最適化されたヘパラン硫酸架橋と鎖間可動性に向けた SARS-CoV-2 スパイク三量体の進化
Evolution of SARS-CoV-2 spike trimers towards optimized heparan sulfate cross-linking and inter-chain mobility
最適化されたヘパラン硫酸架橋と鎖間可動性に向けた SARS-CoV-2 スパイク三量体の進化
Abstract:
The heparan sulfate (HS)-rich extracellular matrix (ECM) serves as an initial interaction site for the homotrimeric spike (S)-protein of SARS-CoV-2 to facilitate subsequent docking to angiotensin-converting enzyme 2 (ACE2) receptors and cellular infection.
ヘパラン硫酸(HS)を多く含む細胞外マトリックス(ECM)は、SARS-CoV-2のホモ三量体スパイク(S)タンパク質の最初の相互作用部位として機能し、その後のアンジオテンシン変換酵素2(ACE2)受容体へのドッキングと細胞感染を促進します。
Recent variants of concern (VOCs), notably Omicron, have evolved by swapping several amino acids to positively charged residues to enhance the S-protein trimer’s interaction with the negatively charged HS polysaccharide chains in the matrix.
最近の懸念される変異体(VOC)、特にオミクロンは、いくつかのアミノ酸を正に帯電した残基に交換することで進化し、マトリックス内の負に帯電したHS多糖鎖とSタンパク質三量体の相互作用を強化しました。
These increased interactions, however, may reduce Omicron’s ability to move through the HS-rich ECM to effectively find ACE2 receptors and infect cells, and raise the question of how HS-associated virus movement can be mechanistically explained.
しかし、これらの相互作用の増加により、HSを多く含むECMを通過してACE2受容体を効果的に見つけて細胞に感染するオミクロンの能力が低下する可能性があり、HS関連ウイルスの動きをメカニズム的にどのように説明できるかという疑問が生じます。
In this work, we show that Omicron S-proteins have evolved to balance HS interaction stability and dynamics, resulting in enhanced mobility on an HS-functionalized artificial matrix.
本研究では、オミクロンSタンパク質がHS相互作用の安定性とダイナミクスのバランスをとるように進化し、HS機能化人工マトリックス上での移動性が向上したことを示しています。
Both properties are achieved by the ability of Omicrons S-proteins to cross-link at least two HS chains, providing both high avidity to retain the protein inside the HS-rich matrix, and fast dynamics, thus enabling direct S-protein switching between HS chains as a prerequisite for mobility at the cell surface.
両方の特性は、オミクロンSタンパク質が少なくとも2つのHS鎖を架橋する能力によって達成され、HSに富むマトリックス内でタンパク質を保持するための高い親和性と高速ダイナミクスの両方を提供し、細胞表面での移動の前提条件としてHS鎖間で直接Sタンパク質を切り替えることを可能にします。
Optimized HS interactions can be targeted pharmaceutically, because an HS mimetic significantly suppressed surface binding and cellular infection specifically of the Omicron VOC.
最適化されたHS相互作用は、HS模倣物が特にオミクロンVOCの表面結合と細胞感染を大幅に抑制したため、薬学的に標的にすることができます。
These findings suggest a robust way to interfere with SARS-CoV-2 Omicron infection and, potentially, future variants.
これらの発見は、SARS-CoV-2オミクロン感染、および将来的には変異体を妨害する強力な方法を示唆しています。
Introduction:
Coronavirus disease (COVID-19) is caused by severe acute respiratory syndrome coronavirus 2 (SARS-CoV-2), which first emerged in the city of Wuhan, Hubei province, China, in 2019.
コロナウイルス感染症(COVID-19)は、2019年に中国湖北省武漢市で初めて発生した重症急性呼吸器症候群コロナウイルス2(SARS-CoV-2)によって引き起こされます。
Since then, SARS-CoV-2 has demonstrated unprecedented global spread and rapid evolution into new variants of concern (VOCs).
それ以来、SARS-CoV-2は前例のない世界的な広がりと、懸念される新たな変異体(VOC)への急速な進化を示してきました。
SARS-CoV-2 VOCs bind more rapidly to respiratory epithelial cells, making them more infectious.
SARS-CoV-2 VOCは呼吸器上皮細胞に素早く結合し、感染力が高まります。
They also have the ability to evade the immune response generated by previous infections or vaccinations.
また、以前の感染やワクチン接種によって生じた免疫反応を回避する能力も備えています。
The Omicron VOC is currently the dominant variant circulating worldwide, and as of January 2024, 99% of the variants circulating in the U.S. were mutations of Omicron, most commonly EG.5 (causing an estimated 24% of all COVID-19), FL 1.5.1 (accounting for 14% of all cases) (https://covid.cdc.gov/covid-data-tracker/#variant-proportions) and, more recently, the KP.2 variant.
オミクロンVOCは現在、世界中で流行している主要な変異株であり、2024年1月現在、米国で流行している変異株の99%はオミクロンの変異であり、最も一般的なのはEG.5(COVID-19全体の推定24%の原因)、FL 1.5.1(全症例の14%を占める)、そして最近ではKP.2変異株である。
An important feature acquired during the evolution of the Omicron variants is that they tend to infect the upper respiratory tract (e.g., nose and throat) more than previous variants such as Wuhan and Delta, which tended to infect the lower respiratory tract (e.g., lungs), resulting in more severe disease and higher mortality.
オミクロン変異株の進化の過程で獲得された重要な特徴は、以前の変異株である武漢やデルタよりも上気道(鼻や喉など)に感染する傾向があることです。以前の変異株は下気道(肺など)に感染する傾向があり、その結果、より重篤な疾患と高い死亡率をもたらしました。
SARS-CoV-2 possesses 24 surface spike (S) glycoprotein trimers per virion that are central to host cell binding and infection. Like the S-proteins of SARS coronavirus (SARS-CoV), SARS-CoV-2 S-proteins bind to the cell surface receptor angiotensin converting enzyme 2 (ACE2) (Figure 1a).
SARS-CoV-2 は、ウイルス粒子 1 つあたり 24 個の表面スパイク (S) 糖タンパク質三量体を有し、これが宿主細胞への結合と感染の中心となります。SARS コロナウイルス (SARS-CoV) の S タンパク質と同様に、SARS-CoV-2 の S タンパク質は細胞表面受容体アンジオテンシン変換酵素 2 (ACE2) に結合します (図 1a)。
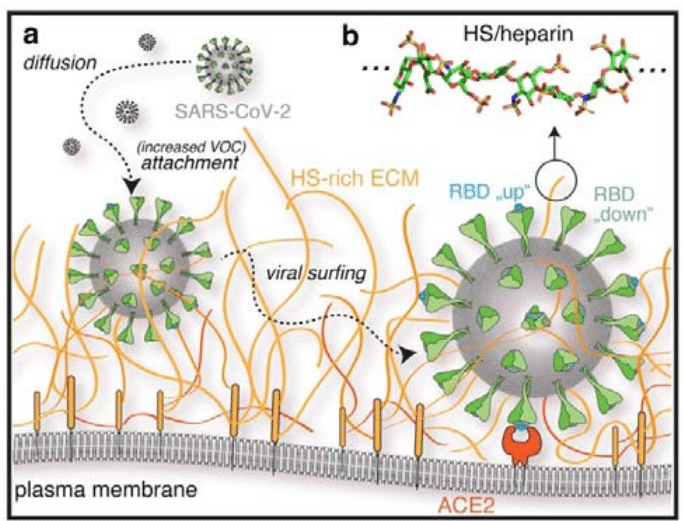
This S-protein/ACE2 interaction triggers a cascade of events leading to the fusion of cell and viral membranes, facilitating viral entry into host cells.
この S タンパク質/ACE2 相互作用により、細胞膜とウイルス膜の融合につながる一連のイベントが引き起こされ、ウイルスが宿主細胞に侵入しやすくなります。
The evolution of this S-protein function is a major determinant of viral infectivity, spread, pathogenesis, and adaptation for infection of new hosts and cell lines.
