SARS-CoV-2のヌクレオカプシド変異は複製と病原性を増強
Nucleocapsid mutations in SARS-CoV-2 augment replication and pathogenesis
SARS-CoV-2のヌクレオカプシド変異は複製と病原性を増強する
元→Nucleocapsid mutations in SARS-CoV-2 augment replication and pathogenesis | PLOS Pathogens
Abstract
While SARS-CoV-2 continues to adapt for human infection and transmission, genetic variation outside of the spike gene remains largely unexplored.
SARS-CoV-2 はヒトへの感染と伝染に適応し続けていますが、スパイク遺伝子以外の遺伝的変異はほとんど未調査のままです。
This study investigates a highly variable region at residues 203–205 in the SARS-CoV-2 nucleocapsid protein.
この研究では、SARS-CoV-2 ヌクレオカプシドタンパク質の残基 203~205 の非常に可変性の高い領域を調査します。
Recreating a mutation found in the alpha and omicron variants in an early pandemic (WA-1) background, we find that the R203K+G204R mutation is sufficient to enhance replication, fitness, and pathogenesis of SARS-CoV-2.
初期パンデミック (WA-1) 背景のアルファおよびオミクロン変異体に見られる変異を再現したところ、R203K+G204R 変異は SARS-CoV-2 の複製、適応度、および病原性を高めるのに十分であることがわかりました。
The R203K+G204R mutant corresponds with increased viral RNA and protein both in vitro and in vivo.
R203K+G204R 変異体は、in vitro と in vivo の両方でウイルス RNA とタンパク質の増加に対応しています。
Importantly, the R203K+G204R mutation increases nucleocapsid phosphorylation and confers resistance to inhibition of the GSK-3 kinase, providing a molecular basis for increased virus replication.
重要なことに、R203K+G204R 変異はヌクレオカプシドのリン酸化を増加させ、GSK-3 キナーゼの阻害に対する耐性を付与し、ウイルス複製の増加の分子的基礎を提供します。
Notably, analogous alanine substitutions at positions 203+204 also increase SARS-CoV-2 replication and augment phosphorylation, suggesting that infection is enhanced through ablation of the ancestral ‘RG’ motif.
注目すべきことに、位置 203+204 での類似のアラニン置換も SARS-CoV-2 の複製を増加させ、リン酸化を増強します。これは、祖先の「RG」モチーフの除去によって感染が強化されることを示唆しています。
Overall, these results demonstrate that variant mutations outside spike are key components in SARS-CoV-2’s continued adaptation to human infection.
全体として、これらの結果は、スパイク以外の変異体変異が、SARS-CoV-2 がヒト感染に継続的に適応するための重要な要素であることを示しています。
Author summary
Since its emergence, SARS-CoV-2 has continued to adapt for human infection resulting in the emergence of variants with unique genetic profiles.
SARS-CoV-2 は出現以来、ヒト感染に適応し続け、その結果、独自の遺伝子プロファイルを持つ変異体が出現しました。
Most studies of genetic variation have focused on spike, the target of currently available vaccines, leaving the importance of variation elsewhere understudied.
遺伝子変異に関する研究のほとんどは、現在利用可能なワクチンのターゲットであるスパイクに焦点を当てており、他の変異の重要性は十分に研究されていません。
Here, we characterize a highly variable motif at residues 203–205 in nucleocapsid.
ここでは、ヌクレオカプシドの残基 203~205 にある非常に可変性の高いモチーフを特徴付けます。
Recreating the prominent nucleocapsid R203K+G204R mutation in an early pandemic background, we show that this mutation is alone sufficient to enhance SARS-CoV-2 replication and pathogenesis.
パンデミック初期の背景で顕著なヌクレオカプシド R203K+G204R 変異を再現し、この変異だけで SARS-CoV-2 の複製と病原性を高めるのに十分であることを示しています。
We also link augmentation of SARS-CoV-2 infection by the R203K+G204R mutation to its modulation of nucleocapsid phosphorylation.
また、R203K+G204R 変異による SARS-CoV-2 感染の増強と、ヌクレオカプシドのリン酸化の調節を関連付けています。
Finally, we characterize an analogous alanine double substitution at positions 203–204.
最後に、位置 203~204 の類似のアラニン二重置換を特徴付けます。
This mutant was found to mimic R203K+G204R, suggesting augmentation of infection occurs by disrupting the ancestral sequence.
この変異体は R203K+G204R を模倣していることが判明しており、感染の増強は祖先の配列を破壊することによって起こることを示唆しています。
Together, our findings illustrate that mutations outside of spike are key components of SARS-CoV-2’s adaptation to human infection.
これらを合わせると、スパイク以外の変異が SARS-CoV-2 のヒト感染への適応の重要な構成要素であることが示されます。
Introduction
The emergence of severe acute respiratory syndrome coronavirus 2 (SARS)-CoV-2 is the most significant infectious disease event of the 21st century.
重症急性呼吸器症候群コロナウイルス2(SARS)-CoV-2の出現は、21世紀で最も重要な感染症イベントです。
Since its initial expansion, SARS-CoV-2 has continued to adapt for human infection and transmission, resulting in several variants of concern.
SARS-CoV-2 は、最初の拡大以来、ヒトへの感染と伝染に適応し続け、懸念されるいくつかの変異体を生み出してきました。
While most mutations occur within a single lineage, a small number are shared across multiple variants.
ほとんどの変異は単一の系統内で発生しますが、少数の変異は複数の変異体で共有されています。
Spike mutations have dominated SARS-CoV-2 variant research, owing to concerns that they enhance replication, augment transmission, or allow escape from immunity.
スパイク変異は、複製を促進したり、感染を増強したり、免疫からの逃避を可能にしたりする懸念があるため、SARS-CoV-2変異体の研究で主流を占めてきました。
However, less attention has been focused on mutations outside spike, despite the existence of other “mutational hotspots” in the genome.
しかし、ゲノム内に他の「変異ホットスポット」が存在するにもかかわらず、スパイク以外の変異にはあまり注目が集まっていません。
The SARS-CoV-2 nucleocapsid (N) gene is one hotspot for coding mutations, particularly at amino acid residues 203–205 within its serine rich (SR) domain.
SARS-CoV-2 ヌクレオカプシド (N) 遺伝子は、特にセリンリッチ (SR) ドメイン内のアミノ酸残基 203~205 で、コード変異のホットスポットの 1 つです。
Three prominent mutations occur in this region including R203K+G204R, a double substitution (KR mt) present in the alpha, gamma, and omicron variants; T205I present in the beta variant; and R203M that occurs in the kappa and delta variants.
この領域には、アルファ、ガンマ、オミクロン変異体に存在する二重置換(KR mt)であるR203K+G204R、ベータ変異体に存在するT205I、カッパおよびデルタ変異体で発生するR203Mの3つの顕著な変異が発生します。
Together, this genetic variation and convergent evolution in residues 203–205 suggests positive selection in this motif of N.
まとめると、 この遺伝的変異と残基 203~205 の収束進化は、この N モチーフにおける正の選択を示唆しています。
Here, we utilize our reverse genetic system to generate the KR nucleocapsid mutation in the ancestral WA-1 strain of SARS-CoV-2.
ここでは、逆遺伝学システムを利用して、SARS-CoV-2 の祖先 WA-1 株の KR ヌクレオカプシド変異を生成します。
This change alone was sufficient to increase viral replication in respiratory cells and exhibited enhanced fitness in direct competition studies with wild type (WT) SARS-CoV-2.
この変化だけで、呼吸器細胞でのウイルス複製を増やすのに十分であり、野生型 (WT) SARS-CoV-2 との直接競合研究で適応度が向上しました。
In the hamster model, the KR mutant (mt) enhanced pathogenesis and outcompeted WT in direct competition.
ハムスターモデルでは、KR 変異体 (mt) は病原性を高め、直接競合で WT に打ち勝ちました。
We subsequently found that the KR mt corresponds with increased viral RNA both in vitro and in vivo.
その後、KR mt は in vitro と in vivo の両方でウイルス RNA の増加と一致することがわかりました。
Notably, we observed that the KR mt resulted in augmented nucleocapsid phosphorylation relative to WT SARS-CoV-2; similar increases in N phosphorylation were also seen in the alpha and kappa variants.
特に、KR mt は WT SARS-CoV-2 と比較してヌクレオカプシドのリン酸化を増強することがわかりました。同様の N リン酸化の増加は、アルファおよびカッパ変異体でも見られました。
Importantly, the KR mt was more resistant to GSK-3 kinase inhibition relative to WT SARS-CoV-2, suggesting that the KR mt alters interactions with N targeting kinases.
重要なのは、KR mt は WT SARS-CoV-2 に比べて GSK-3 キナーゼ阻害に対する耐性が強く、KR mt が N 標的キナーゼとの相互作用を変化させていることを示唆している点です。
Finally, an analogous alanine double substitution mutant at position 203+204 (AA mt) also increased fitness and altered phosphorylation relative to WT SARS-CoV-2.
最後に、位置 203+204 の類似のアラニン二重置換変異体 (AA mt) も、WT SARS-CoV-2 に比べて適応度が高まり、リン酸化が変化しました。
Together, these results suggest that disruption of the ancestral “RG” motif in nucleocapsid augments infection, fitness, and pathogenesis of SARS-CoV-2.
まとめると、これらの結果は、ヌクレオカプシドの祖先「RG」モチーフの破壊が SARS-CoV-2 の感染、適応度、および病因を増強することを示唆しています。
Results
Genetic analysis of a highly variable motif of SARS-CoV-2 nucleocapsid
SARS-CoV-2 ヌクレオカプシドの非常に可変性の高いモチーフの遺伝子解析
Using SARS-CoV-2 genomic data from the GISAID database, we binned each sequence by month of collection and performed an in silico search for variation at residues 203–205 within nucleocapsid (Fig 1A).
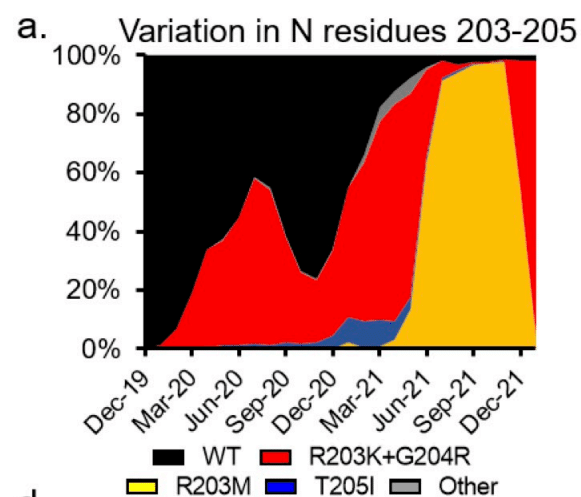
Three prominent mutations emerged from this analysis.
この分析から、3 つの顕著な変異が明らかになりました。
The first is the R203K+G204R double substitution (KR mt), present in the alpha, gamma, and omicron variants (Fig 1D).
