CoV with 80 μg/mL recombinant human angiotensin-converting enzyme (ACE) 2 (R&D Systems, Minneapolis, MN, USA) for 1 h.
SARS-CoV-2 または SARS-CoV を 80 μg/mL の組み換えヒトアンジオテンシン変換酵素 (ACE) 2 (R&D Systems、米国ミネソタ州ミネアポリス) とともに 1 時間プレインキュベートしました。
We added the mixture to VeroE6 cells for 30 min. Subsequently, we washed cells and incubated them with fresh medium for 4 h before cell lysates were harvested for quantitative RT-PCR analysis of virus genome copies.
混合物を VeroE6 細胞に 30 分間加えました。その後、細胞を洗浄し、新鮮な培地で 4 時間インキュベートした後、細胞溶解物を採取してウイルスゲノムコピーの定量的 RT-PCR 分析を行いました。
Statistical analysis
We compared SARS-CoV-2 or SARS-CoV replication in different cell lines with one-way ANOVA.
異なる細胞株における SARS-CoV-2 または SARS-CoV の複製を一元配置分散分析で比較しました。
For the area under the curve (AUC) comparison between SARS-CoV-2 or SARS-CoV replication in Calu3 (pulmonary) and Caco2 (intestinal) cells, we used Student's t test.
Calu3 (肺) 細胞と Caco2 (腸) 細胞における SARS-CoV-2 または SARS-CoV の複製の曲線下面積 (AUC) の比較には、スチューデント t 検定を使用しました。
We analysed cell damage induced by SARS-CoV-2 or SARS-CoV with one-way ANOVA.
SARS-CoV-2 または SARS-CoV によって誘発される細胞損傷を一元配置分散分析で分析しました。
All analyses were done with GraphPad Prism version 6 (GraphPad, San Diego, CA, USA). We judged differences statistically significant when p values were less than 0·05.
すべての分析は、GraphPad Prism バージョン 6 (GraphPad、米国カリフォルニア州サンディエゴ) を使用して行いました。 p 値が 0.05 未満の場合、統計的に有意な差があると判断しました。
Role of the funding source
The funders had no role in study design, data collection, data analysis, data interpretation, or writing of the report. The corresponding author had full access to all data in the study and had final responsibility for the decision to submit for publication.
資金提供者は、研究デザイン、データ収集、データ分析、データ解釈、レポートの執筆には一切関与していません。責任著者は研究のすべてのデータに完全にアクセスでき、出版の決定に対する最終責任を負っています。
Results
Of nine human cell lines tested (table), five were susceptible to SARS-CoV-2 infection, as shown by significant virus replication over a period of 120 h (figure 1A).
検査した 9 つのヒト細胞株 (表) のうち、5 つは SARS-CoV-2 感染の影響を受けやすく、120 時間にわたってウイルスが顕著に複製されたことが示されています (図 1A)。
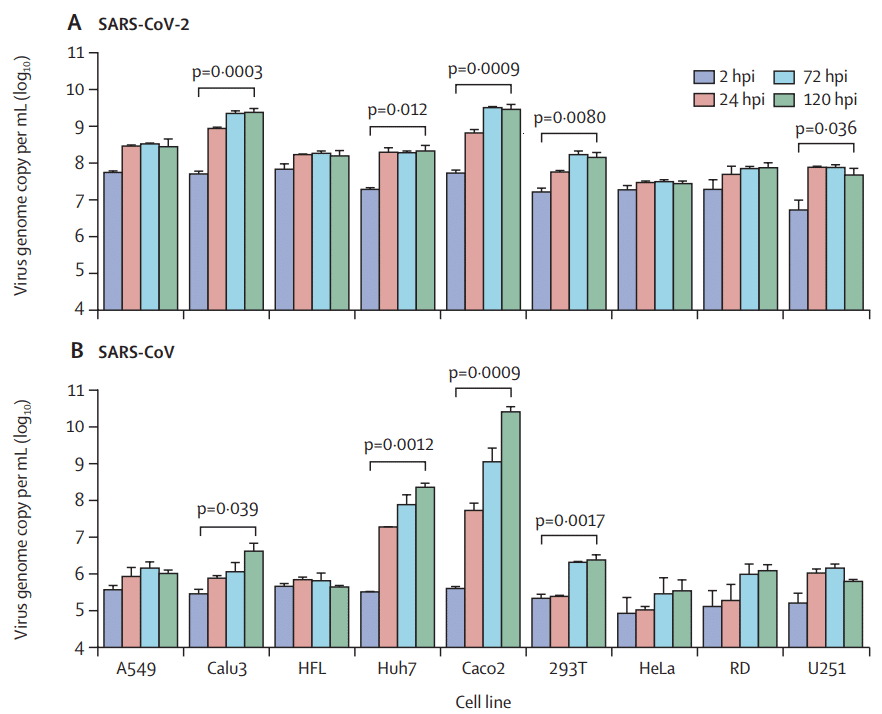
Among these susceptible cell lines, SARS-CoV-2 replication was most robust in Calu3 (pulmonary; p=0·0003) and Caco2 (intestinal; p=0·0009) cells, followed by Huh7 (hepatic; p=0·012) and 293T (renal; p=0·0080) cells.
これらの感受性細胞株のうち、SARS-CoV-2の複製が最も強かったのはCalu3(肺、p=0.0003)細胞とCaco2(腸、p=0.0009)細胞で、次いでHuh7(肝臓、p=0.012)細胞と293T(腎臓、p=0.0080)細胞でした。
Furthermore, modest SARS-CoV-2 replication was detected in U251 (neuronal; p=0·036) cells.