この S タンパク質機能の進化は、ウイルスの感染性、拡散、病原性、および新しい宿主や細胞株への感染への適応の主要な決定要因です。
The SARS-CoV-2 S-protein is structurally divided into S1 and S2 subunits.
SARS-CoV-2 Sタンパク質は、構造的にS1サブユニットとS2サブユニットに分かれています。
The S1 subunit contains the receptor binding domain (RBD), which is required for binding to ACE2 on the host cell, and the S2 subunit drives subsequent membrane fusion.
S1サブユニットには、宿主細胞上のACE2への結合に必要な受容体結合ドメイン(RBD)が含まれており、S2サブユニットはその後の膜融合を促進します。
Importantly, the RBD is hidden within the S1, preventing its interaction with ACE2 and avoiding detection by the immune system.
重要なのは、RBD が S1 内に隠れているため、ACE2 との相互作用を防ぎ、免疫システムによる検出を回避していることです。
To engage the ACE2 receptor, the RBD must first convert from the inaccessible conformation into an exposed conformation (Figure 1a).
ACE2 受容体と結合するには、RBD はまずアクセスできない構造から露出した構造に変換する必要があります (図 1a)。
This essential conformational change of the S-protein is a consequence of its binding to highly negatively charged heparan sulfate (HS, Figure 1b) polysaccharide chains, which are abundant components of the extracellular matrix (ECM).
S タンパク質のこの重要な構造変化は、細胞外マトリックス (ECM) の豊富な成分である、非常に負に帯電したヘパラン硫酸 (HS、図 1b) 多糖鎖への結合の結果です。
In addition to converting the RBD subunit to an accessible conformation for ACE2 binding, HS plays a second important role in SARS-CoV-2 infection by acting as a collector of the viruses at the epithelial surface (Figure 1a).
HSはRBDサブユニットをACE2が結合しやすい構造に変換するだけでなく、上皮表面でウイルスを収集する役割も果たし、SARS-CoV-2感染において2番目に重要な役割を果たします(図1a)。
Indeed, ACE2 expression levels in human epithelial cells and lung tissue are relatively low, which limits the ability of freely diffusing SARS-CoV-2 to infect cells.
実際、ヒト上皮細胞と肺組織におけるACE2発現レベルは比較的低く、自由に拡散するSARS-CoV-2が細胞に感染する能力が制限されています。
To overcome this issue, the virus binds to the HS-rich ECM and moves within it close to the epithelial cell membrane, enhancing the likelihood of interaction with ACE2 (Figure 1a).
この問題を克服するために、ウイルスはHSに富むECMに結合し、その中で上皮細胞膜の近くに移動し、ACE2との相互作用の可能性を高めます(図1a)。
Given their essential role in cellular infection, the enhanced fitness observed in many SARS-CoV-2 VOCs have therefore been attributed primarily to mutations of the S-protein that dramatically increase both ACE2 and HS binding properties (Figure 1c-e) by increasing the total formal charge of the trimeric S-protein (residues 13-1140) from + 3 (Wuhan) to + 18 and to + 24 for Delta and the Omicron B.1.1.529 subvariant, respectively.
細胞感染における重要な役割を考えると、多くのSARS-CoV-2 VOCで観察された適応度の向上は、主にSタンパク質の変異に起因すると考えられています。この変異により、三量体Sタンパク質(残基13-1140)の総形式電荷が、それぞれ+ 3(武漢)から+ 18(デルタ株)および+ 24(オミクロン株B.1.1.529)に増加し、ACE2およびHS結合特性の両方が劇的に向上します(図1c-e)。
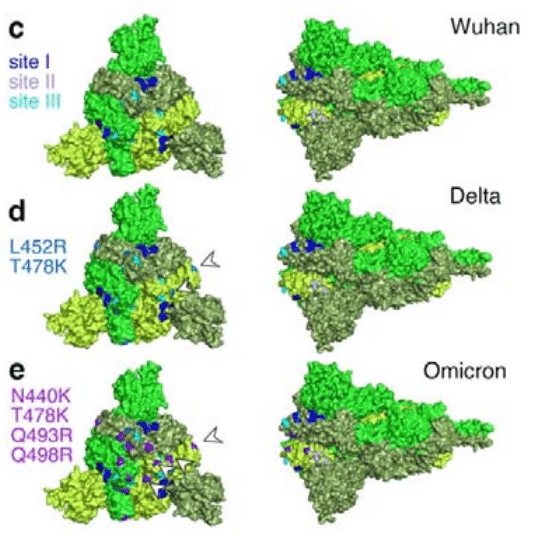
These observations suggest that more infectious variants have undergone selection for increased engagement with the negatively charged cell-surface HS.
これらの観察結果は、より感染性の高い変異体が、負に帯電した細胞表面HSとの関与を増やすための選択を受けたことを示唆している。
This evolution, however, raises an important question: Do these increased HS interactions impair the rapid movement of SARS-CoV-2 through the ECM and across cell surfaces, a process known as “viral surfing” (Figure 1a) or, do the acquired mutations enhance viral surfing in a counterintuitive manner?
しかし、この進化は重要な疑問を提起する。これらのHS相互作用の増加は、SARS-CoV-2のECMおよび細胞表面を介した迅速な移動、いわゆる「ウイルスサーフィン」を阻害するのか(図1a)、それとも、獲得した変異が直感に反する形でウイルスサーフィンを強化するのか?
If the former were true, the virus would be trapped locally and unable to move to ACE2 receptors on the cell surface.
前者が真実であれば、ウイルスは局所的に捕捉され、細胞表面の ACE2 受容体に移動することはできません。
If the latter were true, viral movement on and between cells in tissues would increase and enhance infectivity, as observed for the VOCs.
後者が真実であれば、VOC で観察されたように、組織内の細胞上および細胞間のウイルスの移動が増加し、感染性が強化されます。
Additionally, important questions include how to mechanistically explain viral surfing of HS-bound viruses and whether the additional positively charged amino acids in Delta and Omicron VOCs enhance the efficacy of strongly polyanionic soluble inhibitors to block S-protein function and cellular infection.
さらに、重要な疑問には、HS 結合ウイルスのウイルス サーフィンをメカニズム的に説明する方法と、デルタおよびオミクロン VOC の追加の正電荷アミノ酸が、S タンパク質機能および細胞感染を阻害する強力なポリアニオン性可溶性阻害剤の有効性を高めるかどうかが含まれます。
We investigated these questions by using the well-characterized evolution of SARS-CoV-2 VOCs as a model for HS-binding viruses in general.
我々は、SARS-CoV-2 VOC のよく特徴づけられた進化を、HS 結合ウイルス全般のモデルとして使用して、これらの疑問を調査しました。
To this end, we compared the interactions of isolated RBDs with sulfated oligosaccharides in silico, and the molecular properties of RBDs, trimeric S-proteins and SARS-CoV-2 viral isolates from Wuhan, Delta, and Omicron in vitro.
この目的のために、我々は、単離されたRBDと硫酸化オリゴ糖との相互作用をコンピュータ上で比較し、RBD、三量体Sタンパク質、武漢、デルタ、オミクロンのSARS-CoV-2ウイルス分離株の分子特性をin vitroで比較した。
We hypothesized that the increased HS binding and decreased HS unbinding of the VOCs would come at the expense of decreased mobility, potentially providing an explanation for the current gradual within-lineage evolution of SARS-CoV-2, and recent basic amino acid reversions (such as Q493R (Figure 1e) to the original glutamine in Omicron BA4 and BA.5), which followed prior rapid emergence of highly divergent lineages.
我々は、VOC の HS 結合の増加と HS 解離の減少は、移動性の低下を犠牲にして起こると仮定し、SARS-CoV-2 の現在の緩やかな系統内進化と、非常に異なる系統の急速な出現に続く最近の基本アミノ酸の逆戻り (Omicron BA4 および BA.5 の Q493R (図 1e) から元のグルタミンへの逆戻りなど) を説明できる可能性があるとしました。
This scenario would have suggested that viral surfing acts as a dynamic selective force against the continued accumulation of positive charge on the S-protein.