1 つ目は R203K+G204R 二重置換 (KR mt) で、アルファ、ガンマ、オミクロン変異体に存在します (図 1D)。
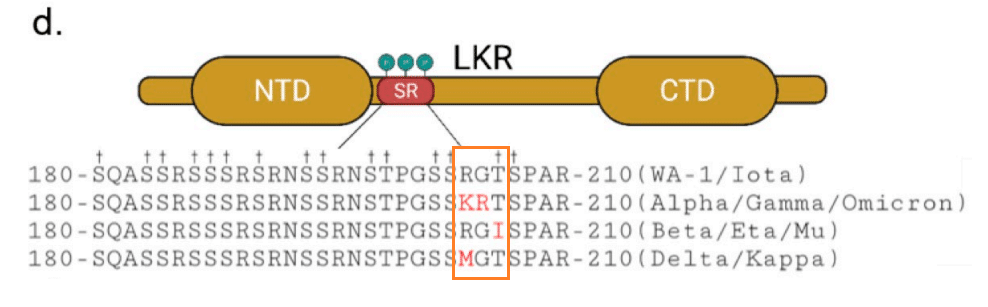
Historically, the KR mt has been the most abundant mutation in this region, emerging early in the pandemic and reaching 73% of reported sequences in April 2021 (Fig 1A and S1 Table).
歴史的に、KR mt はこの地域で最も豊富な変異であり、パンデミックの初期に出現し、2021 年 4 月には報告された配列の 73% に達しました (図 1A および S1 表)。
The second prominent mutation, T205I, is present in the beta, eta, and mu lineages (Fig 1D).
2番目に顕著な変異であるT205Iは、ベータ、イータ、ミュー系統に存在します(図1D)。
While also emerging early in the pandemic, T205I is a minority variant which peaked at 9% in February 2021 (Fig 1A and S1 Table).
パンデミックの初期に出現したT205Iは、少数派の変異体であり、2021年2月に9%でピークに達しました(図1AおよびS1表)。
The third prominent variant mutation is R203M, present in the delta and kappa variants (Fig 1D).
3 番目に目立つ変異体は R203M で、デルタ変異体とカッパ変異体で見られます (図 1D)。
Interestingly, while the R203M mutation was first detected in March 2020, it persisted as a rare (<1%) variant until April 2021 when it began expanding rapidly, reaching 97% of all reported sequences by November 2021, displacing the KR and T205I mutations (Fig 1A and S1 Table).
興味深いことに、R203M 変異は 2020 年 3 月に初めて検出されましたが、2021 年 4 月までまれな変異体 (<1%) として存続し、その後急速に拡大し、2021 年 11 月までに報告されたすべての配列の 97% に達し、KR 変異体と T205I 変異体を置き換えました (図 1A と S1 表)。
However, with the recent emergence of the omicron variant, the KR mt has regained prominence, displacing R203M as the most common mutation and reaching 93% of all newly reported sequences in January 2022.
しかし、最近オミクロン変異体が出現したことで、KR mt が再び目立つようになり、最も一般的な変異体として R203M を置き換え、2022 年 1 月には新たに報告されたすべての配列の 93% に達しました。
Together, these data reveal a complex pattern of genetic variation and convergent evolution for residues 203–205 of SARS-CoV-2 N.
これらのデータを合わせると、SARS-CoV-2 N の残基 203~205 の遺伝的変異と収束進化の複雑なパターンが明らかになります。
To determine if mutations in this variable motif have the potential to enhance infection, we evaluated the replication kinetics of SARS-CoV-2 variants.
この可変モチーフの変異が感染を増強する可能性があるかどうかを判断するために、SARS-CoV-2 変異体の複製速度を評価しました。
Two cell models were selected for this analysis: Vero E6 (commonly used for propagation and titration of SARS-CoV-2) and Calu-3 2b4 (a respiratory cell line used to study coronavirus and influenza infection).
この分析では、2 つの細胞モデルが選択されました。Vero E6 (SARS-CoV-2 の増殖と滴定によく使用される) と Calu-3 2b4 (コロナウイルスとインフルエンザの感染を研究するために使用される呼吸器細胞株) です。
Briefly, Vero E6 or Calu-3 2b4 cells were inoculated at a low multiplicity of infection (MOI) of 0.01 plaque forming units/cell with the early pandemic Washington-1 (WA-1) strain or a SARS-CoV-2 variant and replication kinetics monitored for 48 hours post infection (hpi).
簡単に説明すると、Vero E6 または Calu-3 2b4 細胞に、初期パンデミックの Washington-1 (WA-1) 株または SARS-CoV-2 変異株を 0.01 プラーク形成単位/細胞という低い感染多重度 (MOI) で接種し、感染後 48 時間 (hpi) にわたって複製速度をモニタリングしました。
In Vero E6 cells, while the alpha and beta variants replicated to equivalent endpoint titers compared to WA-1, both variants had slightly lower titers at 24 hpi (Figs 1B and S1A).
Vero E6 細胞では、アルファ変異株とベータ変異株は WA-1 と比較して同等のエンドポイント力価に複製されましたが、24 hpi では両方の変異株の力価がわずかに低くなりました (図 1B および S1A)。
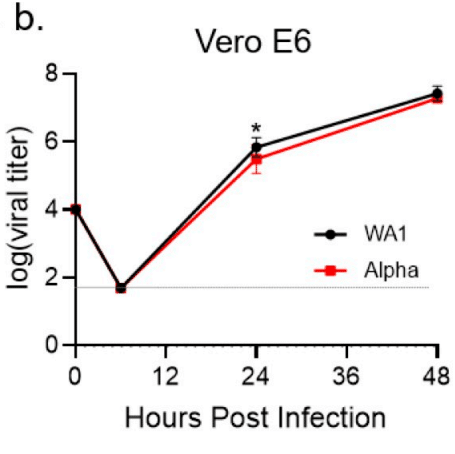
In contrast, the kappa variant replicated to ~15-fold lower titer than WA-1 throughout infection (S1A Fig), potentially due to processing mutations in SARS-CoV-2 spike shared with the delta variant.
対照的に、カッパ変異体は感染中、WA-1よりも約15倍低い力価で複製されました(S1A図)。これは、デルタ変異体と共有されているSARS-CoV-2スパイクの処理変異が原因である可能性があります。
Interestingly, in Calu-3 2b4 cells, the alpha variant replicated to a 5.6 fold higher endpoint titer compared to WA-1 (Fig 1C).
興味深いことに、Calu-3 2b4 細胞では、アルファ変異体は WA-1 と比較して 5.6 倍高いエンドポイント力価で複製されました (図 1C)。
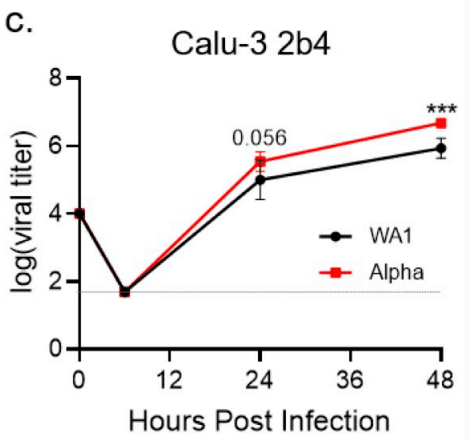
In contrast, the beta variant replicated to a lower (2.7 fold) mean endpoint titer compared to WA-1 while the kappa variant showed no significant differences with WA-1 (S1B Fig).
対照的に、ベータ変異体は WA-1 と比較して低い (2.7 倍) 平均エンドポイント力価で複製されましたが、カッパ変異体は WA-1 と有意差を示しませんでした (S1B 図)。
Together, these data demonstrate that SARS-CoV-2 variants harboring N mutations have altered replication kinetics, suggesting N mutants may modulate infection.
まとめると、これらのデータは、N 変異体を含む SARS-CoV-2 変異体は複製速度が変化していることを示しており、N 変異体が感染を調節する可能性があることを示唆しています。
The KR and R203M mts alone are sufficient to increase viral replication
KRとR203M mtsだけでもウイルスの複製を増やすのに十分である
Because the alpha variant exhibited increased replication in Calu-3 2b4 cells, we hypothesized that the KR mt may enhance infection.
アルファ変異体はCalu-3 2b4細胞で複製が増加したため、KR mtが感染を増強する可能性があるという仮説を立てました。
To study the effects of the KR mt in isolation, we utilized a SARS-CoV-2 reverse genetic system to recreate the KR mt in a WA-1 background (Fig 1E).
KR mt単独の効果を調べるために、SARS-CoV-2逆遺伝学システムを使用して、WA-1バックグラウンドでKR mtを再現しました(図1E)。
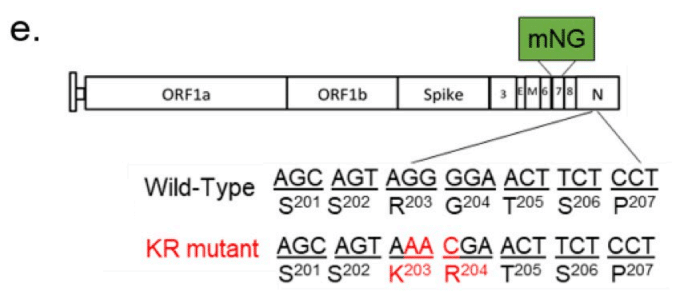
In addition, due to gain-of-function concerns, the accessory protein ORF7 was replaced with mNeonGreen (mNG), which reduces but does not eliminate disease in golden Syrian hamsters (S2 Fig).
さらに、機能獲得の懸念から、補助タンパク質 ORF7 を mNeonGreen (mNG) に置き換えました。これにより、ゴールデンシリアンハムスターの病気は軽減されますが、完全には治りません (S2 図)。
After recovery of recombinant virus, we evaluated the KR mt’s effects on SARS-CoV-2 replication.
組み換えウイルスの回収後、KR mt が SARS-CoV-2 複製に与える影響を評価しました。
Both Vero E6 and Calu-3 2b4 cells were infected at a low MOI (0.01) with either SARS-CoV-2 WA-1 harboring the mNG reporter (herein referred to as WT) or the KR mt and viral titer monitored for 48 hpi.
Vero E6 細胞と Calu-3 2b4 細胞の両方を、mNG レポーター を保有する SARS-CoV-2 WA-1 (以下、WT と呼ぶ) または KR mt のいずれかに低い MOI (0.01) で感染させ、48 hpi までウイルス力価をモニタリングした。
Like the alpha variant, the KR mt grew to a lower titer at 24 hpi, but had an equivalent endpoint titer in Vero E6 cells (Fig 1F).
アルファ変異体と同様に、KR mt は 24 hpi でより低い力価にまで成長したが、Vero E6 細胞では同等のエンドポイント力価を示した (図 1F)。
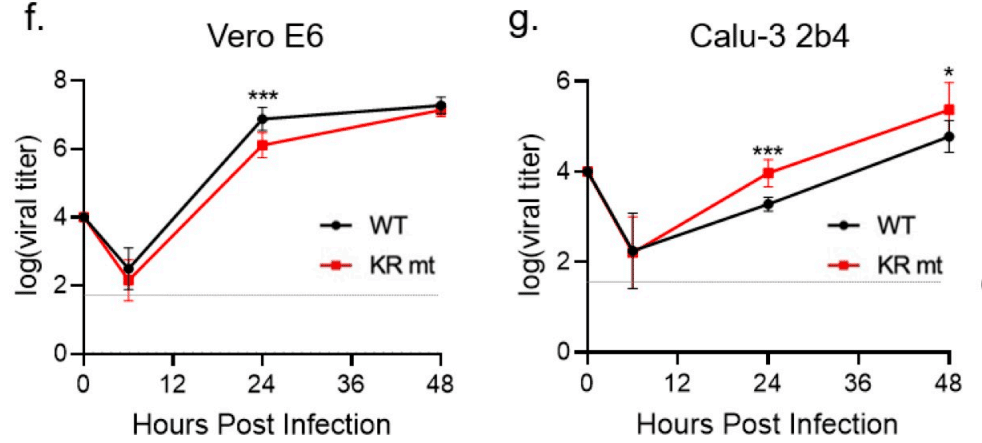
Notably, in Calu-3 2b4 cells, the KR mt had increased viral titer at both 24 and 48 hpi compared to WT SARS-CoV-2 (Fig 1G).