さらに、U251(神経細胞、p=0.036)細胞では、中程度のSARS-CoV-2複製が検出されました。
In general, the cellular tropism of SARS-CoV-2 was similar to that of SARS-CoV, which also showed significant virus replication in Calu3 (p=0·039), Caco2 (p=0·0009), Huh7 (p=0·0012), and 293T (p=0·0017) cells, but not in U251 cells (figure 1B).
一般的に、SARS-CoV-2の細胞指向性はSARS-CoVのものと類似していました。 SARS-CoVは、Calu3(p=0.039)、Caco2(p=0.0009)、Huh7(p=0.0012)、および293T(p=0.0017)細胞でも顕著なウイルス複製が見られましたが、U251細胞では見られませんでした(図1B)。
Although SARS-CoV showed higher replication capacity in Caco2 cells than in Calu3 cells (>3·5 log difference between Caco2 and Calu3 at 120 hpi; p=0·0098), SARS-CoV-2 replicated efficiently in both Caco2 and Calu3 cells (<0·1 log difference between Caco2 and Calu3 at 120 hpi; p=0·52).
SARS-CoV は Caco2 細胞の方が Calu3 細胞よりも高い複製能力を示したものの (120 hpi で Caco2 と Calu3 の log 差は 3.5 超、p=0.0098)、SARS-CoV-2 は Caco2 細胞と Calu3 細胞の両方で効率的に複製されました (120 hpi で Caco2 と Calu3 の log 差は 0.1 未満、p=0.52)。
These findings were supported by the AUC analysis, which showed that the total virus production in Calu3 cells infected by SARS-CoV-2 was significantly higher than that of SARS-CoV (p=0·0022), but the total virus production in Caco2 cells infected by SARS-CoV was significantly higher than that of SARS-CoV-2 (p=0·015), over the 120 h period (figure 1C).
これらの結果は AUC 分析によって裏付けられ、120 時間にわたって SARS-CoV-2 に感染した Calu3 細胞の総ウイルス生産量は SARS-CoV よりも有意に高かった (p=0.0022) が、SARS-CoV に感染した Caco2 細胞の総ウイルス生産量は SARS-CoV-2 よりも有意に高かった (p=0.015) ことが示されました (図 1C)。
The difference in SARS-CoV-2 and SARS-CoV replication in Calu3 and Caco2 cells was further validated by assays to ascertain the median tissue culture infectious dose (appendix p 3).
Calu3 細胞と Caco2 細胞における SARS-CoV-2 と SARS-CoV の複製の違いは、組織培養の感染量中央値を確認するためのアッセイによってさらに検証されました (付録 3 ページ)。
Overall, despite SARS-CoV-2 and SARS-CoV sharing a similar profile of cellular tropism, the two viruses might differ in their capacity to infect or replicate in pulmonary, intestinal, and neuronal cells.
全体として、SARS-CoV-2 と SARS-CoV は細胞向性のプロファイルが類似しているにもかかわらず、2 つのウイルスは肺、腸、神経細胞に感染または複製する能力が異なる可能性があります。
Of 16 non-human cell lines tested (table), six were susceptible to SARS-CoV-2 infection over a period of 120 h, including cells originating from non-human primates (VeroE6 [p=0·013], FRhK4 [p=0·0031], and LLCMK2 [p<0·0001]), cat (CRFK [p=0·014]), rabbit (RK-13 [p=0·0064]), and pig (PK-15 [p<0·0001]; figure 2A).
試験した16の非ヒト細胞株(表)のうち、6つは120時間にわたってSARS-CoV-2感染の影響を受けやすく、その中には非ヒト霊長類由来の細胞(VeroE6 [p=0·013]、FRhK4 [p=0·0031]、LLCMK2 [p<0·0001])、ネコ(CRFK [p=0·014])、ウサギ(RK-13 [p=0·0064])、ブタ(PK-15 [p<0·0001]、図2A)が含まれていた。
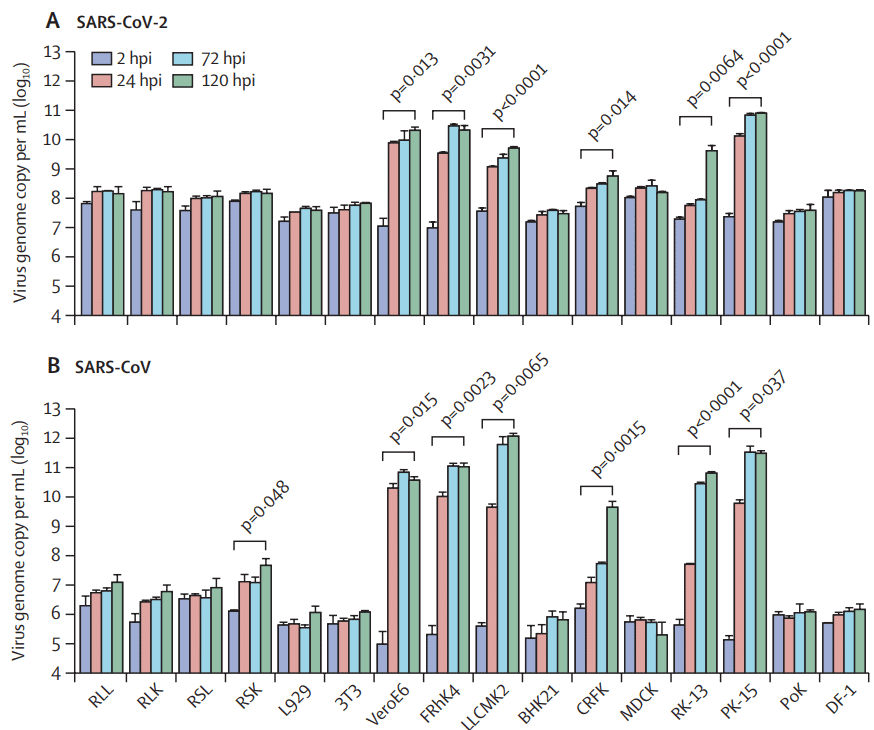
SARS-CoV-2 replicated most robustly in non-human primate cells and pig cells, as shown by a 3 log or greater increase in mean viral load over a period of 120 h in VeroE6, FRhK4, and PK-15 cells.