このシナリオは、ウイルスサーフィンがSタンパク質上の正電荷の継続的な蓄積に対する動的な選択力として作用することを示唆している。
Alternatively, SARS-CoV-2 Omicron may have reached an optimal state that allows both processes, i.e. strong HS binding and effective movement through the HS-rich ECM, to occur.
あるいは、SARS-CoV-2オミクロンは、強力なHS結合とHSに富むECMを介した効果的な移動という両方のプロセスを可能にする最適な状態に達した可能性がある。
This latter scenario, however, would require an explanation of the apparent paradox that increased HS interaction strength (i.e, increased kon and decreased koff) does not restrict viral movement to the receptor, as would be expected from short stretches of free virus movement by diffusion interrupted by repeated cycles of prolonged ECM binding and virus immobilization.
しかし、後者のシナリオでは、HS相互作用強度の増加(すなわち、konの増加とkoffの減少)が受容体へのウイルスの移動を制限しないという明らかな矛盾を説明する必要がある。これは、拡散による短い自由ウイルス移動が、長期にわたるECM結合とウイルス固定化の繰り返しサイクルによって中断されることから予想される。
In this study, we used automated docking analyses to confirm increased free binding energies between the RBDs of the Wuhan, Delta and Omicron variants and a synthetic HS hexasaccharide model, and employed quartz crystal microbalance with dissipation monitoring (QCM-D) in combination with heparin- or HS-functionalized artificial membranes to mimic RBD and S-protein binding to the cellular surface ECM.
本研究では、自動ドッキング解析を使用して、武漢、デルタ、オミクロン変異体のRBDと合成HS六糖モデル間の自由結合エネルギーの増加を確認し、ヘパリンまたはHS機能化人工膜と組み合わせて散逸モニタリング機能付き水晶振動子マイクロバランス(QCM-D)を使用して、細胞表面ECMへのRBDおよびSタンパク質の結合を模倣しました。
These methods confirmed increasing HS interactions of the VOC RBDs and trimeric S-proteins, suggesting potentially restricted SARS-CoV-2 mobility on cellular surfaces.
これらの方法により、VOC RBD と三量体 S タンパク質の HS 相互作用が増加していることが確認され、細胞表面での SARS-CoV-2 の移動が制限される可能性があることが示唆されました。
However, we also found that trimeric Omicron S-proteins optimized HS binding along with the dynamics of “surfing” on and between HS without the need to detach from HS, potentially allowing this VOC to more effectively scan the cell surface and find the ACE2 receptor.
ただし、三量体 Omicron S タンパク質は、HS から分離する必要なく、HS 上および HS 間の「サーフィン」のダイナミクスとともに HS 結合を最適化し、この VOC が細胞表面をより効果的にスキャンして ACE2 受容体を見つけられるようにする可能性があることもわかりました。
Finally, we observed that the soluble, highly sulfated polysaccharide pentosan polysulfate (PPS) affected S-protein switching in vitro and was most effective in preventing Omicron infection in a cell-based bioassay.
最後に、可溶性で高度に硫酸化された多糖類ペントサンポリ硫酸 (PPS) が in vitro で S タンパク質の切り替えに影響し、細胞ベースのバイオアッセイで Omicron 感染を防ぐのに最も効果的であることが観察されました。
This suggests that the increased positive charge in Omicron S-proteins and their increased propensity to bind HS and “surf” the chains could be exploited pharmaceutically to target these important first steps in the viral infection pathway.
これは、Omicron S タンパク質の正電荷の増加と、HS に結合して鎖を「サーフィン」する傾向の増加を、ウイルス感染経路のこれらの重要な最初のステップを標的として薬理学的に利用できることを示唆しています。
Results:
Molecular modelling of VOC S-protein RBD binding to IAGAIA and PPS hexasaccharides reveals the evolution of stronger interactions
VOC Sタンパク質RBDのIAGAIAおよびPPS六糖類への結合の分子モデリングにより、より強い相互作用の進化が明らかになった
HS and heparin polysaccharides consist of alternating N-acetyl D-glucosamine and D-glucuronic acid residues that undergo extensive sulfation and epimerization during biosynthesis (Supplementary Figure 1).
HSとヘパリン多糖類は、生合成中に広範囲に硫酸化とエピマー化を受けるN-アセチルD-グルコサミンとD-グルクロン酸残基が交互に並んで構成されています(補足図1)。
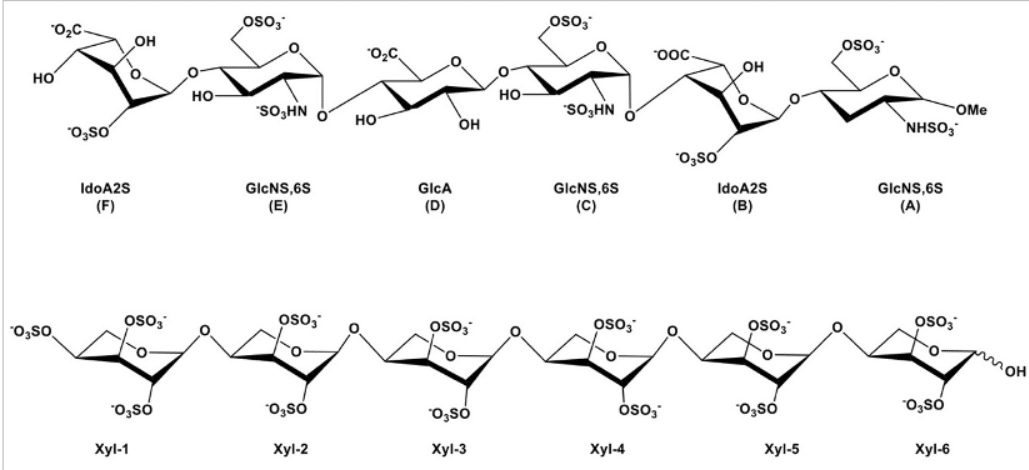
Owing to their high negative charge, linear HS can dynamically bind to positively charged sites on the surface of various soluble proteins and viruses.
線状HSは高い負電荷を帯びているため、さまざまな可溶性タンパク質やウイルスの表面にある正電荷を帯びた部位に動的に結合することができます。
Previous analyses of the Wuhan strain RBD surface revealed three putative heparin-binding sites.
武漢株RBD表面のこれまでの分析では、3つの推定ヘパリン結合部位が明らかになった。
Site I is defined by amino acid acids residues R346, N354, R355, K356, R357 and R466, while sites II and III contain K424, R454, R457, K458, K462, R466, and R403, R408, K417, K444, respectively (Figure 1c).
サイト I はアミノ酸残基 R346、N354、R355、K356、R357、および R466 によって定義され、サイト II および III にはそれぞれ K424、R454、R457、K458、K462、R466、および R403、R408、K417、K444 が含まれます (図 1c)。
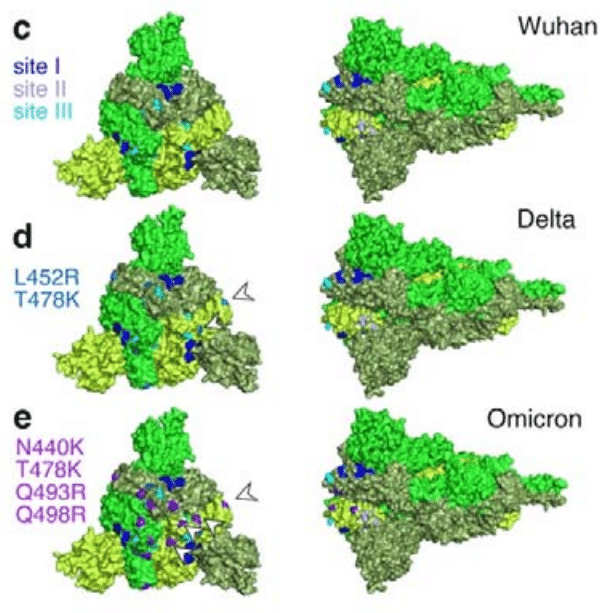
A hexasaccharide composed of uronic acid (α-IdoA2S/ β-GlcA) 1-4 linked to N,6-O disulfated glucosamine (α-GlcNS,6S) repeating units with the composition MeO-(4)-α-L-IdoA2S(1→4) α-D-GlcNS,6S (1→4) β-D-GlcA(1→4) α-D-GlcNS,6S (1→4) α-L-IdoA2S α-D-GlcNS,6S(1)-OMe (IAGAIA, Figure 2, Supplementary Figure 1) was previously identified as the minimal interacting heparin epitope.