特に、Calu-3 2b4 細胞では、KR mt は 24 hpi と 48 hpi の両方で WT SARS-CoV-2 と比較してウイルス力価が増加した (図 1G)。
These data suggest the KR mt in nucleocapsid alone is sufficient to enhance viral replication.
これらのデータは、ヌクレオカプシド内の KR mt だけでウイルス複製を増強するのに十分であることを示唆している。
Next, we wanted to examine the ability of other variant mutations in the 203–205 motif of nucleocapsid to enhance SARS-CoV-2 replication.
次に、ヌクレオカプシドの 203~205 モチーフにおける他の変異体が SARS-CoV-2 の複製を強化する能力を調べたいと考えました。
Using our reverse genetic system, we recreated the R203M mutation in a WA-1 mNG background and evaluated its replication in Vero E6 and Calu-3 2b4 cells (S3 Fig).
リバースジェネティクスシステムを使用して、WA-1 mNG バックグラウンドで R203M 変異を再現し、Vero E6 および Calu-3 2b4 細胞での複製を評価しました (S3 図)。
Interestingly, like the KR mt, in Vero E6 cells the R203M mutant grew to lower titer at 24 hpi but had a similar endpoint titer compared to WT SARS-CoV-2 (S3B Fig).
興味深いことに、KR mt と同様に、Vero E6 細胞では R203M 変異体は 24 hpi でより低い力価に成長しましたが、WT SARS-CoV-2 と比較してエンドポイント力価は類似していました (S3B 図)。
In Calu-3 2b4 cells, the R203M mutant again mimicked the KR mt, growing to a higher titer than WT at 24 and 48 hpi (S3C Fig).
Calu-3 2b4 細胞では、R203M 変異体は再び KR mt を模倣し、24 hpi および 48 hpi で WT よりも高い力価に成長しました (S3C 図)。
These data suggest that like the KR mt, the R203M mutation alone is sufficient to enhance SARS-CoV-2 replication.
これらのデータは、KR mtと同様に、R203M変異だけでもSARS-CoV-2の複製を強化するのに十分であることを示唆しています。
The KR mt enhances SARS-CoV-2 fitness during direct competition
KR mtは直接的な競争中にSARS-CoV-2の適応度を高める
We next determined if the KR mt increases SARS-CoV-2 fitness using competition assays, which offer increased sensitivity compared to individual culture experiments.
次に、個々の培養実験に比べて感度が高い競合アッセイを使用して、KR mt が SARS-CoV-2 の適応度を高めるかどうかを判定しました。
WT SARS-CoV-2 and the KR mt were directly competed by infecting Vero E6 and Calu-3 2b4 cells at a 1:1 plaque forming unit ratio.
WT SARS-CoV-2とKR mtは、1:1のプラーク形成単位比でVero E6細胞とCalu-3 2b4細胞に感染することで直接競合した。
Twenty-four hpi, total cellular RNA was harvested and the ratio of WT to KR mt genomes determined by next generation sequencing (NGS).
感染後24時間で全細胞RNAを採取し、次世代シーケンシング(NGS)によってWTとKR mtゲノムの比率を測定した。
Consistent with the kinetic data, WT outcompeted the KR mt at a ratio of ~4:1 in Vero E6 cells (Fig 1H).
運動データと一致して、WT は Vero E6 細胞で KR mt を約 4:1 の比率で打ち負かしました (図 1H)。
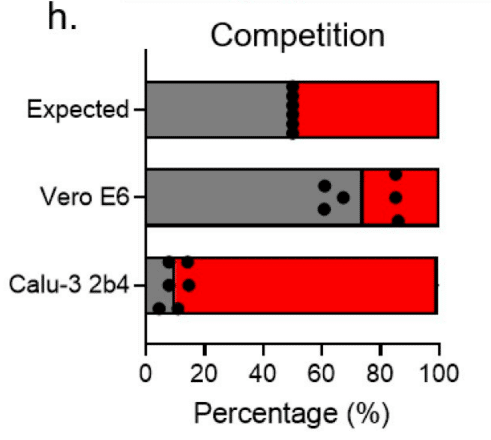
In contrast, the KR mt outcompeted WT at a ratio of ~10:1 in Calu-3 2b4 cells (Fig 1H).
対照的に、KR mt は Calu-3 2b4 細胞で WT を約 10:1 の比率で打ち負かしました (図 1H)。
These data indicate that the KR mt has a fitness advantage over WT SARS-CoV-2 in Calu-3 2b4, but not Vero E6 cells.
これらのデータは、KR mt が Calu-3 2b4 では WT SARS-CoV-2 よりも適応度が優れているが、Vero E6 細胞ではそうではないことを示しています。
The KR mt increases pathogenesis and fitness in vivo
KR mtは生体内での病原性と適応性を高める
We next determined the effects of the KR mt in vivo using a golden Syrian hamster model of SARS-CoV-2 infection.
次に、SARS-CoV-2感染のゴールデンシリアンハムスターモデルを使用して、KR mtの生体内効果を測定した。
Three- to four-week-old male hamsters underwent intranasal inoculation with either PBS (mock) or 10⁴ plaque forming units (PFU) of WT SARS-CoV-2 or the KR mt and weight loss was monitored for 10 days post infection (dpi, Fig 2A).
3~4週齢の雄ハムスターに、PBS(模擬)または10⁴プラーク形成単位(PFU)のWT SARS-CoV-2またはKR mtを鼻腔内接種し、感染後10日間(感染日、図2A)体重減少をモニタリングしました。
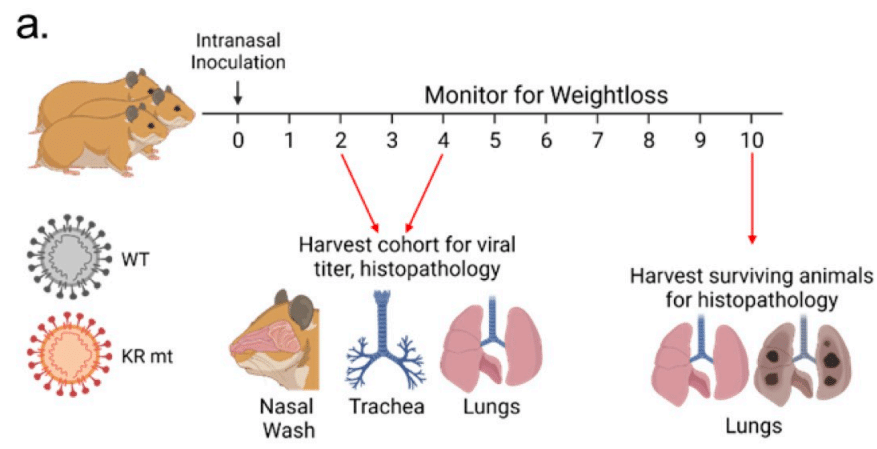
On days 2 and 4, a cohort of five animals from each group underwent nasal washing, were euthanized, and trachea and lung tissue harvested for measurement of viral loads and histopathologic analysis.
2 日目と 4 日目に、各グループから 5 匹の動物のコホートに鼻洗浄を施し、安楽死させ、気管と肺の組織を採取してウイルス量の測定と組織病理学的分析を行いました。
On day 10, surviving animals were euthanized and lung tissue harvested for histopathological analysis.
10 日目に、生き残った動物を安楽死させ、肺の組織を採取して組織病理学的分析を行いました。
Strikingly, animals infected with the KR mt had increased weight loss compared to WT throughout the experiment (Fig 2B).
驚くべきことに、KR mt に感染した動物は、実験全体を通じて WT と比較して体重減少が増加しました (図 2B)。
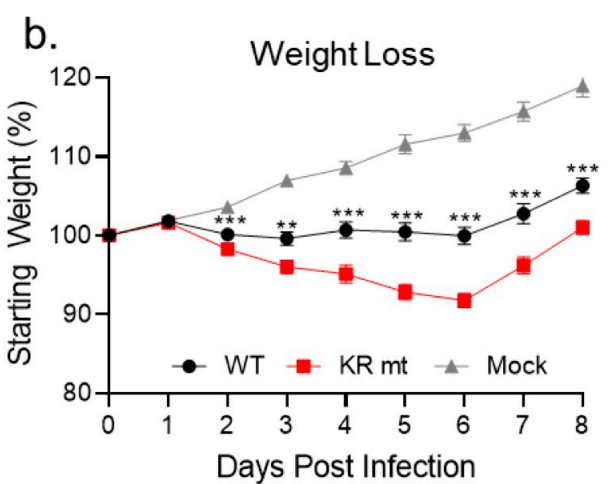
Curiously, weight loss changes did not correlate with increased viral loads, as no significant viral titer difference in the lung or trachea was observed between WT and the KR mt (Fig 2C and 2D).
興味深いことに、体重減少の変化はウイルス量の増加とは相関せず、WT と KR mt の間で肺または気管のウイルス力価に有意な差は見られませんでした (図 2C および 2D)。
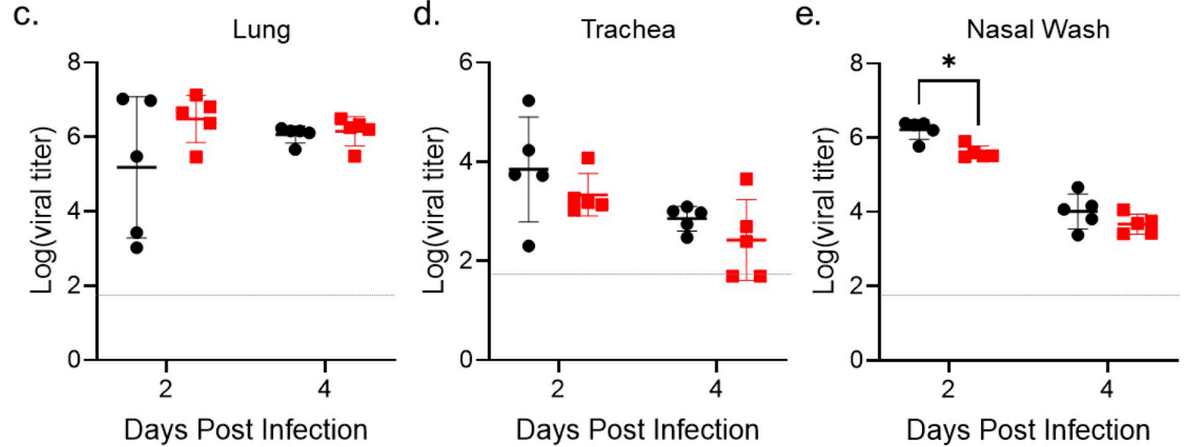
Furthermore, the KR mt caused a small, but significant, decrease in titer in nasal washes on day 2, but not day 4 (Fig 2E).