SARS-CoV-2は非ヒト霊長類細胞とブタ細胞で最も強力に複製され、VeroE6、FRhK4、PK-15細胞では120時間にわたって平均ウイルス量が3 log以上増加したことが示された。
Similar to the human cell tropism profile, the cellular tropism of SARS-CoV-2 in non-human cells largely matched that of SARS-CoV, which was also capable of infecting and replicating in non-human primate, cat, rabbit, and pig cells (figure 2B).
ヒト細胞向性プロファイルと同様に、非ヒト細胞における SARS-CoV-2 の細胞向性は、非ヒト霊長類、ネコ、ウサギ、ブタの細胞に感染して複製する能力も持つ SARS-CoV の細胞向性とほぼ一致しました (図 2B)。
Importantly, SARS-CoV, but not SARS-CoV-2, could replicate in Rhinolophus sinicus primary bat kidney cells (RSK; p=0·048).
重要なことに、SARS-CoV は Rhinolophus sinicus の一次コウモリ腎細胞で複製できましたが、SARS-CoV-2 は複製できませんでした (RSK; p=0.048)。
With the recombinant human ACE2 protein blocking assay, we confirmed that, similar to SARS-CoV, infection of SARS-CoV-2 was dependent on ACE2 (appendix p 4).
組み換えヒト ACE2 タンパク質阻害アッセイにより、SARS-CoV と同様に、SARS-CoV-2 の感染は ACE2 に依存していることを確認しました (付録 p 4)。
In addition to cellular tropism and replication kinetics profiles, cell damage induced by SARS-CoV-2 was also assessed in nine human (figure 3A) and 16 non-human (figure 3B) cell lines.