MeO-(4)-α-L-IdoA2S(1→4) α-D-GlcNS,6S (1→4) β-D-GlcA(1→4) α-D-GlcNS,6S (1→4) α-L-IdoA2S α-D-GlcNS,6S(1)-OMe (IAGAIA、図2、補足図1) という組成を持つ、N,6-O二硫酸化グルコサミン (α-GlcNS,6S) 繰り返し単位に結合したウロン酸 (α-IdoA2S/ β-GlcA) 1-4 からなる六糖類は、以前に、最小限の相互作用ヘパリンエピトープとして同定されました。
Analogously, a hexasaccharide mimicking pentosan polysulfate (PPS), consisting of repeating, 1→4 linked 2,3-di-sulfo-Xyl-β (14) units, was included in our analysis (Figure 2, Supplementary Figure 1).
同様に、1→4 結合した 2,3-ジスルホキシル-β (14) 単位の繰り返しからなるペントサンポリ硫酸 (PPS) を模倣した六糖類も、私たちの分析に含めました (図 2、補足図 1)。
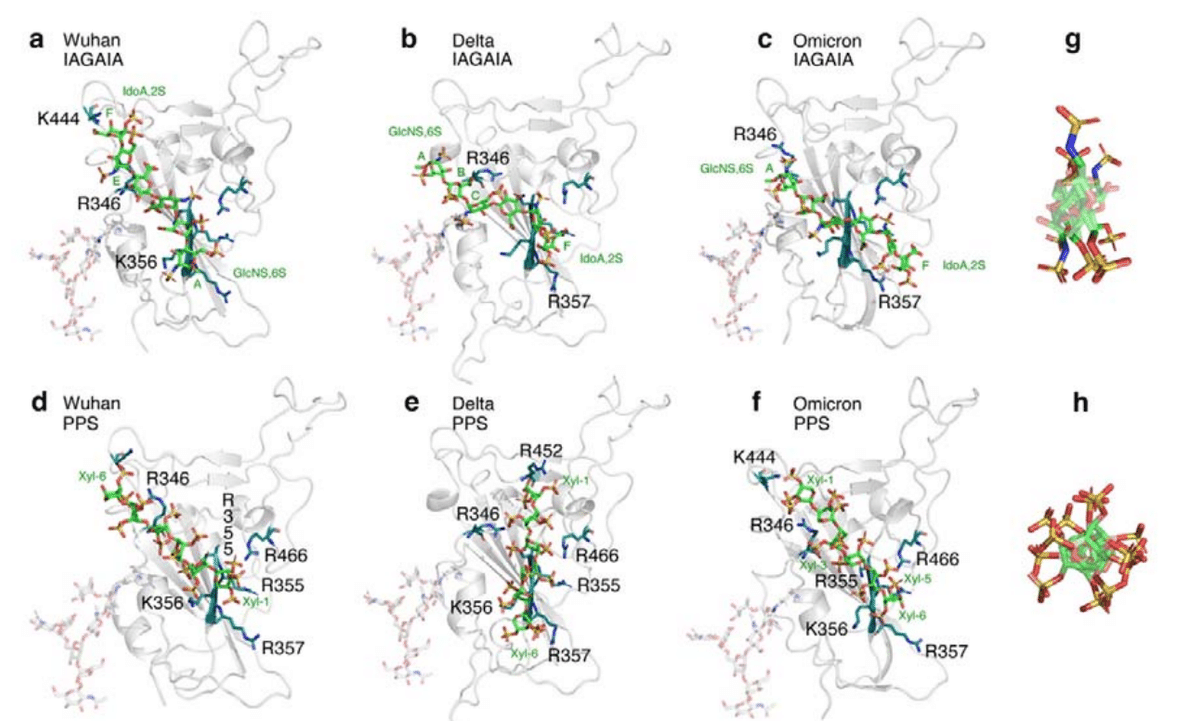
PPS is related to heparin and HS glycosaminoglycans in terms of anti-inflammatory and anticoagulant properties, but has a higher overall negative charge (>3 sulfo groups per disaccharide (PPS) versus <2.4 sulfo groups per disaccharide for heparin and approximately one sulfate group per HS disaccharide).
PPS は、抗炎症性および抗凝固性の点でヘパリンおよび HS グリコサミノグリカンに関連していますが、全体的な負電荷がより高くなっています (ヘパリンでは二糖類あたりスルホ基が 2.4 未満、HS 二糖類あたり硫酸基が約 1 であるのに対し、PPS では二糖類あたりスルホ基が 3 超)。
The PPS used here is a plant-derived drug obtained by sulfonation of β-(1→4) xylan from beech wood and is the active ingredient in Elmiron®, an FDA-approved drug for the treatment of interstitial cystitis and for thromboembolic prophylaxis.
ここで使用されるPPSは、ブナ材由来のβ-(1→4)キシランをスルホン化して得られる植物由来の薬剤であり、間質性膀胱炎の治療および血栓塞栓症予防のためのFDA承認薬であるエルミロン®の有効成分です。
Furthermore, PPS has recently attracted attention due to interesting activity against SARS-CoV-2 replication.
さらに、PPSは最近、SARS-CoV-2の複製に対する興味深い活性により注目を集めています。
We analyzed the interaction between these two hexasaccharide models and the RBDs of the Wuhan, Delta, and Omicron variants using molecular docking (Glide tool, Schrödinger Inc.) and ranked the selected poses by re-scoring the estimated free energy of binding according to the Molecular Mechanics, General Born Surface Area (MMGBSA) approach, a method to calculate free energies of ligand-protein interactions (Prime tool, Schrödinger Inc.).
分子ドッキング(Glide ツール、Schrödinger Inc.)を使用して、これら 2 つの六糖モデルと Wuhan、Delta、および Omicron バリアントの RBD 間の相互作用を分析し、リガンド - タンパク質相互作用の自由エネルギーを計算する方法である分子力学、一般ボーン表面積(MMGBSA)アプローチに従って推定結合自由エネルギーを再スコアリングして、選択したポーズをランク付けしました(Prime ツール、Schrödinger Inc.)。
Molecular docking predicts that glycosaminoglycan oligosaccharides IAGAIA and PPS preferentially bind site I of the Wuhan RBD: residues R346, N354, R355, K356, R357 and R466 are found within 3 Å from the oligosaccharide in the best-selected poses (Supplementary Table 3), despite their different relative positioning and orientation (Figure 2, Supplementary Figure 2a-c).
分子ドッキングにより、グリコサミノグリカンオリゴ糖 IAGAIA と PPS が武漢 RBD のサイト I に優先的に結合すると予測されます。残基 R346、N354、R355、K356、R357、および R466 は、相対的な位置と方向が異なっているにもかかわらず、最適選択されたポーズ (補足表 3) でオリゴ糖から 3 Å 以内にあります (図 2、補足図 2a-c)。
Indeed, the best pose between IAGAIA and the Wuhan RBD shows close contact between residues of site I and monosaccharide sugars GlcNS6S (E in Supplementary Figure 1), GlcNS6S (C), IdoA (B), and GlcNS6S (A), in that order (Figure 2a).