さらに、KR mt は 2 日目に鼻洗浄液中の力価をわずかながらも有意に低下させたが、4 日目には低下させなかった (図 2E)。
Contrasting the titer data, histopathologic analysis of lungs revealed that the KR mt had more severe lesions compared to WT SARS-CoV-2 (Fig 3).
力価データと対照的に、肺の組織病理学的分析により、KR mt は WT SARS-CoV-2 と比較してより重篤な病変を有することが明らかになりました (図 3)。
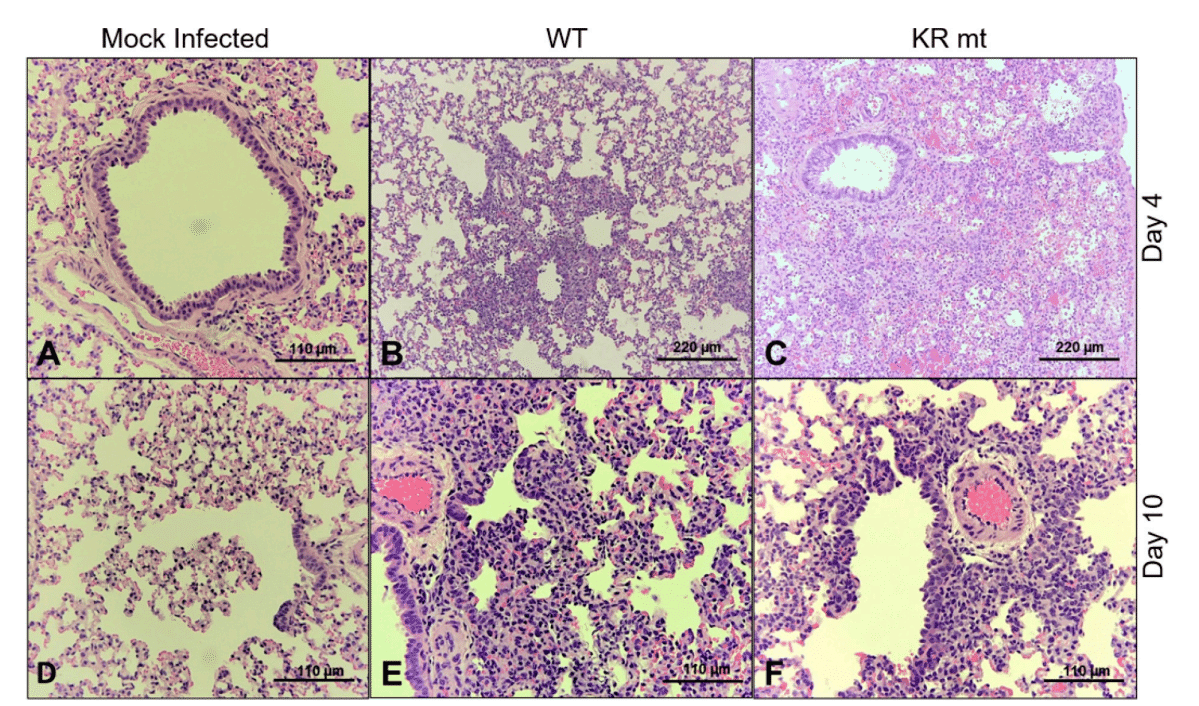
Compared to mock (Fig 3A), both WT and the KR mt had bronchiolitis and interstitial pneumonia; however, larger and more diffuse pulmonary lesions were observed in the KR mt on day 4 (Fig 3B and 3C).
モック (図 3A) と比較すると、WT と KR mt の両方に細気管支炎と間質性肺炎が見られましたが、4 日目に KR mt ではより大きく、より拡散した肺病変が観察されました (図 3B および 3C)。
In addition, the KR mt had cytopathic alveolar pneumocytes and alveoli containing both mononuclear cells and red blood cells (Fig 3C).
さらに、KR mt には細胞変性した肺胞細胞と、単核細胞と赤血球の両方を含む肺胞がありました (図 3C)。
By day 10, both WT and the KR mt showed signs of recovery, but maintained interstitial pneumonia adjacent to the bronchi absent in mock-infected animals (Fig 3D–3F).
10 日目までに、WT と KR mt の両方が回復の兆候を示しましたが、模擬感染動物には見られなかった気管支に隣接する間質性肺炎が維持されました (図 3D~3F)。
Notably, the KR mt had evidence of cytopathic effect in bronchioles, perivascular edema, and immune infiltration of the endothelium.
特に、KR mt には細気管支における細胞変性効果、血管周囲の浮腫、および内皮への免疫浸潤の証拠がありました。
Together, these data demonstrate that the KR mt increases disease following SARS-CoV-2 infection in vivo.
まとめると、これらのデータは、KR mt が体内での SARS-CoV-2 感染後に疾患を増加させることを示しています。
We next evaluated the KR mt’s effects on SARS-CoV-2 fitness and transmission in vivo.
次に、KR mt が SARS-CoV-2 の適応度と体内での伝染に及ぼす影響を評価しました。
Singly housed three- to four-week-old male donor hamsters were intranasally inoculated with 10⁴ PFU of WT SARS-CoV-2 and the KR mt at a ratio of 1:1 (Fig 4A).
3~4 週齢のオスのドナーハムスターを 1 匹ずつ飼育し、10⁴ PFU の WT SARS-CoV-2 と KR mt を 1:1 の割合で鼻腔内接種しました (図 4A)。
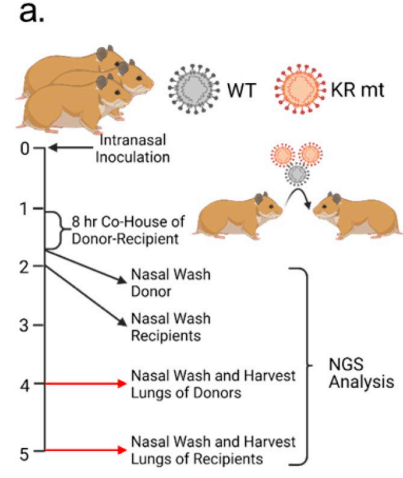
On day 1 of infection, each donor hamster was co-housed with a recipient for 8 hours to allow transmission.
感染1日目、各ドナーハムスターは、感染を許すために8時間、レシピエントと同居した。
Hamsters were then separated and SARS-CoV-2 present in the nasal cavities of the donors sampled by nasal wash.
その後、ハムスターは分離され、ドナーの鼻腔に存在するSARS-CoV-2が鼻洗浄によりサンプル採取された。
On day 2, each recipient hamster underwent nasal washing to sample transmitted SARS-CoV-2.
2日目に、各レシピエントハムスターは鼻洗浄を受け、感染したSARS-CoV-2を採取した。
Donor and recipient hamsters then underwent nasal washing and harvesting of lung tissue on days 4 and 5, respectively, and the ratio of WT to KR mt in all samples was determined by NGS.
ドナーおよびレシピエントのハムスターは、それぞれ4日目と5日目に鼻洗浄と肺組織の採取を受け、すべてのサンプルにおけるWTとKR mtの比率がNGSによって決定された。
[14]. Neither WT nor the KR mt consistently dominated in the donor washes on day 1; similarly, the day 2 nasal washes from the recipients showed no distinct advantage between KR mt and WT for transmission (Fig 4B and 4C).
1日目のドナー洗浄液では、WTもKR mtも一貫して優勢ではありませんでした。同様に、2日目のレシピエントの鼻洗浄液でも、KR mtとWTの間に感染伝播に関する明確な優位性は見られませんでした(図4Bおよび4C)。
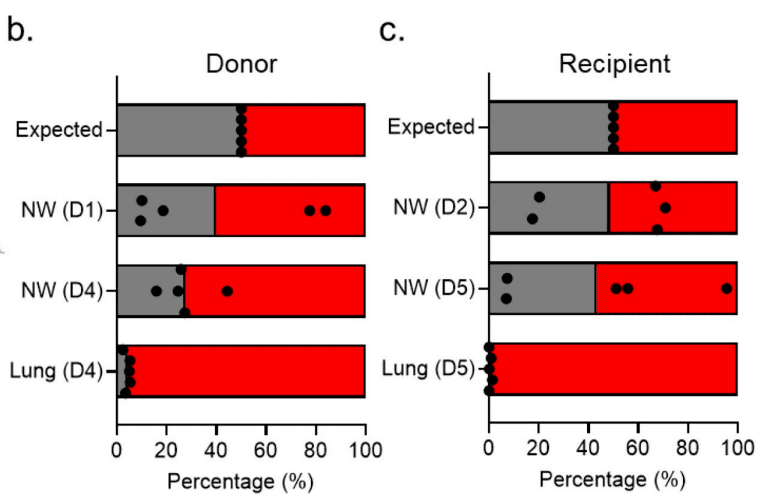
However, at day 4 and 5 in the donor and recipient, respectively, the KR mt was slightly more predominant in the nasal wash (Fig 4B and 4C).
しかし、4日目と5日目には、ドナーとレシピエントでそれぞれKR mtが鼻洗浄液でわずかに優勢でした(図4Bおよび4C)。
Notably, the KR mt dominated the SARS-CoV-2 population found in lungs of both donor and recipient animals on days 4 and 5 (Fig 4B and 4C).
注目すべきことに、4日目と5日目には、ドナーとレシピエントの動物の両方の肺で見つかったSARS-CoV-2集団でKR mtが優勢でした(図4Bおよび4C)。
Together, these results suggest that the KR mt outcompetes WT in vivo independent of transmission.
まとめると、これらの結果は、感染伝播とは無関係に、KR mtが生体内でWTに勝つことを示唆しています。
The KR mt increases viral RNA and antigen levels
KR mtはウイルスRNAと抗原レベルを増加させる
The CoV N protein has previously been shown to play a role in the transcription of viral RNA.
CoV Nタンパク質は、これまでウイルスRNAの転写に関与していることが示されています。
To evaluate changes in viral RNA levels during infection with the KR mt, we performed RT-qPCR to measure levels of SARS-CoV-2 transcripts following infection of Calu-3 2b4 cells (S4 Fig).
KR mt による感染中のウイルス RNA レベルの変化を評価するために、Calu-3 2b4 細胞に感染した後の SARS-CoV-2 転写産物のレベルを測定する RT-qPCR を実施しました (S4 図)。
Compared to WT infected cells, the KR mt had a >32-fold increase in levels of all viral transcripts, demonstrating a broad increase in SARS-CoV-2 RNA levels (Fig 5A).
WT 感染細胞と比較すると、KR mt ではすべてのウイルス転写産物のレベルが 32 倍以上増加し、SARS-CoV-2 RNA レベルが幅広く増加していることが示されました (図 5A)。
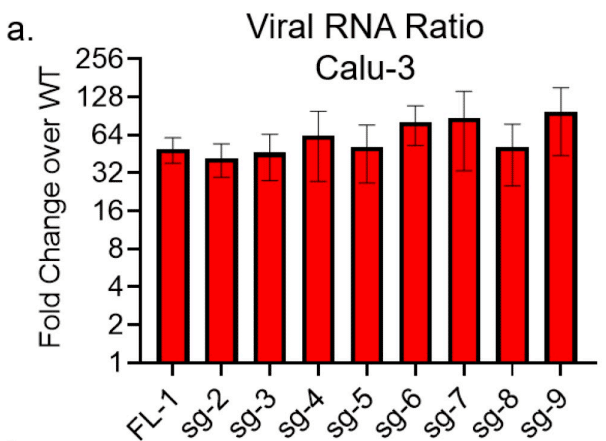
This finding is not surprising considering the increased viral titer observed in Calu-3 2b4 cells (Fig 1G).