細胞向性と複製速度論プロファイルに加えて、SARS-CoV-2 によって誘発される細胞損傷も、9 つのヒト細胞株 (図 3A) と 16 の非ヒト細胞株 (図 3B) で評価されました。
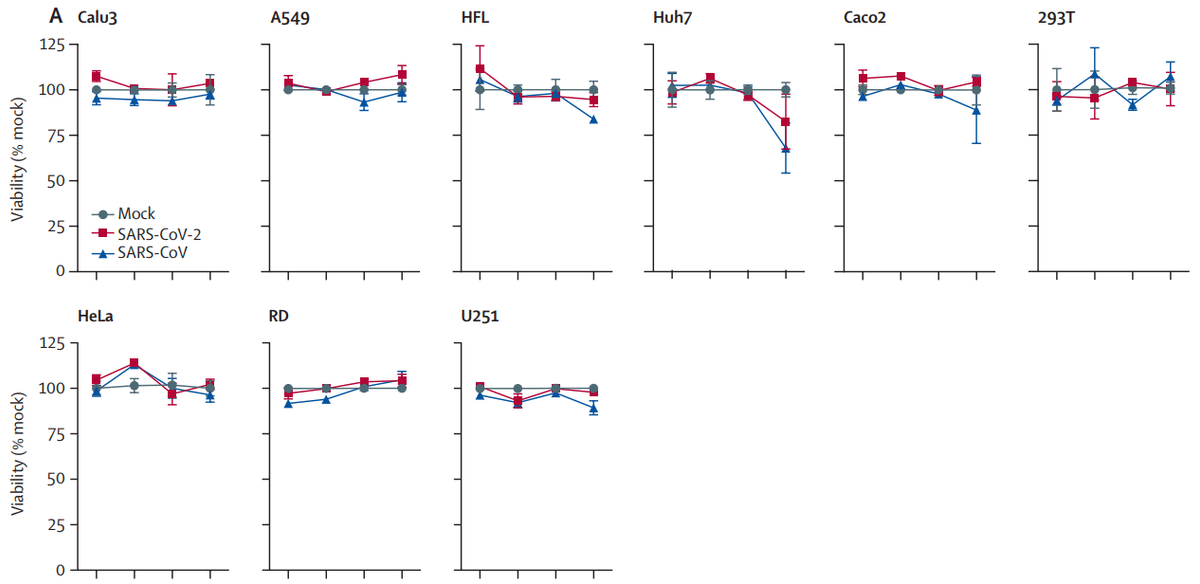
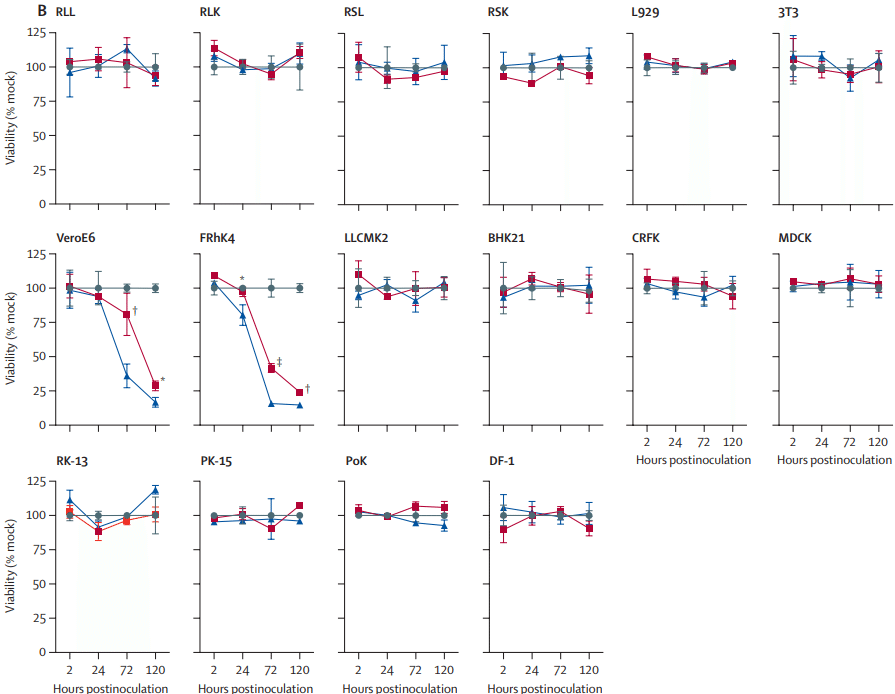
Among the 11 cell lines that supported SARS-CoV-2 replication (Calu3, Huh7, Caco2, 293T, U251, VeroE6, FRhK4, LLCMK2, CRFK, RK-13, and PK-15), SARS-CoV-2 only induced substantial cell damage in VeroE6 cells (28·7% viability at 120 hpi; p<0·0001) and FRhK4 cells (24·0% viability at 120 hpi; p<0·0001).
SARS-CoV-2 複製をサポートする 11 の細胞株 (Calu3、Huh7、Caco2、293T、U251、VeroE6、FRhK4、LLCMK2、CRFK、RK-13、および PK-15) のうち、SARS-CoV-2 は VeroE6 細胞 (120 hpi で 28.7% の生存率、p<0.0001) と FRhK4 細胞 (120 hpi で 24.0% の生存率、p<0.0001) でのみ大幅な細胞損傷を引き起こしました。
Typical cytopathic effects in VeroE6 and FRhK4 cells included cell rounding, detachment, degeneration, and syncytium formation (figure 4).
VeroE6 細胞および FRhK4 細胞における典型的な細胞変性効果には、細胞の丸み、剥離、変性、および合胞体形成が含まれます (図 4)。
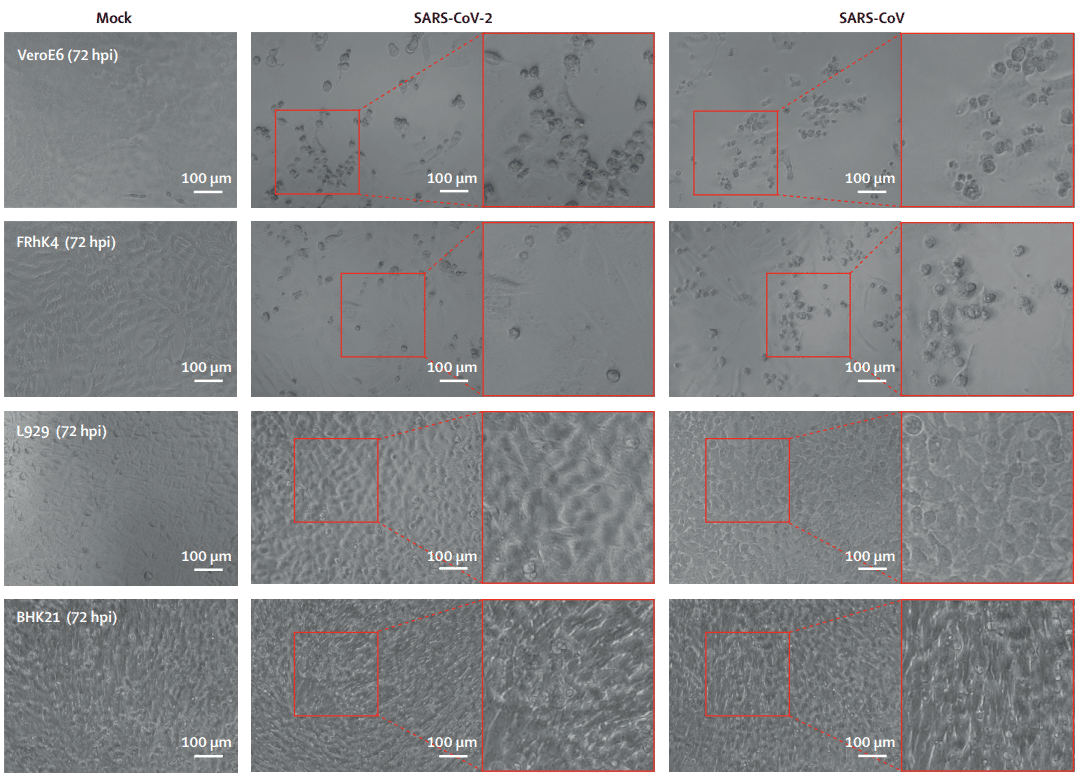
Despite robust SARS-CoV-2 replication in Calu3 and Caco2 cells, substantial cell death was not detected up to 120 hpi (at 120 hpi, Calu3 viability was 103% and Caco2 viability was 104%).