実際、IAGAIA と武漢 RBD 間の最適ポーズは、サイト I の残基と単糖糖 GlcNS6S (補足図 1 の E)、GlcNS6S (C)、IdoA (B)、および GlcNS6S (A) の順で密接に接触していることを示しています (図 2a)。
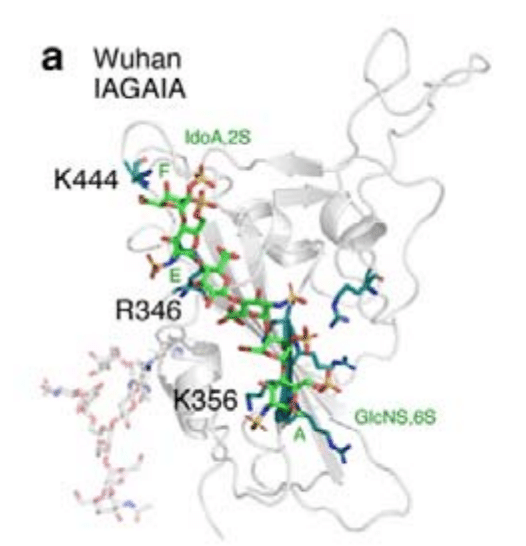
In contrast, the best pose of IAGAIA and the Delta RBD shows that this set of amino acids contacts the following sugars: IdoA (B), GlcNS6S (C), IdoA (D), GlcNS6S (E), and IdoA (F), in that order (Figure 2b).
対照的に、IAGAIA と Delta RBD の最適なポーズは、このアミノ酸セットが次の糖と接触していることを示しています: IdoA (B)、GlcNS6S (C)、IdoA (D)、GlcNS6S (E)、IdoA (F)の順序で (図 2b)。
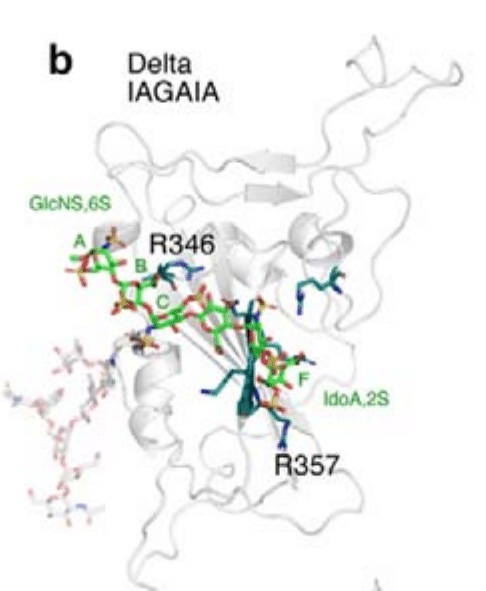
The head-tail orientation of IAGAIA in the Delta RBD is opposite to that predicted in the Wuhan RBD model.
Delta RBD における IAGAIA の頭尾方向は、武漢 RBD モデルで予測されたものとは逆です。
Even though the total number of contacts between IAGAIA and site I do not significantly change among both RBD models, the number of salt bridges and van der Waals contacts increases, and correspondingly, the estimated free energy of binding increases (slightly) from -18 to -26 Kcal/mol (Table 1).
IAGAIA とサイト I の間の接触の総数は両方の RBD モデル間で大きく変化しませんが、塩橋とファンデルワールス接触の数が増加し、それに応じて推定結合自由エネルギーが (わずかに) -18 Kcal/mol から -26 Kcal/mol に増加します (表 1)。
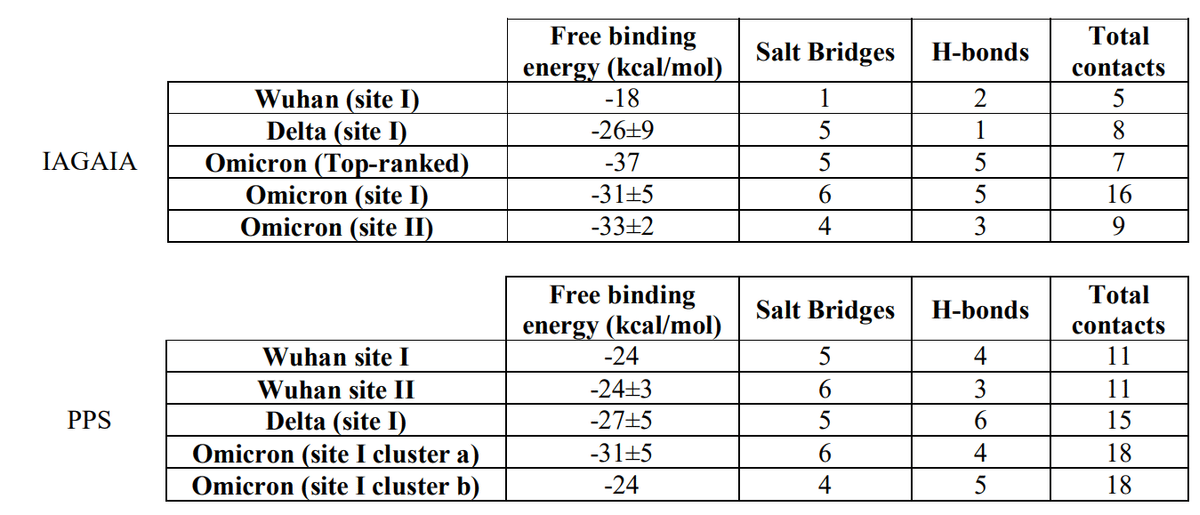
The IAGAIA in the bound state with the Omicron RBD shows an epitope binding comparable to that of the Delta model and reveals the same monosaccharide – protein contact order: residues R346, N354, R355, K356, R357 and R466 are in contact with the monosaccharides GlcNS6S (A), IdoA (B), GlcNS6S (C), IdoA (D), GlcNS6S (E), and IdoA (F), in that order (Figure 2 c).
Omicron RBD と結合した状態の IAGAIA は、Delta モデルと同等のエピトープ結合を示し、同じ単糖 - タンパク質接触順序を示します。残基 R346、N354、R355、K356、R357、および R466 は、単糖 GlcNS6S (A)、IdoA (B)、GlcNS6S (C)、IdoA (D)、GlcNS6S (E)、および IdoA (F) とこの順序で接触しています (図 2 c)。
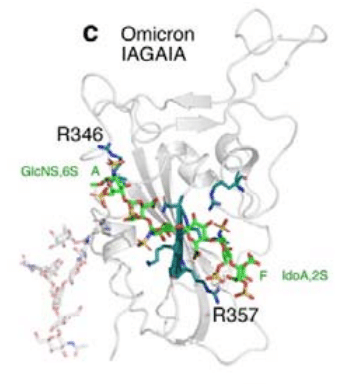
The estimated free energy of binding that characterizes the interaction between IAGAIA and Omicron is significantly greater than what we observed when the same glycan binds the Delta and Wuhan RBDs, as shown by the higher number of favourable contacts compared to the other models.
IAGAIA と Omicron の相互作用を特徴付ける推定結合自由エネルギーは、同じグリカンが Delta および Wuhan RBD に結合したときに観測されたものよりも大幅に大きく、他のモデルと比較して好ましい接触の数が多いことからもわかります。
This suggests a greater propensity of the surface of Omicron RBD to host heparin oligosaccharides (Table 1).
これは、Omicron RBD の表面がヘパリンオリゴ糖をホストする傾向が高いことを示しています (表 1)。
The PPS oligosaccharide displays a radial distribution of negatively charged sulfated groups along its molecular axis (Figure2 g,h).
PPS オリゴ糖は、分子軸に沿って負に帯電した硫酸基の放射状分布を示します (図 2 g、h)。
Therefore, we propose a higher probability of the PPS sulfate groups to engage, in non-directional salt bridge interactions, the positively charged patches of Arg and Lys residues that are solvent exposed on the RBD surface (Supplementary Figure 2d-f).
したがって、PPS 硫酸基が、非方向性塩橋相互作用において、RBD 表面で溶媒にさらされている Arg および Lys 残基の正電荷パッチと係合する確率が高いと考えられます (補足図 2d-f)。
In the best docking pose of PPS in the Wuhan RBD model, the monosaccharide units Xyl-6, Xyl-2, and Xyl-1, in that order, are closer to site I residues (Figure 2 d).