Calu-3 2b4 細胞で観察されたウイルス力価の増加を考慮すると、この結果は驚くべきものではありません (図 1G)。
We subsequently examined the levels of full-length viral RNA in the lungs of infected hamsters (Fig 5B), finding a significant increase at 2- and 4- dpi.
その後、感染ハムスターの肺における全長ウイルスRNAのレベルを調べたところ(図5B)、感染後2日目と4日目に有意な増加が見られました。
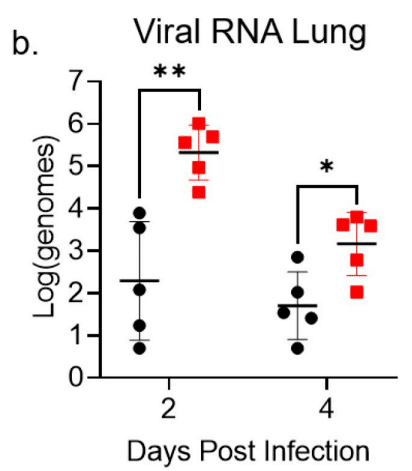
In contrast, the virus lung titer at both time points had no significant difference (Fig 2C), indicating that the KR mt increases the levels of viral RNA despite not increasing titer.
対照的に、両時点でのウイルス肺力価には有意差がありませんでした(図2C)。これは、KR mtが力価を増加させないにもかかわらず、ウイルスRNAのレベルを増加させることを示しています。
Further extending our analysis, we explored in vivo SARS-CoV-2 N antigen staining in the lungs of infected animals (Fig 5C).
さらに分析を拡張し、感染動物の肺における生体内 SARS-CoV-2 N 抗原染色を調査しました (図 5C)。
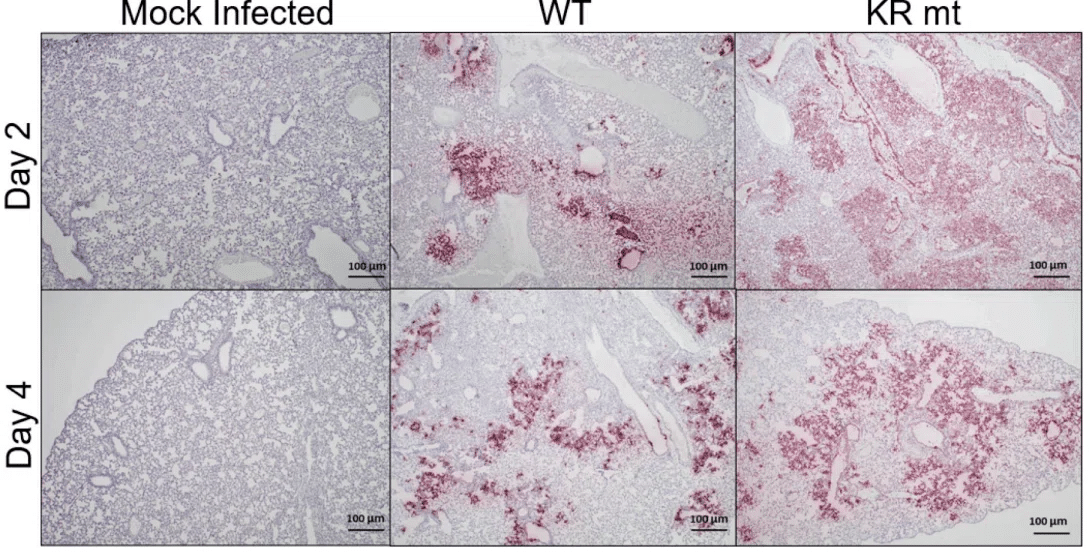
KR mt infected animals showed increased viral antigen staining and substantially larger lesion size compared to WT.
KR mt 感染動物は、WT と比較してウイルス抗原染色が増加し、病変サイズが大幅に大きくなりました。
Additionally, while lesions in WT infected animals were mostly limited to cells adjacent to the airways, KR mt infected animals showed staining throughout the parenchyma.
さらに、WT 感染動物の病変は主に気道に隣接する細胞に限定されていたのに対し、KR mt 感染動物では実質全体に染色が見られました。
Together, these data indicate despite having no effect on lung titer, the KR mt leads to increased viral RNA accumulation and greater virus spread in the lung compared to WT SARS-CoV-2.
まとめると、これらのデータは、肺の力価には影響がないにもかかわらず、KR mt は WT SARS-CoV-2 と比較してウイルス RNA の蓄積を増加させ、肺でのウイルス拡散を拡大することを示しています。
The KR mt increases phosphorylation of SARS-CoV-2 N
KR mtはSARS-CoV-2 Nのリン酸化を増加させる
Having confirmed a role in viral RNA transcription, we next considered how mutations in residues 203–205 of nucleocapsid’s SR domain might provide an advantage for SARS-CoV-2.
ウイルスRNA転写における役割を確認した後、次に、ヌクレオカプシドのSRドメインの残基203〜205の変異がSARS-CoV-2にどのような利点をもたらすかを検討しました。
Nsp3, the multi-faceted viral protease, interacts with the SR domain to increase viral transcription.
多面的なウイルスプロテアーゼである Nsp3 は、SR ドメインと相互作用してウイルスの転写を増加させます。
Importantly, this interaction is governed by phosphorylation of the SR domain, which is targeted by the SRPK, GSK-3, and Cdk1 kinases.
重要なのは、この相互作用は SR ドメインのリン酸化によって制御され、SRPK、GSK-3、および Cdk1 キナーゼによって標的とされることです。
Given the proximity to key priming residues required for GSK-3 mediated phosphorylation (Fig 6D), we hypothesized that the KR mt alters nucleocapsid phosphorylation.
GSK-3 媒介リン酸化に必要な主要なプライミング残基に近いこと (図 6D) から、KR mt がヌクレオカプシドのリン酸化を変化させるという仮説を立てました。
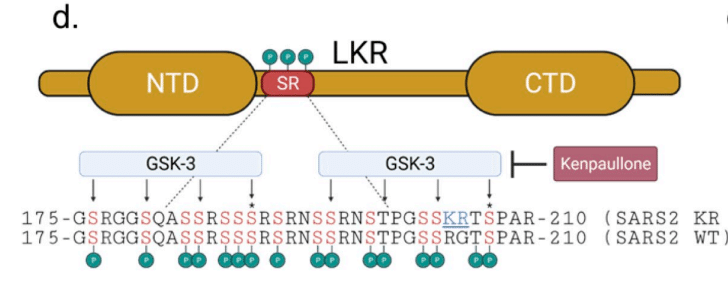
To overcome the lack of phospho-specific antibodies for nucleocapsid, we used phosphate-affinity SDS-PAGE (PA SDS-PAGE).
ヌクレオカプシドに対するリン酸化特異的抗体の不足を克服するために、リン酸アフィニティー SDS-PAGE (PA SDS-PAGE) を使用しました。
PA SDS-PAGE utilizes a divalent Zn2+ compound (Phos-Tag) within acrylamide gels that selectively binds to phosphorylated serine, threonine, and tyrosine residues; the bound Zn2+ decreases electrophoretic mobility of a protein proportionally with the number of phosphorylated amino acids.
PA SDS-PAGEは、リン酸化されたセリン、スレオニン、チロシン残基に選択的に結合するアクリルアミドゲル内の二価Zn2+化合物(Phos-Tag)を使用します。結合したZn2+は、リン酸化アミノ酸の数に比例してタンパク質の電気泳動移動度を低下させます。
Importantly, if a protein exhibits multiple phosphorylation states, this will cause a laddering effect, with each phospho-species appearing as a distinct band (Fig 6A).
重要なのは、タンパク質が複数のリン酸化状態を示す場合、ラダー効果が発生し、各リン酸化種が明確なバンドとして現れることです (図 6A)。
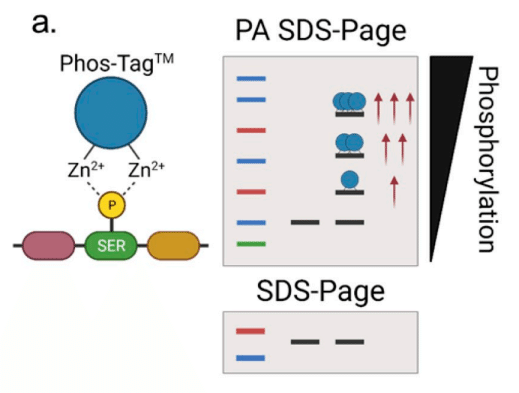
To assess the KR mt’s effects on N-phosphorylation, we infected Calu-3 2b4 cells at a MOI of 0.01 and harvested whole cell lysates 48 hpi.
KR mt の N リン酸化への影響を評価するために、Calu-3 2b4 細胞を MOI 0.01 で感染させ、48 hpi で全細胞溶解物を採取しました。
Lysates then underwent PA SDS-PAGE followed by western blotting with an N-specific antibody.
溶解物は PA SDS-PAGE にかけ、続いて N 特異的抗体でウェスタンブロッティングを行いました。
When analyzed by PA SDS-PAGE, WT SARS-CoV-2 displayed a two-band pattern consisting of a faint upper and prominent lower band, corresponding to a highly phosphorylated and a less phosphorylated species, respectively (Fig 6B, lane 1).
PA SDS-PAGE で分析すると、WT SARS-CoV-2 は、それぞれ高度にリン酸化されている種とリン酸化されていない種に対応する、かすかな上部バンドと目立つ下部バンドからなる 2 つのバンド パターンを示しました (図 6B、レーン 1)。
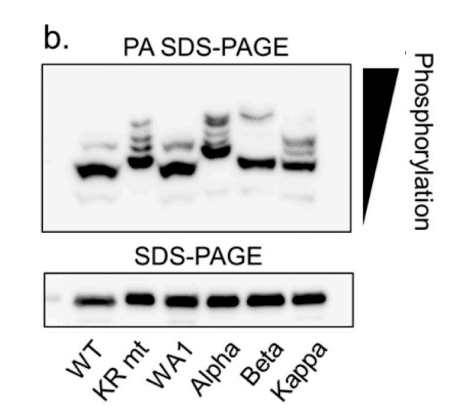
In contrast, the KR mt displayed four dark bands of progressively slower mobility, indicating a substantially different phosphorylation pattern (Fig 6B, lane 2).
対照的に、KR mt は徐々に移動が遅くなる 4 つの暗いバンドを示し、リン酸化パターンが大幅に異なることを示しています (図 6B、レーン 2)。
Importantly, all four bands migrated more slowly than the prominent WT band, indicating an overall increase in phosphorylation in the KR mt, which corresponds to increased virus replication (Fig 1G).
重要なのは、4 つのバンドすべてが顕著な WT バンドよりもゆっくりと移動したことです。 これは、KR mt のリン酸化が全体的に増加していることを示しており、これはウイルスの複製の増加に相当します (図 1G)。
We next examined N phosphorylation in Vero E6 cells; cells were infected at a MOI of 0.01 and whole cell lysates taken 24 hpi.