Calu3 細胞と Caco2 細胞における SARS-CoV-2 の強力な複製にもかかわらず、感染後 120 時間までは実質的な細胞死は検出されませんでした (感染後 120 時間で、Calu3 の生存率は 103%、Caco2 の生存率は 104%)。
To understand if SARS-CoV-2 would induce cell death in cells that supported efficient virus replication at a delayed timepoint, cell viability of SARS-CoV-2-infected Calu3, Caco2, LLCMK2, PK-15, and RK-13 cells was assessed at 7 days postinfection; cell death was not detected in these cell types (appendix p 5).
SARS-CoV-2 が、遅延した時点で効率的なウイルス複製をサポートする細胞で細胞死を引き起こすかどうかを理解するために、SARS-CoV-2 に感染した Calu3、Caco2、LLCMK2、PK-15、および RK-13 細胞の細胞生存率を感染後 7 日目に評価したところ、これらの細胞タイプでは細胞死は検出されませんでした (付録 p5)。
Although SARS-CoV-2 and SARS-CoV were inoculated with the same MOI, SARS-CoV-2 induced less cell damage than did SARS-CoV (figure 3).
SARS-CoV-2 と SARS-CoV は同じ MOI で接種されたが、SARS-CoV-2 は SARS-CoV よりも細胞損傷が少なかった (図 3)。
This observation was supported by the significantly higher percentage of cell viability in VeroE6 and FRhK4 cells infected by SARS-CoV-2 than in those infected by SARS-CoV, at multiple timepoints.
この観察結果は、SARS-CoV-2に感染したVeroE6細胞とFRhK4細胞の細胞生存率が、複数の時点でSARS-CoVに感染した細胞よりも有意に高かったことによって裏付けられました。
The AUC analysis also showed a significantly higher amount of viable cells on SARS-CoV-2 infection by comparison with SARS-CoV infection in both VeroE6 cells (p=0·016) and FRhK4 cells (p=0·0004) over the 120 h period (appendix p 6).
AUC分析では、120時間にわたって、VeroE6細胞(p=0.016)とFRhK4細胞(p=0.0004)の両方で、SARS-CoV-2感染時の生存細胞量がSARS-CoV感染時よりも有意に高かったことも示されました(付録p6)。
In parallel with the viral replication assays, SARS-CoV-2 antigen expression was detected in several representative cell types using antiserum against the SARS-CoV-2-NP antibody (appendix p 7).
ウイルス複製アッセイと並行して、SARS-CoV-2-NP抗体に対する抗血清を使用して、いくつかの代表的な細胞タイプでSARS-CoV-2抗原発現が検出されました(付録p7)。
By immunostaining and confocal microscopy, SARS-CoV-2-NP could be abundantly detected at as early as 16 hpi. SARS-CoV-2-NP appeared diffusely distributed across the cytoplasm of the infected cells (appendix p 8).
免疫染色と共焦点顕微鏡検査により、SARS-CoV-2-NPは感染後16時間で豊富に検出されました。SARS-CoV-2-NPは感染細胞の細胞質全体に拡散して分布しているように見えました(付録p8)。
The abundance of antigen expression corresponded with viral replication kinetics (appendix p 9).
抗原発現の豊富さは、ウイルス複製の動態と一致していました(付録p9)。
Abundant SARS-CoV-2-NP expression was detected in cell lines that supported SARS-CoV-2 replication, including VeroE6, Calu3, and Huh7 cells.
SARS-CoV-2複製をサポートする細胞株(VeroE6、Calu3、Huh7細胞など)で、豊富なSARS-CoV-2-NP発現が検出されました。
By contrast, viral antigen expression was not detected from non-susceptible BHK21 cells inoculated with SARS-CoV-2 (figure 5).
対照的に、SARS-CoV-2を接種した非感受性BHK21細胞ではウイルス抗原発現は検出されませんでした(図5)。
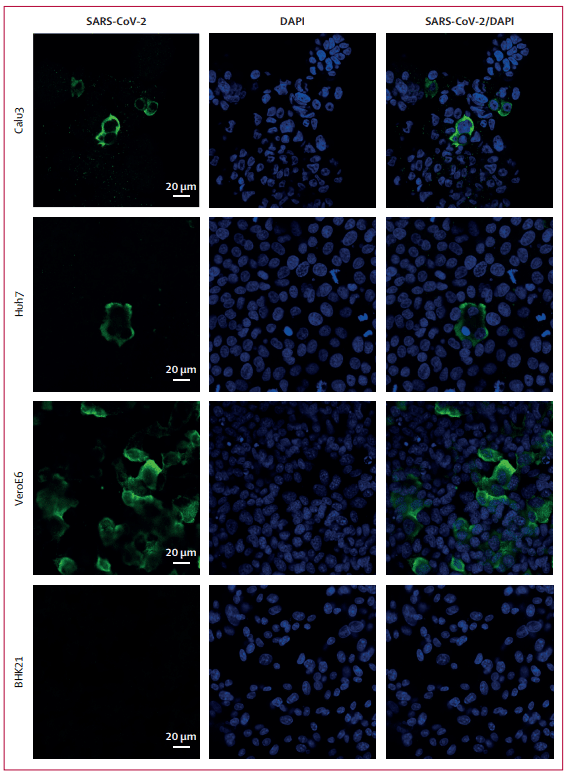
Discussion
In the past 3 months, most publications on COVID-19 have been observational studies in clinical cohorts, epidemiological investigations and forecasts, and in-silico genomic and structural analyses.