Wuhan RBD モデルにおける PPS の最適なドッキング ポーズでは、単糖ユニット Xyl-6、Xyl-2、Xyl-1 の順に、サイト I 残基に近くなります (図 2 d)。
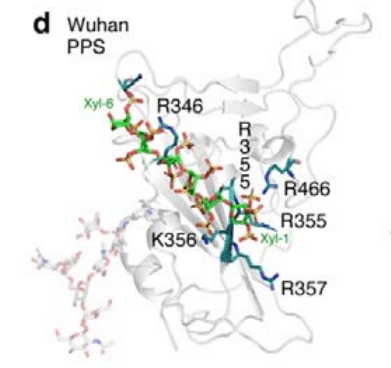
Interestingly, PPS shows a greater number of salt bridges and contacts, probably correlated to both an increase of the negative charges and a uniform distribution of sulfate groups compared to IAGAIA.
興味深いことに、PPS はより多くの塩橋と接触を示しており、これはおそらく IAGAIA と比較して負電荷の増加と硫酸基の均一な分布の両方に相関していると考えられます。
This allows for more efficient interactions with the basic channel of the RBD surface (Supplementary Table 3).
これにより、RBD 表面の基本チャネルとのより効率的な相互作用が可能になります (補足表 3)。
Interestingly, the contact efficiency between PPS and RBD increases from Wuhan to Delta to Omicron RBD variant models, respectively, and the free energy of binding also increases (Table 1).
興味深いことに、PPS と RBD 間の接触効率は、それぞれ Wuhan から Delta、Omicron RBD バリアント モデルへと増加し、結合の自由エネルギーも増加します (表 1)。
Indeed, in the best pose of PPS in both the Delta and Omicron RBDs, all residues of site I engage the ligand at the Xyl-5, Xyl-4, Xyl-2 and Xyl-1 units via an additional contact between the mutated residue R452 and Xyl-1 (Figure 2e).
実際、Delta および Omicron RBD の両方における PPS の最適な姿勢では、サイト I のすべての残基が、変異残基 R452 と Xyl-1 間の追加の接触を介して、Xyl-5、Xyl-4、Xyl-2、および Xyl-1 ユニットでリガンドと結合します (図 2e)。
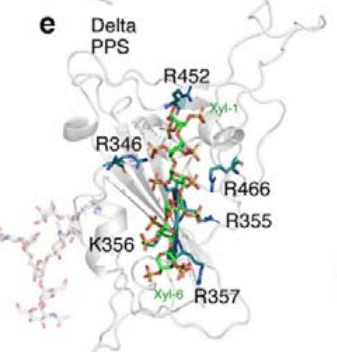
In summary, the docking results for the IAGAIA and PPS oligosaccharides show a preference for binding to site I for all of the VOC RBDs.
要約すると、IAGAIA および PPS オリゴ糖のドッキング結果は、すべての VOC RBD に対してサイト I への結合が優先されることを示しています。
The analysis of the docking calculations suggests that the interactions between the basic RBD residues and the negatively charged groups of IAGAIA and PPS are mainly charge-based.
ドッキング計算の分析は、基本 RBD 残基と IAGAIA および PPS の負に帯電したグループ間の相互作用は主に電荷に基づいていることを示唆しています。
For PPS, the docking results reveal a conserved binding mode for each RBD tested, establishing an extended network of salt bridges and H-bonds compared to IAGAIA.
PPS の場合、ドッキング結果から、テストされた各 RBD の結合モードが保存されていることが明らかになり、IAGAIA と比較して塩橋と水素結合の拡張ネットワークが確立されました。
This increases the affinity to sulfated glycans during the evolution of Wuhan to Delta and to Omicron RBDs (Table 1, Supplementary Table 2).
これにより、Wuhan から Delta および Omicron RBD への進化中に硫酸化グリカンに対する親和性が増加します (表 1、補足表 2)。
SARS-CoV-2 VOC S-protein RBDs have evolved towards increased HS crosslinking
SARS-CoV-2 VOC Sタンパク質RBDはHS架橋の増加に向かって進化した
Next, we tested the prediction of improved glycan interactions during SARS-CoV-2 evolution with HS using QCM-D.
次に、QCM-D を使用して、SARS-CoV-2 の進化中に HS とグリカンの相互作用が改善されるという予測をテストしました。
The core of QCM-D is an oscillating quartz crystal sensor disk with a resonance frequency related to the mass of the disk (Supplementary Figure 4a).
QCM-D の核となるのは、ディスクの質量に関連する共振周波数を持つ振動する水晶センサー ディスクです (補足図 4a)。
This allows the real-time detection of nanoscale mass changes on the sensor surface by monitoring changes in the (normalized) resonance frequency (ΔF): adsorption of molecules on the surface decreases F, while mass loss of mass increases F (Supplementary Figure 4b).
これにより、(正規化された)共振周波数(ΔF)の変化を監視することで、センサー表面のナノスケールの質量変化をリアルタイムで検出できます。表面への分子の吸着はFを減少させ、質量損失はFを増加させます(補足図4b)。
QCM-D measures an additional parameter, the change in energy dissipation D, which is particularly useful for studying soft layer properties, as ΔD correlates with layer softness (Supplementary Figure 4b).
QCM-D は、エネルギー散逸 D の変化という追加のパラメータを測定します。これは、ΔD が層の柔らかさと相関するため、特にソフト層の特性を研究するのに有用です (補足図 4b)。
Using this technique, we investigated the interaction of RBDs with HS functionalized surfaces, which were formed on top of supported lipid bilayers (SLBs) as a plasma membrane model (Supplementary Figure 4a).
この技術を使用して、RBD と HS 機能化表面との相互作用を調査しました。HS 機能化表面は、細胞膜モデルとして支持脂質二重層 (SLB) の上に形成されました (補足図 4a)。
We used heparin instead of HS because the RBD, monomeric S-protein, and trimeric S-proteins show strong binding to polysaccharides that are composed of trisulfated repeating units (IdoA2S-GlcNS6S), a motif typically found in heparin chains.
RBD、単量体Sタンパク質、三量体Sタンパク質は、ヘパリン鎖に典型的に見られるモチーフである三硫酸化繰り返し単位(IdoA2S-GlcNS6S)で構成される多糖類に強く結合するため、HSの代わりにヘパリンを使用しました。
The SLBs were formed through the rupture of small unilamellar vesicles (SUVs) containing 5 mol% biotinylated lipids.
SLBは、5 mol%のビオチン化脂質を含む小さな単層小胞(SUV)の破裂によって形成されました。
Highly sulfated heparin chains were anchored to the SLBs through a streptavidin linker via a biotin moiety at the reducing end and served as a proxy for cell surface HS (Supplementary Figure 4a).
高度に硫酸化されたヘパリン鎖は、還元末端のビオチン部分を介してストレプトアビジンリンカーを介してSLBに固定され、細胞表面HSの代理として機能しました(補足図4a)。
To mimic RBD contact with the extracellular matrix, we then added the RBD and monitored mass increases upon its adsorption to the heparin surface, as indicated by decreased resonance frequencies (-ΔF), as well as changes in the softness of the heparin film upon protein binding (as reflected by the dissipation shift ΔD).
RBDと細胞外マトリックスの接触を模倣するために、RBDを追加し、ヘパリン表面への吸着時の質量増加(共鳴周波数の低下(-ΔF)で示される)と、タンパク質結合時のヘパリンフィルムの柔らかさの変化(散逸シフトΔDで反映)をモニタリングしました。
As shown in Figure 3a, addition of unlabeled RBDs of the Wuhan, Delta and Omicron variants on top of a heparin functionalized QCM-D chip induced rapid frequency decreases (- ΔF) of approximately 60 Hz, indicating similarly fast binding of all RBDs to heparin (and specific binding to heparin and not the lipid or streptavidin, Supplementary Figure 4c).