次に、Vero E6 細胞における N リン酸化を調べました。細胞は MOI 0.01 で感染し、24 hpi で全細胞溶解物を採取しました。
In Vero E6 cells, WT SARS-CoV-2 exhibited 2-bands of equal strength, indicating a relative increase in phosphorylation compared to Calu-3 2b4 cells (Fig 6C, lane 1).
Vero E6 細胞では、WT SARS-CoV-2 は同等の強度の 2 つのバンドを示し、Calu-3 2b4 細胞と比較してリン酸化が相対的に増加していることを示しています (図 6C、レーン 1)。
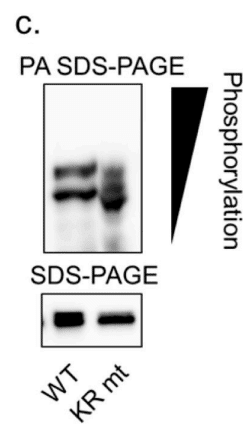
In contrast, the KR mt displayed 3 dark bands with faster mobility relative to WT, indicating a decrease in overall phosphorylation (Fig 6C, lane 2).
対照的に、KR mt は WT と比較して移動速度が速い 3 つの暗いバンドを示し、全体的なリン酸化の減少を示しています (図 6C、レーン 2)。
This reduced phosphorylation corresponds with the replication attenuation seen in this cell type (Fig 1F).
このリン酸化の減少は、この細胞タイプで見られる複製減衰と一致しています (図 1F)。
Together, these data indicate that the relative level of SARS-CoV-2 nucleocapsid phosphorylation plays a role in virus replication.
これらのデータを合わせると、SARS-CoV-2 ヌクレオカプシドのリン酸化の相対レベルがウイルスの複製に役割を果たしていることがわかります。
Given the KR mt’s effects, we next determined if SARS-CoV-2 variants had altered N- phosphorylation.
KR mt の効果を考慮して、次に SARS-CoV-2 変異体が N リン酸化を変化させたかどうかを判断しました。
Calu-3 2b4 cells were infected at a MOI of 0.01 with WA-1 or the alpha, beta, or kappa variants and whole cell lysates harvested at 48 hpi.
Calu-3 2b4 細胞を WA-1 またはアルファ、ベータ、またはカッパ変異体に MOI 0.01 で感染させ、48 hpi で全細胞溶解物を採取しました。
When analyzed by PA SDS-PAGE, WA-1 had a two-band pattern similar to WT SARS-CoV-2, while the alpha variant displayed a four-band pattern with slower mobility similar to that of the KR mt (Fig 6B, lanes 3–4).
PA SDS-PAGEで分析したところ、WA-1はWT SARS-CoV-2に似た2バンドパターンを示しましたが、アルファ変異体はKR mtに似たより遅い移動度を持つ4バンドパターンを示しました(図6B、レーン3〜4)。
Interestingly, the mobilities of the alpha variant bands were decreased compared to the KR mt indicating an even higher level of phosphorylation, potentially due to the alpha variant’s additional nucleocapsid mutations at D3L and S235F.
興味深いことに、アルファ変異体のバンドの移動度はKR mtと比較して低下しており、さらに高いレベルのリン酸化を示している可能性があります。これは、アルファ変異体のD3LとS235Fでの追加のヌクレオカプシド変異によるものと考えられます。
While both the beta (T205I) and kappa (R203M) variants also displayed slower electrophoretic mobility compared to WA-1, the beta variant displayed a two-band pattern reminiscent of WA-1 while kappa displayed a laddered pattern similar to the KR mt (Fig 6B, lanes 5–6).
ベータ(T205I)およびカッパ(R203M)変異体もWA-1と比較して電気泳動移動度が遅いことが示されましたが、ベータ変異体はWA-1を彷彿とさせる2つのバンドパターンを示し、カッパはKR mtに似たラダーパターンを示しました(図6B、レーン5〜6)。
Together, these data suggest variant mutations at residues 203–205 result in increased N phosphorylation.
これらのデータを合わせると、残基203〜205での変異体変異によりNリン酸化が増加することがわかります。
The KR mt does not alter phosphorylation of virion-associated N
KR mtはウイルス粒子関連Nのリン酸化を変化させない
While CoV N proteins are hyperphosphorylated intracellularly, they are believed to lack phosphorylation within the mature virion.
CoV Nタンパク質は細胞内で過剰にリン酸化されているが、成熟したウイルス粒子内ではリン酸化されていないと考えられている。
Nevertheless, given the ability of the KR mt to augment phosphorylation, we were curious if it influenced virion-associated SARS-CoV-2 N.
それでも、KR mt がリン酸化を増強する能力があることを考えると、それがウイルス粒子に関連する SARS-CoV-2 N に影響を与えるかどうか興味がありました。
To examine this, Calu-3 2b4 cells were infected with WT SARS-CoV-2 or the KR mt.
これを調べるために、Calu-3 2b4 細胞を WT SARS-CoV-2 または KR mt に感染させました。
48 hpi, Viral supernatants were taken, clarified, and virions pelleted on a 20% sucrose cushion by ultracentrifugation.
48 hpi、ウイルス上清を採取して清澄化し、20% ショ糖クッション上で超遠心分離によりウイルス粒子をペレット化しました。
Protein recovered from the pellets was then analyzed by PA SDS-PAGE followed by western blotting with an N-specific antibody.
ペレットから回収したタンパク質は、PA SDS-PAGE で分析し、その後 N 特異的抗体でウェスタンブロッティングを行いました。
Curiously, for both WT and the KR mt, a light upper and dark lower band were detected, indicating some level of N phosphorylation is present in the SARS-CoV-2 virion, albeit at a lower level than intracellular N (S5 Fig).
興味深いことに、WT と KR mt の両方で、上部に明るいバンド、下部に暗いバンドが検出されました。これは、SARS-CoV-2 ウイルス粒子に、細胞内 N よりは低いレベルですが、ある程度の N リン酸化が存在することを示しています (S5 図)。
However, the KR mt had no effect on the banding pattern, indicating the KR mt does not affect phosphorylation of mature virions.
ただし、KR mt はバンドパターンに影響を与えず、KR mt は成熟ウイルス粒子のリン酸化には影響を及ぼさないことを示しています。
The KR mt is more resistant to GSK-3 inhibition
KR mtはGSK-3阻害に対してより耐性がある
Our results indicate that changes in N phosphorylation correlate with differences in virus replication; thus, we sought to modulate N phosphorylation using kinase inhibitors.
我々の結果は、Nリン酸化の変化がウイルス複製の違いと相関していることを示している; したがって、我々はキナーゼ阻害剤を使用してNリン酸化を調節しようとした。
Prior work has identified two consensus sites for GSK-3 phosphorylation within the SR domain and inhibition of GSK-3 has been shown to reduce SARS-CoV-2 replication (Fig 6D).
以前の研究で、SRドメイン内のGSK-3リン酸化の2つのコンセンサス部位が特定されており、GSK-3の阻害はSARS-CoV-2の複製を減らすことが示されている(図6D)。
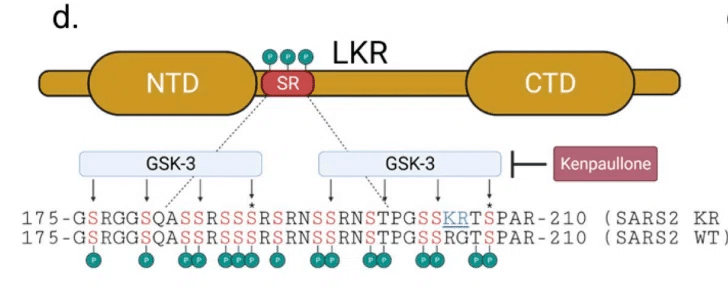
Importantly, the KR mt is proximal to the priming residue of the C-terminus GSK-3 consensus site, suggesting it may impact GSK-3 mediated N phosphorylation.
重要なのは、KR mt が C 末端 GSK-3 コンセンサス部位のプライミング残基に近接していることです。 これは、KR mt が GSK-3 を介した N リン酸化に影響を与える可能性があることを示唆しています。
Therefore, we examined the impact of GSK-3 inhibition on both WT and the KR mt.
そのため、WT と KR mt の両方に対する GSK-3 阻害の影響を調べました。
Using kenpaullone, a GSK-3 inhibitor, we showed a dose dependent inhibitory effect on both WT and KR mt titer at 48 hpi (Fig 6E).
GSK-3 阻害剤であるケンパウロンを使用して、48 hpi で WT と KR mt の両方の力価に対する用量依存的な阻害効果を示しました (図 6E)。
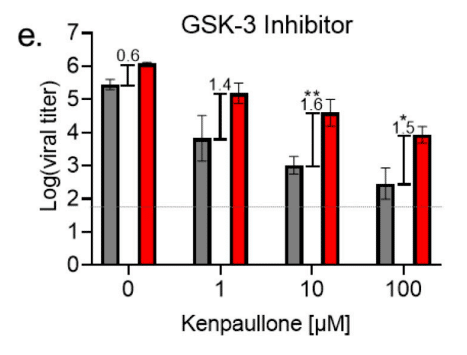
Importantly, GSK-3 inhibition had a greater impact on WT, significantly increasing the mean titer difference between WT and the KR mt from ~4-fold (0.6 log-titer) to ~38-fold (1.6 log-titer) at 10 μM kenpaullone.
重要なのは、GSK-3 阻害が WT に大きな影響を与え、10 μM ケンパウロンで WT と KR mt の平均力価差が約 4 倍 (0.6 ログ力価) から約 38 倍 (1.6 ログ力価) に大幅に増加したことです。
This suggests that the KR mt is more resistant to GSK-3 inhibition, and that the change at position 203–204 increases affinity of the KR mt for GSK-3.
これは、KR mt が GSK-3 阻害に対してより耐性があり、位置 203-204 の変化によって KR mt の GSK-3 に対する親和性が増加することを示唆しています。
An alanine double substitution mimics the KR mt
アラニン二重置換はKR mtを模倣する
Given that variant mutations at residues 203–205 are diverse in sequence, and the KR and R203M mutants phenocopy each other, we assessed the importance of the specific R→K and G→R mutations to the KR mt’s enhancement of infection.
残基 203~205 の変異体の変異は配列が多様であり、KR および R203M 変異体は互いに表現型を模倣していることから、特定の R→K および G→R 変異が KR mt の感染増強に及ぼす重要性を評価しました。
To do so, we made a R203A+G204A double alanine substitution mutant (AA mt) in the WA-1 mNG background (S6 Fig).
そのために、WA-1 mNG バックグラウンドで R203A+G204A 二重アラニン置換変異体 (AA mt) を作成しました (S6 図)。
After recovery of recombinant SARS-CoV-2, we assessed replication in Vero E6 and Calu-3 2b4 cells.
組み換えSARS-CoV-2の回収後、Vero E6およびCalu-3 2b4細胞での複製を評価しました。
In contrast with the KR mt, the AA mt had no significant effect on titers in Vero E6 cells (Fig 7A).