過去 3 か月間、COVID-19 に関する出版物のほとんどは、臨床コホート、疫学的調査と予測、およびコンピューターによるゲノムと構造の解析における観察研究でした。
Many basic virological questions of SARS-CoV-2 remain unanswered.
SARS-CoV-2 に関する多くの基本的なウイルス学的疑問は未解決のままです。
In this study, we systematically compared the differential cell tropism, viral replication kinetics, and cell damage profiles of SARS-CoV-2 and SARS-CoV.
この研究では、SARS-CoV-2 と SARS-CoV の異なる細胞向性、ウイルス複製速度、および細胞損傷プロファイルを体系的に比較しました。
Our data show biological characteristics of SARS-CoV-2 that might provide insights into understanding this virus's unique clinical manifestations and transmissibility and rationalising laboratory diagnostics.
私たちのデータは、SARS-CoV-2 の生物学的特性を示しており、このウイルスの独特な臨床症状と伝染性を理解し、臨床検査の合理化に役立つ可能性があります。
As expected from use of human ACE2 as an entry receptor, SARS-CoV-2 and SARS-CoV showed significant replication in Calu3 (pulmonary) cells, which corresponds with the abilities of these coronaviruses to cause lower respiratory tract infection.
ヒト ACE2 をエントリー レセプターとして使用することから予想されるように、SARS-CoV-2 と SARS-CoV は Calu3 (肺) 細胞で顕著な複製を示しました。これは、これらのコロナウイルスが下気道感染を引き起こす能力と一致しています。
By contrast, HCoV-229E, which primarily causes self-limiting upper respiratory tract infection, does not replicate efficiently in Calu3 cells.
対照的に、主に自己限定性の上気道感染を引き起こす HCoV-229E は、Calu3 細胞では効率的に複製されません。
SARS-CoV-2 replicated to comparable levels in both Calu3 and Caco2 (intestinal) cells, whereas SARS-CoV replicated significantly more efficiently in Caco2 than in Calu3 cells, with the same MOI.
SARS-CoV-2 は Calu3 細胞と Caco2 (腸) 細胞の両方で同程度に複製されましたが、SARS-CoV は、同じ MOI で、Caco2 細胞よりも Calu3 細胞で大幅に効率的に複製されました。
This finding supports the higher incidence of diarrhoea in patients with SARS than in COVID-19 patients.
この結果は、SARS 患者の下痢の発生率が COVID-19 患者よりも高いことを裏付けています。
Among 337 patients with COVID-19 reported in four large clinical cohorts, only 20 (6%) developed diarrhoea.
4つの大規模臨床コホートで報告されたCOVID-19患者337人のうち、下痢を発症したのはわずか20人(6%)でした。
By contrast, diarrhoea was the most common extrapulmonary clinical manifestation of SARS and was reported in up to 130 (20%) of 647 SARS patients.
対照的に、下痢はSARSの最も一般的な肺外臨床症状であり、647人のSARS患者のうち最大130人(20%)で報告されました。
Clinical deterioration of SARS commonly occurred 1 week after symptom onset and was typically accompanied by non-inflammatory watery diarrhoea.
SARSの臨床的悪化は、症状発現後1週間で一般的に発生し、典型的には非炎症性の水様性下痢を伴いました。
The mean viral load in stool specimens from SARS patients was 1·2 log10 copies per mL higher than that in nasopharyngeal aspirate specimens at days 10–15 after symptom onset.
SARS患者の便検体の平均ウイルス量は、症状発現後10~15日目の鼻咽頭吸引検体よりも1mLあたり1.2 log10コピー高かったです。
Among other non-pulmonary cell lines, SARS-CoV-2 and SARS-CoV both showed significant replication in Huh7 (hepatic) and 293T (renal) cells.
その他の非肺細胞株では、SARS-CoV-2とSARS-CoVはともにHuh7(肝臓)細胞と293T(腎臓)細胞で顕著な複製を示した。
Up to 43% of patients with COVID-19 and 44% of SARS patients developed hepatic dysfunction.
COVID-19患者の最大43%とSARS患者の44%が肝機能障害を発症した。
Patients with COVID-19 needing intensive care had significantly higher amounts of hepatic aminotransferases than did those not needing intensive care.
集中治療を必要とするCOVID-19患者は、集中治療を必要としない患者よりも肝臓アミノトランスフェラーゼの量が有意に高かった。
In terms of renal manifestations, 3–7% of patients with COVID-19 developed acute kidney injury or needed renal replacement therapy.
腎臓の症状に関しては、COVID-19患者の3~7%が急性腎障害を発症するか、腎代替療法が必要となった。
Similarly, 7% of SARS patients developed acute renal impairment, which was associated with a significantly higher mortality rate than in those without acute renal impairment (91·7% vs 8·8%; p<0·0001).