図 3a に示すように、ヘパリン機能化 QCM-D チップ上に Wuhan、Delta、Omicron バリアントの非標識 RBD を追加すると、約 60 Hz の急速な周波数低下 (- ΔF) が誘発され、すべての RBD がヘパリンに同様に速く結合していることを示しています (脂質やストレプトアビジンではなくヘパリンに特異的に結合しています、補足図 4c)。
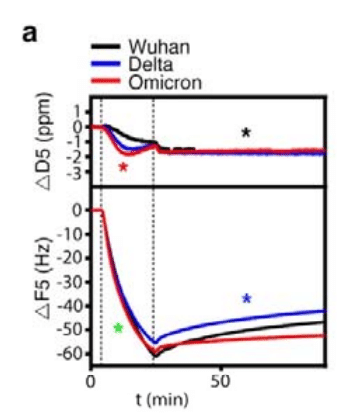
We also observed that the energy dissipation (ΔD) of the layer decreased proportionally during RBD binding, suggesting protein-induced stiffening of the heparin film, which could be caused by cross-linking the heparin chains.
また、RBD 結合中に層のエネルギー散逸 (ΔD) が比例して減少することも観察されました。これは、ヘパリン フィルムのタンパク質誘導硬化を示唆しており、これはヘパリン鎖の架橋によって引き起こされる可能性があります。
However, while the overall decrease in D was very similar for all RBDs (Figure 3a, black asterisk), indicating similar capacities to cross-link surface heparin, we found that the decrease in D occurred earlier (that is, at a lower RBD surface coverage) for Omicron and Delta RBDs compared to the Wuhan RBD.
しかし、D の全体的な減少はすべての RBD で非常に類似しており (図 3a、黒のアスタリスク)、表面ヘパリンを架橋する能力が類似していることを示していますが、D の減少は、武漢 RBD と比較して、オミクロン RBD とデルタ RBD でより早く (つまり、RBD 表面被覆率が低いときに) 発生することがわかりました。
This finding suggests a higher cross-linking propensity of these variants compared to the Wuhan RBD (red asterisk).
この発見は、武漢 RBD (赤のアスタリスク) と比較して、これらのバリアントの架橋傾向が高いことを示唆しています。
Parametric plots of ΔD/-ΔF (a measure of film softness) vs. -ΔF (a measure of surface density) confirmed the evolving cross-linking propensity (Figure 3b), with more pronounced rigidification (and thus cross-linking) of the Delta and Omicron RBDs compared to the Wuhan RBD at any given RBS surface coverage (-ΔF shift), except at the highest coverage where the degree of stiffening is similar for all variants.
ΔD/-ΔF(フィルムの柔らかさの尺度)対-ΔF(表面密度の尺度)のパラメトリックプロットは、進化する架橋傾向(図3b)を確認し、任意のRBS表面被覆率(-ΔFシフト)で、デルタおよびオミクロンRBDの硬直化(したがって架橋)が武漢RBDよりも顕著でした。 ただし、すべてのバリアントで硬化の程度が類似している最高被覆率を除きます。
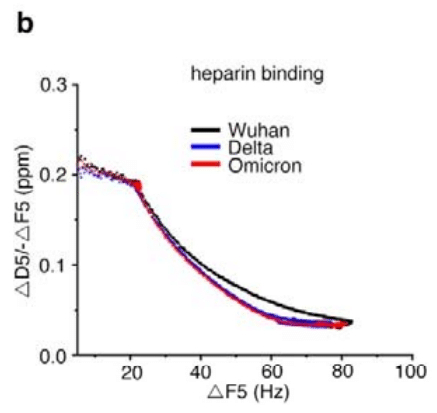
The same effect was also observed when a lower-sulfated HS was coupled to the QCM-D sensor surface instead of heparin (Figure 3c).
ヘパリンの代わりに硫酸化の低いHSをQCM-Dセンサー表面に結合させた場合にも、同じ効果が観察されました(図3c)。
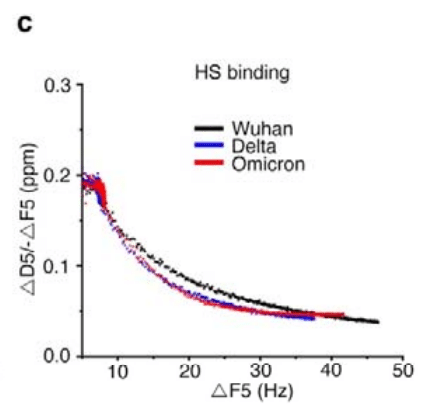
This confirmed that SARS-CoV-2 RBD binding and the associated but distinct HS film stiffening are qualitatively preserved over different HS sulfation levels.
これにより、SARS-CoV-2 RBD 結合と、それに関連するが明確な HS フィルムの硬化が、HS 硫酸化レベルの違いにかかわらず定性的に維持されていることが確認されました。
Finally, we observed that while all RBDs remained largely bound during wash buffer injection into the QCM-D chambers, dissociation of the Wuhan RBD (and to a lesser degree also the Delta variant) was increased relative to that of the Omicron variant, as evidenced by an observable increase in ΔF during the 60-minute wash step (Figure 3a, blue asterisk, Supplementary Figure 4d-f).
最後に、すべての RBD は QCM-D チャンバーへの洗浄バッファー注入中に大部分が結合したままでしたが、武漢 RBD (および程度は低いが Delta バリアント) の解離は、60 分間の洗浄ステップ中に ΔF が顕著に増加したことからわかるように、オミクロン バリアントに比べて増加しました (図 3a、青いアスタリスク、補足図 4d-f)。
Taken together, the faster increase in heparin layer stiffness (evidenced by -ΔD) and the increased half-life of the interaction (evidenced by small changes in ΔF during prolonged washing of the sensor surface) indicate that the Omicron RBD has evolved to rapidly establish multivalency and to reduce the koff of the interaction.
まとめると、ヘパリン層の剛性の急速な増加(-ΔD で証明)と相互作用の半減期の増加(センサー表面の長時間洗浄中の ΔF の小さな変化で証明)は、Omicron RBD が多価性を急速に確立し、相互作用の koff を低下させるように進化したことを示しています。
These findings are supported by the in silico calculations predicting stronger HS binding of the Delta and Omicron RBDs (Supplementary Table 1) and by an increase of the number of contacts (Figure 2, Supplementary Table 3).
これらの知見は、Delta および Omicron RBD の HS 結合が強くなると予測する in silico 計算(補足表 1)と接触数の増加(図 2、補足表 3)によって裏付けられています。
Nevertheless, the RBD association kinetics with the heparin-functionalized sensor surface are not much affected by accumulated charge during evolution, as evidenced by a similar slope of ΔF during RBD binding (Figure 3a, green asterisk).
それにもかかわらず、RBD とヘパリン機能化センサー表面との結合速度は、進化中に蓄積された電荷によってあまり影響されません。これは、RBD 結合中の ΔF の同様の傾斜によって証明されています (図 3a、緑のアスタリスク)。
Instead, these evolutionary changes ensure that the virus remains bound to HS more effectively by increasing the cross-linking kinetics so that the newer virus variants do not diffuse away from the cell surface, which would hinder infection.
代わりに、これらの進化的変化により、架橋速度が上昇し、ウイルスがより効果的に HS に結合したままでいることが保証され、新しいウイルス変異体が細胞表面から拡散しなくなります。 拡散すると感染が妨げられます。
Finally, we note that despite the relatively short range of electrostatic forces of soluble proteins at physiological ionic strength, their marked increase in the RBDs of the newer virus variants (Figure 3d) may facilitate favorable RBD orientations toward HS of the opposite charge to increase the speed of HS/heparin cross-linking over short distances.