KR mtとは対照的に、AA mtはVero E6細胞での力価に有意な影響を及ぼしませんでした(図7A)。
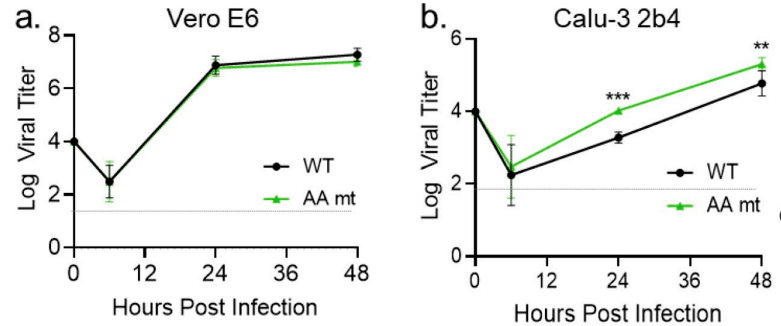
Nevertheless, the AA mt increased viral titers over WT in Calu-3 2b4 cells throughout infection, mimicking the augmented replication of the KR mt (Fig 7B).
それにもかかわらず、AA mtは感染中ずっとCalu-3 2b4細胞でWTよりもウイルス力価を高め、KR mtの増強された複製を模倣しました(図7B)。
We next tested the fitness of the AA mt by direct competition with WT SARS-CoV-2.
次に、WT SARS-CoV-2との直接競合によるAA mtの適応度をテストしました。
Vero E6 and Calu-3 2b4 cells were infected with WT and the AA mt at a 1:1 ratio, whole cell RNA harvested 24 hpi, and the ratio of WT to AA mt determined by NGS.
Vero E6およびCalu-3 2b4細胞をWTとAA mtに1:1の比率で感染させ、全細胞RNAを24hpiで採取し、WTとAA mtの比率をNGSで決定しました。
In Vero E6 cells, the AA mt had a small but consistent fitness advantage over WT (Fig 7C).
Vero E6細胞では、AA mtはWTに対してわずかですが一貫した適応度優位性を示しました(図7C)。
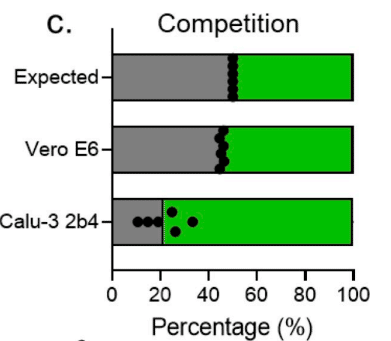
In contrast, the AA mt outcompeted WT with a ~5:1 ratio during infection of Calu-3 2b4 cells (Fig 7C).
対照的に、Calu-3 2b4 細胞の感染中、AA mt は WT を約 5:1 の比率で打ち負かしました (図 7C)。
Overall, the similarities in replication and fitness between the KR, R203M, and AA mts in Calu-3 2b4 cells suggest that the KR mt enhances infection primarily by ablating the ancestral ‘RG’ motif.
全体的に、Calu-3 2b4 細胞における KR、R203M、および AA mt 間の複製と適応度の類似性は、KR mt が主に祖先の「RG」モチーフを除去することによって感染を強化することを示唆しています。
Given that the AA mt mimicked the KR mt’s enhancement of in vitro infection, we next determined if they employed the same mechanism.
AA mt が KR mt の in vitro 感染の増強を模倣したことから、次に、それらが同じメカニズムを採用しているかどうかを判断しました。
Using RT-qPCR, we examined viral RNA expression in the AA mt (Fig 7D).
RT-qPCR を使用して、AA mt でのウイルス RNA 発現を調べました (図 7D)。
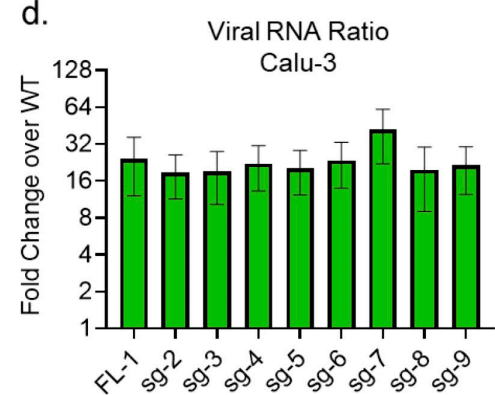
Consistent with the KR mt, at 24 hpi the AA mt increased viral transcript levels compared to WT in Calu-3 2b4 cells, although the magnitude of change in the AA mt was less than the KR mt (16 fold vs 32 fold).
KR mtと一致して、感染後24時間で、Calu-3 2b4細胞では、WTと比較してAA mtのウイルス転写レベルが増加しましたが、AA mtの変化の程度はKR mtより小さかったです(16倍対32倍)。
We next examined changes in N phosphorylation.
次に、Nリン酸化の変化を調べました。
Calu-3 2b4 cells were infected at a MOI of 0.01 with WT SARS-CoV-2 or the AA mt and whole cell lysates were harvested 48 hpi.
Calu-3 2b4細胞を、WT SARS-CoV-2またはAA mtでMOI 0.01で感染させ、48 hpiで全細胞溶解物を採取しました。
Lysates then underwent PA SDS-PAGE, followed by Western blotting for nucleocapsid.
次に、溶解物を PA SDS-PAGE にかけ、続いてヌクレオカプシドのウェスタンブロッティングを行いました。
Interestingly, the AA mt exhibited several dark bands with laddered mobility absent in WT, suggesting augmented phosphorylation similar to the KR mt.
興味深いことに、AA mt は、WT には見られないラダー状の移動性を持ついくつかの暗いバンドを示しました。 これは、KR mt と同様にリン酸化が増強されていることを示しています。
However, contrasting with the KR mt, in the AA mt the electrophoretic mobility of the lowest band was similar to WT (Fig 7E).
しかし、KR mt とは対照的に、AA mt では最も低いバンドの電気泳動移動度は WT と同様でした (図 7E)。
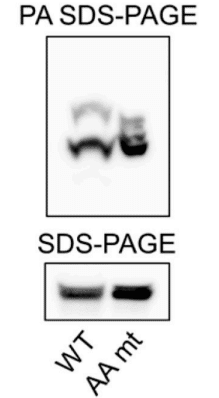
These data suggest that while the KR and AA mts both alter nucleocapsid phosphorylation, their effects are not identical.
これらのデータは、KR mt と AA mt はどちらもヌクレオカプシドのリン酸化を変化させますが、その効果は同一ではないことを示唆しています。
Nevertheless, the changes in phosphorylation induced by the KR and AA mt are both sufficient to enhance SARS-CoV-2 replication.
それにもかかわらず、KR mt と AA mt によって誘発されるリン酸化の変化はどちらも SARS-CoV-2 の複製を促進するのに十分です。
Finally, we examined GSK-3 inhibition comparing WT and AA mt (Fig 7F).
最後に、WT と AA mt を比較して GSK-3 阻害を調べました (図 7F)。
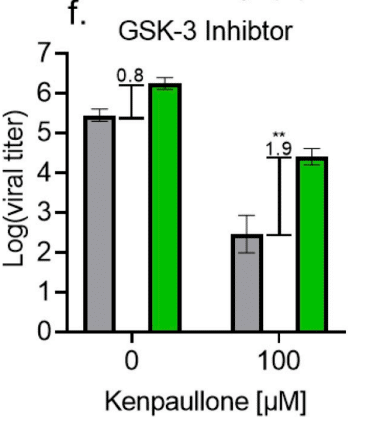
Similar to the KR mt, the AA mt was less sensitive to GSK-3 inhibition than WT, suggesting that disrupting the original RG motif resulted in increased affinity for the kinase.
KR mt と同様に、AA mt は WT よりも GSK-3 阻害に対する感受性が低かったです。 これは、元の RG モチーフを破壊するとキナーゼに対する親和性が増したことを示しています。
Overall, these data demonstrate that mutations at positions 203–204 disrupt an ancestral motif that interferes with GSK-3 kinase activity.
全体として、これらのデータは、位置 203~204 の変異が GSK-3 キナーゼの活性を妨げる祖先モチーフを破壊することを示しています。
Discussion
In this manuscript, we investigate a highly variable motif in SARS-CoV-2 nucleocapsid by characterizing the effects of the R203K+G204R double substitution mutation (KR mt), present in the alpha and omicron variants.
本稿では、SARS-CoV-2 ヌクレオカプシドの非常に可変性の高いモチーフを調査し、アルファおよびオミクロン変異体に存在する R203K+G204R 二重置換変異 (KR mt) の効果を特徴付けます。
Inserting the KR mt in the WA-1 background demonstrated that the KR mt alone is sufficient to increase titer and fitness in respiratory cells.
WA-1 バックグラウンドに KR mt を挿入すると、KR mt だけで呼吸器細胞における力価と適応度を高めるのに十分であることが実証されました。
Inserting the R203M mutation from the delta variant in WA-1 similarly enhanced virus replication.
デルタ変異体の R203M 変異を WA-1 に挿入すると、同様にウイルスの複製が強化されました。
The KR mt is also sufficient to increase disease in infected hamsters and enhance fitness in the lung during direct competition studies.
KR mt は、感染したハムスターの疾患を増加させ、直接競合研究中に肺の適応度を高めるのにも十分です。
Importantly, we demonstrate that the KR mt augmented nucleocapsid phosphorylation, which correlates with increased virus replication.
重要なのは、KR mt がヌクレオカプシドのリン酸化を増強し、それがウイルス複製の増加と相関していることを実証したことです。
We also demonstrate that the KR mt is resistant to inhibition of the GSK-3 kinase, mechanistically linking N phosphorylation with phenotypic changes.
また、KR mt は GSK-3 キナーゼの阻害に耐性があり、N リン酸化と表現型の変化がメカニズム的に関連していることも実証しました。
SARS-CoV-2 variants harboring analogous mutations in the 203–204 motif also exhibit augmented nucleocapsid phosphorylation, suggesting that increasing N phosphorylation is a common mechanism driving variant emergence.
203-204 モチーフに類似の変異を持つ SARS-CoV-2 変異体もヌクレオカプシドのリン酸化が増強しており、N リン酸化の増加が変異体出現の一般的なメカニズムであることを示唆しています。
Notably, an analogous double alanine substitution (AA mt) also showed increased replication, fitness, altered nucleocapsid phosphorylation, and similar resistance to kinase inhibition.
注目すべきことに、類似の二重アラニン置換 (AA mt) も、複製、適応度の増加、ヌクレオカプシドのリン酸化の変化、およびキナーゼ阻害に対する同様の耐性を示しました。
Together, these data suggest that the KR mt and similar mutations enhance SARS-CoV-2 infection by increasing N phosphorylation through disruption of the ancestral “RG” motif.
これらのデータを合わせると、KR mt および類似の変異が、祖先の「RG」モチーフの破壊を通じて N リン酸化を増加させ、SARS-CoV-2 感染を強化することが示唆されます。
The KR mt occurs within the serine-rich (SR) domain of nucleocapsid, which has a complex role during SARS-CoV-2 infection.
KR mt は、SARS-CoV-2 感染中に複雑な役割を果たすヌクレオカプシドのセリンリッチ (SR) ドメイン内に存在します。
This region of N is hyper-phosphorylated intracellularly, but hypo-phosphorylated within the mature virion.
この N 領域は細胞内で過剰にリン酸化されていますが、成熟したウイルス粒子内では低リン酸化されています。
Several studies suggest that phosphorylation of the SR domain acts as a biophysical switch, regulating nucleocapsid function through phase separation.
いくつかの研究では、SRドメインのリン酸化は生物物理学的スイッチとして機能し、相分離を通じてヌクレオカプシドの機能を制御することが示唆されている。
In the proposed model, unphosphorylated nucleocapsid forms gel-like condensates with viral RNA and the SARS-CoV-2 membrane (M) protein to facilitate genome packaging and virus assembly.