同様に、SARS 患者の 7% が急性腎機能障害を発症し、急性腎機能障害のない患者よりも死亡率が有意に高かった (91.7% 対 8.8%、p<0.0001)。
The prognostic value of hepatic and renal dysfunction in patients with COVID-19 should be further investigated.
COVID-19 患者の肝機能障害および腎機能障害の予後価値については、さらに調査する必要がある。
Our data show modest replication of SARS-CoV-2, but not SARS-CoV, in U251 (neuronal) cells.
我々のデータは、U251(神経)細胞でSARS-CoV-2が適度に複製されているが、SARS-CoVは複製されていないことを示している。
This finding might correlate with the observation that up to 9% of patients with COVID-19 develop confusion or dizziness, and some are reported to have anosmia and ageusia, whereas these neurological manifestations were rarely reported in SARS patients.
この発見は、COVID-19患者の最大9%が混乱やめまいを呈し、一部の患者は嗅覚障害や味覚障害を呈すると報告されているが、これらの神経学的症状はSARS患者ではほとんど報告されていないという観察と相関している可能性がある。
The potential for SARS-CoV-2 to directly infect the CNS needs closer scrutiny, because another betacoronavirus (HCoV-OC43) has been associated with fatal encephalitis in an 11-month-old boy with severe combined immunodeficiency.
SARS-CoV-2がCNSに直接感染する可能性については、より綿密な調査が必要である。なぜなら、別のベータコロナウイルス(HCoV-OC43)が重症複合免疫不全症の11か月の男児の致命的な脳炎と関連しているからである。
Our data also provide new insights into the apparently high transmissibility of SARS-CoV-2.
私たちのデータは、SARS-CoV-2 の明らかに高い伝染性に関する新たな洞察も提供します。
In the AUC analysis, SARS-CoV-2 showed more efficient replication in Calu3 cells than did SARS-CoV.
AUC 分析では、SARS-CoV-2 は SARS-CoV よりも Calu3 細胞でより効率的に複製されました。
Both SARS-CoV-2 and SARS-CoV can use human ACE2 as a cell entry receptor.
SARS-CoV-2 と SARS-CoV はどちらも、細胞侵入受容体としてヒト ACE2 を使用できます。
However, the spike protein S1 subunits of the two viruses share only approximately 70% amino acid identity.
ただし、2 つのウイルスのスパイク タンパク質 S1 サブユニットは、約 70% のアミノ酸同一性しか共有していません。
Significant amino acid differences between SARS-CoV-2 and SARS-CoV are located in the ectodomain of the spike protein, which is important for receptor binding.
SARS-CoV-2とSARS-CoVの重要なアミノ酸の違いは、受容体結合に重要なスパイクタンパク質のエクトドメインにあります。
Findings of a study showed that the binding affinity between the SARS-CoV-2 spike ectodomain and human ACE2 was approximately 10–20-fold higher than the binding affinity between the SARS-CoV spike ectodomain and human ACE2.
研究結果によると、SARS-CoV-2スパイクエクトドメインとヒトACE2の結合親和性は、SARS-CoVスパイクエクトドメインとヒトACE2の結合親和性よりも約10~20倍高いことが示されました。
This higher receptor-binding capacity might facilitate virus entry into pulmonary cells and lead to more efficient person-to-person transmission through direct or indirect contact with respiratory droplets from patients with COVID-19.
この高い受容体結合能力により、ウイルスが肺細胞に侵入しやすくなり、COVID-19 患者の呼吸器飛沫との直接的または間接的な接触を通じて、より効率的な人から人への感染につながる可能性があります。
Although SARS-CoV-2 and SARS-CoV showed similar tropism in 16 non-human cell lines derived from various animal species, SARS-CoV, but not SARS-CoV-2, showed significant replication in RSK cells.
SARS-CoV-2 と SARS-CoV は、さまざまな動物種に由来する 16 の非ヒト細胞株で同様の向性を示しましたが、SARS-CoV は RSK 細胞で顕著な複製を示しましたが、SARS-CoV-2 はそうではありませんでした。
We and others have previously identified R sinicus as the likely natural animal reservoir of SARS-like coronavirus.
私たちと他の研究者は以前、R sinicus が SARS 様コロナウイルスの自然動物リザーバーである可能性が高いことを特定しました。
SARS-CoV-2 has been postulated to originate from R sinicus bats, because the virus is phylogenetically most closely related to coronaviruses found in these bats.
SARS-CoV-2 は、R sinicus コウモリに由来すると推定されています。これは、このウイルスが系統学的にこれらのコウモリに見られるコロナウイルスに最も近いためです。
Our findings raise the possibility that SARS-CoV-2 has already adapted well to humans and, thus, the virus is no longer able to propagate well in R sinicus bat cells, providing a possible explanation for the efficient person-to-person transmission of COVID-19.
私たちの研究結果から、SARS-CoV-2はすでに人間によく適応しており、そのためこのウイルスはR sinicusコウモリの細胞内ではもはやうまく増殖できない可能性が浮上した。 これは、COVID-19の効率的な人から人への感染を説明する可能性がある。
Our cell culture model data are useful for optimising laboratory methods for studying COVID-19.
私たちの細胞培養モデルデータは、COVID-19 の研究のための実験方法を最適化するのに役立ちます。
First, we showed that SARS-CoV-2 replicated efficiently in non-human primate (VeroE6, FRhK4, LLCMK2), cat (CRFK), rabbit (RK-13), and pig (PK-15) cells.