提案されたモデルでは、リン酸化されていないヌクレオカプシドはウイルスRNAおよびSARS-CoV-2膜(M)タンパク質とゲル状の凝縮物を形成し、ゲノムパッケージングとウイルスの組み立てを促進します。
In contrast, phosphorylated nucleocapsid forms distinct liquid-like condensates to promote N binding to SARS-CoV-2 nsp3, G3BP1 in stress granules, and (presumably) other nucleocapsid functions.
対照的に、リン酸化ヌクレオカプシドは明確な液体状の凝縮物を形成し、SARS-CoV-2 nsp3、ストレス顆粒内のG3BP1、および(おそらく)他のヌクレオカプシド機能へのNの結合を促進します。
While not tested in the context of infection, this model is consistent with studies demonstrating interactions between N and M, G3BP1 and G3BP2 within stress granules, and phosphorylated N and nsp3 to promote the synthesis of viral RNA.
感染の文脈ではテストされていないが、このモデルは、NとM、ストレス顆粒内のG3BP1とG3BP2、およびリン酸化Nとnsp3の間の相互作用がウイルスRNAの合成を促進することを示す研究と一致している。
Within this context, the KR mt may optimize this biomolecular switch for human infection, increasing the amount of phosphorylated nucleocapsid and shifting the overall function of N during infection.
この文脈では、KR mt はヒト感染のためのこの生体分子スイッチを最適化し、リン酸化ヌクレオカプシドの量を増やし、感染中の N の全体的な機能を変化させる可能性があります。
Alternatively, the KR mt may impact the interaction between nucleocapsid and host 14-3-3 proteins which bind the SR domain in a phosphorylation dependent manner.
あるいは、KR mt は、リン酸化依存的に SR ドメインに結合するヌクレオカプシドと宿主 14-3-3 タンパク質との相互作用に影響を与える可能性がある。
This interaction is required for cytoplasmic localization of nucleocapsid in SARS-CoV.
この相互作用は、SARS-CoVの核カプシドの細胞質局在に必要である。
Notably, one 14-3-3 binding site encompasses the 203–204 motif examined in this study, suggesting that the KR mt may enhance infection by altering this interaction.
注目すべきことに、14-3-3 結合部位の 1 つは、本研究で調べた 203-204 モチーフを包含しており、KR mt がこの相互作用を変化させることで感染を増強する可能性があることを示唆しています。
Our finding that the R203M, R203K+G204R, and R203A+G204A mutations phenocopy one another suggests they enhance infection by disrupting the ancestral N sequence in the WT WA-1 strain.
R203M、R203K+G204R、および R203A+G204A 変異が互いに表現型を模倣するという私たちの発見は、WT WA-1 株の祖先 N 配列を破壊することで感染を増強することを示唆しています。
We propose a model where mutations at residues 203–204 are selected for because they eliminate the ancestral ‘RG’ sequence, which disrupts phosphorylation of the SR domain in WT SARS-CoV-2.
残基203~204の変異は祖先の「RG」配列を排除し、WT SARS-CoV-2のSRドメインのリン酸化を破壊するため選択されるというモデルを提案します。
Consistent with this model, the wild type RG sequence is present <1% of extant SARS-CoV-2 (Fig 1A), while the RG sequence is conserved in SARS2-like Bat-CoV RaTG13 and Pangolin-CoV MP789 (S7 Fig).
このモデルと一致して、野生型RG配列は現存するSARS-CoV-2の1%未満に存在し(図1A)、RG配列はSARS2類似のBat-CoV RaTG13およびPangolin-CoV MP789で保存されています(S7図)。
Further supporting this model, nucleocapsid phosphorylation of the alpha, and kappa variants (containing the KR and R203M mutations, respectively) are all enhanced relative to WA-1 (Fig 6B).
このモデルをさらに裏付けるように、αおよびκ変異体(それぞれKRおよびR203M変異を含む)のヌクレオカプシドリン酸化はすべてWA-1と比較して強化されています(図6B)。
Notably, the SARS-CoV-2 beta variant, SARS-CoV, and SARS-like Bat-CoV WIV1 contain an asparagine rather than a threonine at position 205, suggesting additional mutations in this region may impact phosphorylation of the SR domain; this idea is supported by increased N phosphorylation observed in the beta variant relative to WT.
特に、SARS-CoV-2 ベータ変異体、SARS-CoV、および SARS に似た Bat-CoV WIV1 には、位置 205 にスレオニンではなくアスパラギンが含まれています。 この領域でさらに変異があると、SR ドメインのリン酸化に影響する可能性があります; この考えは、WT と比較してベータ変異体で観察される N リン酸化の増加によって裏付けられています。
Overall, this high degree of variation suggests that this motif is under selection among these closely related viruses.
全体的に、この高い変異度は、このモチーフがこれらの近縁ウイルスの間で選択を受けていることを示唆しています。
Further studies are needed to determine how mutations in the 203–205 motif modulate infection and nucleocapsid phosphorylation in bat and other hosts, which may provide insight into the adaptation necessary for human infection.
203~205 モチーフの変異がコウモリや他の宿主における感染とヌクレオカプシドのリン酸化をどのように調節するかを決定するには、さらなる研究が必要です。これは、ヒト感染に必要な適応についての洞察を提供する可能性があります。
It is unclear why the KR mt enhances infection in Calu-3 2b4, but not Vero E6 cells.
KR mt が Calu-3 2b4 細胞では感染を増強するが、Vero E6 細胞では増強しない理由は不明です。
Our results show that while the KR mt enhances nucleocapsid phosphorylation in Calu-3 2b4 cells, it decreases phosphorylation in Vero E6 cells (Fig 6B and 6C).
我々の結果は、KR mt が Calu-3 2b4 細胞ではヌクレオカプシドのリン酸化を促進する一方で、Vero E6 細胞ではリン酸化を減少させることを示している (図 6B および 6C)。
These results suggest that cell lines may use kinases differentially, changing the dynamics of nucleocapsid phosphorylation.
これらの結果は、細胞株がキナーゼを差別的に使用し、ヌクレオカプシドのリン酸化のダイナミクスを変化させる可能性があることを示唆している。
The divergent phenotypes may reflect species or tissue specific differences between Calu-3 2b4 (human, lung) and Vero E6 (African green monkey, kidney) cells.
異なる表現型は、Calu-3 2b4(ヒト、肺)細胞とVero E6(アフリカミドリザル、腎臓)細胞間の種または組織特有の違いを反映している可能性があります。
Notably, divergent between Vero E6 and Calu3 2b4 have previously been reported.
特に、Vero E6とCalu3 2b4の相違は以前にも報告されています。
Importantly, phenotypes from Calu-3 2b4 cells have consistently matched the in vivo hamster model.
重要なのは、Calu-3 2b4 細胞の表現型が、生体内ハムスターモデルと一貫して一致していることです。
The results confirm the utility of Calu-3 2b4 cells and suggests limitations to studies using Vero E6 cells for understanding SARS-CoV-2 infection.
この結果は、Calu-3 2b4 細胞の有用性を確認し、SARS-CoV-2 感染を理解するために Vero E6 細胞を使用する研究の限界を示唆しています。
A second unanswered question is why the KR mt results in increased fitness, RNA levels, lesion size, and disease in infected hamsters without increasing lung titer.
2 つ目の未解決の疑問は、KR mt が肺力価を増加させることなく、感染ハムスターのフィットネス、RNA レベル、病変サイズ、および疾患の増加をもたらす理由です。
Several possibilities exist.
いくつかの可能性があります。
First, recent work suggests that SARS-CoV-2 is capable of direct cell-to-cell transmission in addition to ACE2-mediated extracellular entry.
まず、最近の研究では、SARS-CoV-2 は ACE2 を介した細胞外侵入に加えて、直接的な細胞間伝播が可能であることが示唆されています。
It is possible that the increase in viral found in the KR mt augments cell-to-cell transmission, enhancing infection without increasing detectable viral titer.
KR mt で見つかったウイルスの増加が細胞間感染を増強し、検出可能なウイルス力価を増加させることなく感染を増強する可能性があります。
Alternatively, the KR mt may allow the infection of additional cell types that are less permissive for infection, resulting in increased RNA levels and antigen staining without increasing titer.
あるいは、KR mt は感染に許容性が低い追加の細胞タイプの感染を可能にし、力価を増加させることなく RNA レベルと抗原染色を増加させる可能性があります。
Finally, the KR mt may provide an early kinetic advantage to SARS-CoV-2.
最後に、KR mt は SARS-CoV-2 に早期の運動学的利点をもたらす可能性があります。
In this scenario, the KR mt enhances replication early in infection, but this advantage dissipates by the time of our measurement of lung titer on day 2.
このシナリオでは、KR mt は感染初期に複製を促進しますが、この利点は 2 日目に肺力価を測定した時点では消失しています。
This hypothesis is consistent with our Calu-3 2b4 data showing greater titer differences between the KR mt and WT at 24 hpi compared to 48 hpi (Fig 1G).
この仮説は、感染後 24 hpi では KR mt と WT の力価の差が 48 hpi よりも大きいことを示す Calu-3 2b4 データと一致しています (図 1G)。
Additional studies are required to distinguish between these possibilities.
これらの可能性を区別するには、追加の研究が必要です。
Overall, in this study we establish that the KR mt enhances SARS-CoV-2 infection relative to WT, increasing viral fitness in vitro and in vivo, which along with the N501Y mutation, likely selected for the emergence of the alpha variant.
全体として、この研究では、KR mt が WT と比較して SARS-CoV-2 の感染を促進し、in vitro および in vivo でのウイルス適応度を高め、これが N501Y 変異とともにアルファ変異体の出現を選択した可能性が高いことが立証されました。
We also find that the KR mt increases nucleocapsid phosphorylation; coupled with increased resistance to GSK-3 inhibition, these results provide a molecular basis for the KR mt’s effects.
また、KR mt がヌクレオカプシドのリン酸化を増加させることもわかりました。GSK-3 阻害に対する耐性の増加と相まって、これらの結果は KR mt の効果の分子的根拠となります。
Importantly, we show that other variant mutations in this motif also increase nucleocapsid phosphorylation, which may have aided in the emergence of their respective lineages.
重要なことに、このモチーフの他の変異体変異もヌクレオカプシドのリン酸化を増加させ、それがそれぞれの系統の出現を助けた可能性があることが示されています。
When taken with the prevalence of mutations in residues 203–205 of the SR domain among circulating variants, these data suggest that mutations increasing nucleocapsid phosphorylation represent a positive selection event for SARS-CoV-2 during adaptation to human infection.
循環変異体における SR ドメインの残基 203~205 の変異の蔓延を考慮すると、これらのデータは、ヌクレオカプシドのリン酸化を増加させる変異が、ヒト感染への適応中の SARS-CoV-2 の正の選択イベントを表していることを示唆しています。
Importantly, our work highlights that mutations outside of SARS-CoV-2 spike have significant effects on infection, and must not be overlooked while characterizing mechanisms of variant emergence.
重要なのは、我々の研究が、SARS-CoV-2 スパイク以外の変異が感染に重大な影響を及ぼし、変異体出現のメカニズムを特徴付ける際に見逃してはならないことを強調していることです。
以下省略。
この記事が気に入ったらサポートをしてみませんか?