まず、SARS-CoV-2 が非ヒト霊長類 (VeroE6、FRhK4、LLCMK2)、ネコ (CRFK)、ウサギ (RK-13)、ブタ (PK-15) の細胞で効率的に複製されることを示しました。
Non-human primates (including Rhesus macaques, cynomolgus macaques, African green monkeys, and common marmosets) were susceptible to infection with SARS-CoV, MERS-CoV, or both viruses.
非ヒト霊長類 (アカゲザル、カニクイザル、アフリカミドリザル、コモンマーモセットを含む) は、SARS-CoV、MERS-CoV、または両方のウイルスに感染しやすいことがわかりました。
SARS-CoV-infected domestic cats developed asymptomatic infection with virus shedding from their pharynx from days 2 to 14 post infection.
SARS-CoV に感染した飼いネコは、感染後 2 日目から 14 日目まで咽頭からウイルスを排出する無症候性の感染を起こしました。
Similarly, MERS-CoV-infected rabbits and domestic pigs also developed asymptomatic virus shedding from their respiratory tract.
同様に、MERS-CoV に感染したウサギと飼いブタも呼吸器から無症候性のウイルスを排出しました。
The susceptibility of mice transgenic for human ACE2 to SARS-CoV-2 infection should be assessed so animal models can be developed that could recapitulate the different disease severities of COVID-19 in humans (ranging from asymptomatic to fatal infection).
ヒトACE2をトランスジェニック化したマウスのSARS-CoV-2感染に対する感受性を評価し、ヒトにおけるCOVID-19のさまざまな重症度(無症状から致死的感染まで)を再現できる動物モデルを開発する必要があります。
Second, our data show that the abundance of SARS-CoV-2 antigen expression correlated with viral replication kinetics.
次に、私たちのデータは、SARS-CoV-2抗原発現の豊富さがウイルス複製動態と相関していることを示しています。
Although immunofluorescent antigen tests generally have lower sensitivity than do RT-PCR assays, this non-labour-intensive diagnostic could be considered as a screening test in laboratories without the resources and expertise for RT-PCR assays for COVID-19.
免疫蛍光抗原検査は一般にRT-PCR検査よりも感度が低いが、この労力のかからない診断法は、COVID-19のRT-PCR検査のためのリソースや専門知識がない研究室でのスクリーニング検査として考えることができる。
Finally, our data show that among the 25 cell lines assessed, cytopathic effects were only seen in VeroE6 and FRhK4 cells after SARS-CoV-2 inoculation for up to 120 hpi.
最後に、私たちのデータは、評価した25の細胞株のうち、SARS-CoV-2接種後120hpiまでの細胞変性効果はVeroE6細胞とFRhK4細胞でのみ見られたことを示しています。
These findings are important for optimisation of antiviral assays based on cell protection assessment, because cell lines without obvious cytopathic effects might lead to overestimation of cell viability and drug efficacy.
これらの知見は、細胞保護評価に基づく抗ウイルス検査の最適化にとって重要です。なぜなら、明らかな細胞変性効果のない細胞株は、細胞生存率と薬効の過大評価につながる可能性があるからです。
Our study had several limitations.
私たちの研究にはいくつかの限界がありました。
First, cell line tropism might not fully represent how SARS-CoV-2 replicates and affects human organs in the physiological state.
第一に、細胞株の向性は、生理的状態で SARS-CoV-2 が複製してヒトの臓器に影響を及ぼす方法を完全には表していない可能性があります。
Thus, our cell line susceptibility results and the clinical manifestations of patients with COVID-19 might not completely accord.
したがって、私たちの細胞株感受性の結果と COVID-19 患者の臨床症状は完全には一致しない可能性があります。
It is essential to further characterise virus–host interactions in more physiological models, such as ex-vivo human organ tissue and human organoids from patients of different ages, sexes, and with underlying diseases.
さまざまな年齢、性別、基礎疾患を持つ患者の ex-vivo ヒト臓器組織やヒトオルガノイドなど、より生理的なモデルでウイルスと宿主の相互作用をさらに特徴付けることが重要です。
Nevertheless, findings in cell line susceptibility studies of MERS-CoV were highly corroborative with those seen in patients with MERS.
それでも、MERS-CoV の細胞株感受性研究の知見は、MERS 患者で見られるものと非常によく一致しています。
Second, for all our experiments, we used one viral isolate that was highly homologous to other reported SARS-CoV-2 isolates.
第二に、すべての実験で、報告されている他の SARS-CoV-2 分離株と非常に相同性の高い 1 つのウイルス分離株を使用しました。
Isolates with additional amino acid mutations, particularly involving the receptor binding domain, should be studied to identify viral factors that might affect virus entry to host cells and virus replication kinetics.
特に受容体結合ドメインに関連する追加のアミノ酸変異を持つ分離株を研究して、ウイルスの宿主細胞への侵入やウイルス複製の速度に影響を与える可能性のあるウイルス因子を特定する必要があります。
Finally, the viral kinetics of SARS-CoV-2 in other human cell lines (eg, cardiomyocytes) and animal cell lines should be assessed to investigate virus-induced damage in cardiac cells and potential animal reservoirs.
最後に、他のヒト細胞株 (心筋細胞など) および動物細胞株における SARS-CoV-2 のウイルス速度を評価して、心臓細胞および潜在的な動物リザーバーにおけるウイルス誘発性損傷を調査する必要があります。
以下省略。
この記事が気に入ったらサポートをしてみませんか?