最後に、生理的イオン強度での可溶性タンパク質の静電力の範囲は比較的短いにもかかわらず、新しいウイルス変異体の RBD の顕著な増加 (図 3d) により、反対電荷の HS に向かって RBD が有利に配向し、短距離での HS/ヘパリン架橋の速度が上昇する可能性があることに注目します。
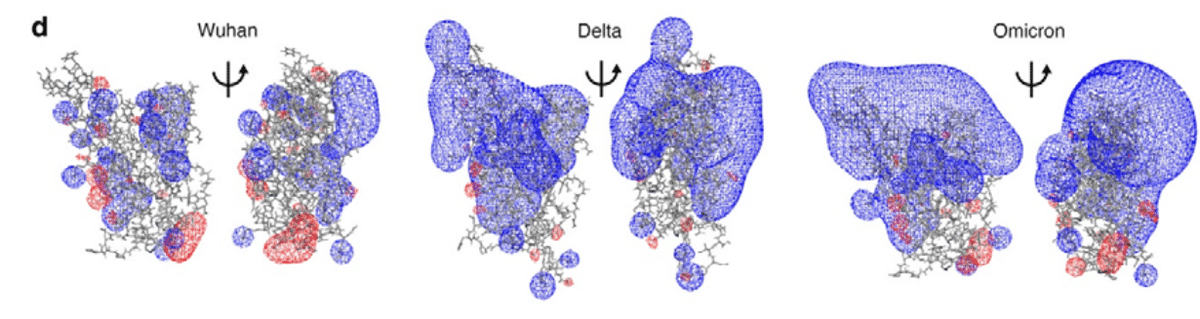
SARS-CoV-2 VOC S-protein RBDs have evolved towards increased HS interaction stability
SARS-CoV-2 VOC Sタンパク質RBDはHS相互作用の安定性の向上に向けて進化した
Although our results indicated only modest rates of dissociation of VOC RBDs from the sensor surface into the wash buffer, this is true in general for surface measurements due to analyte rebinding.
我々の結果は、VOC RBD がセンサー表面から洗浄バッファーに解離する速度が中程度であることを示しているが、これは分析対象物の再結合による表面測定では一般的に当てはまる。
In these cases, the exchange rate between surface molecules can be obtained by the addition of soluble acceptor ligands.
これらの場合、表面分子間の交換速度は可溶性アクセプターリガンドを追加することで得られる。
To account for this, we adapted our previously established protocol and injected increasing amounts of soluble heparin onto the RBD-loaded heparin film as a competitor.
これを説明するため、私たちは以前に確立したプロトコルを改良し、競合相手としてRBDを充填したヘパリンフィルムに可溶性ヘパリンを徐々に注入しました。
As shown in Figure 4a and Supplementary Figure 5a, soluble heparin served as a moderately effective acceptor for the RBD of the Wuhan variant, as evidenced by an increase in D (black top asterisk) and F as a result of RBD desorption from the sensor surface into the soluble phase (black bottom asterisk, and Supplementary Figure 5b).
図4aと補足図5aに示されているように、可溶性ヘパリンは武漢変異体のRBDに対して中程度の効果的な受容体として機能しました。これは、RBDがセンサー表面から可溶性相(黒い下部のアスタリスク)に脱着した結果としてD(黒い上部のアスタリスク)とFが増加したことで証明されています(補足図5b)。
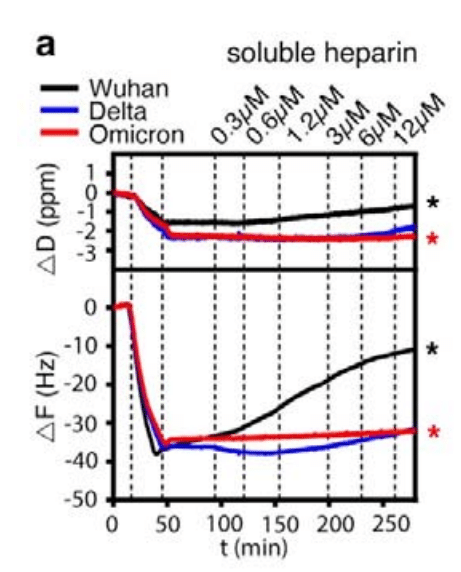
We also found that soluble heparin desorbed the Delta RBD, at least at high heparin concentrations.
また、可溶性ヘパリンが Delta RBD を脱着することもわかりました。少なくともヘパリン濃度が高い場合です。
In remarkable contrast, the RBD of the Omicron variant remained firmly associated with the QCM-D sensor surface, even when challenged with 12 mM heparin as a soluble acceptor (Figure 4a, red asterisks).
対照的に、Omicron バリアントの RBD は、可溶性受容体として 12 mM ヘパリンを作用させた場合でも、QCM-D センサー表面としっかりと結合したままでした (図 4a、赤いアスタリスク)。
This indicated that Omicron RBD has slower disassociation kinetics from HS chains compared to the Wuhan variant RBD, consistent with no observable dissociation during buffer wash (Figure 3a, Supplementary Figure 5b).
これは、オミクロンRBDは武漢変異体RBDと比較してHS鎖からの解離速度が遅いことを示し、緩衝液洗浄中に解離が観察されなかったことと一致しています(図3a、補足図5b)。
Furthermore, we observed the same trend when PPS was added to the wash buffer instead of heparin (Figure 4b, and Supplementary Figure 5c).
さらに、ヘパリンの代わりにPPSを洗浄緩衝液に加えた場合も同じ傾向が観察された(図4b、補足図5c)。
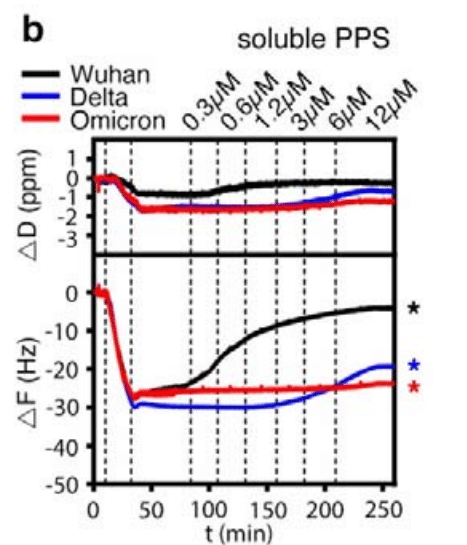
Consistent with the increase in negative charge, the capacity of PPS to elute the RBDs of the Wuhan and Delta variants was higher than that of heparin.
負電荷の増加と一致して、PPS が武漢およびデルタ変異体の RBD を溶出する能力はヘパリンよりも高かった。
However, the RBD of the Omicron variant also remained firmly associated with the QCM-D sensor surface when challenged with PPS (Figure 4b, red asterisk, Supplementary Figure 5d).
しかし、PPS で刺激すると、オミクロン変異体の RBD も QCM-D センサー表面としっかりと結合したままであった (図 4b、赤いアスタリスク、補足図 5d)。
This confirmed that the Omicron RBD has evolved towards more persistent HS binding, probably facilitated by its ability to bind two or more chains simultaneously and the increased free binding energies of interaction (Table 1).
これにより、オミクロン RBD がより持続的な HS 結合に向けて進化したことが確認された。これは、おそらく 2 つ以上の鎖を同時に結合する能力と、相互作用の自由結合エネルギーの増加によって促進されたと考えられる (表 1)。
It has been shown previously that pre-incubation with soluble heparin impairs subsequent S-protein or RBD binding to cells and abrogates SARS-CoV-2 infection.
可溶性ヘパリンでプレインキュベーションすると、その後のSタンパク質またはRBDの細胞への結合が阻害され、SARS-CoV-2感染が阻止されることが以前に示されています。
As an in vitro model, we preincubated the RBDs with 100 nM heparin or PPS before passing them over the heparin-functionalized QCM-D sensors.
試験管内モデルとして、RBD を 100 nM ヘパリンまたは PPS でプレインキュベートしてから、ヘパリン機能化 QCM-D センサーに通しました。
Pre-incubation of 200nM (5µg/ml) of the Wuhan variant RBD with heparin or PPS greatly delayed protein association with the heparin-functionalized sensor surface, as evidenced by a strongly reduced binding slope and a strongly reduced –ΔF (Figure 4c).
200nM (5µg/ml) の武漢変異体 RBD をヘパリンまたは PPS でプレインキュベートすると、ヘパリン機能化センサー表面とのタンパク質の結合が大幅に遅れました。これは、結合勾配が大幅に減少し、–ΔF が大幅に減少したことで証明されています (図 4c)。
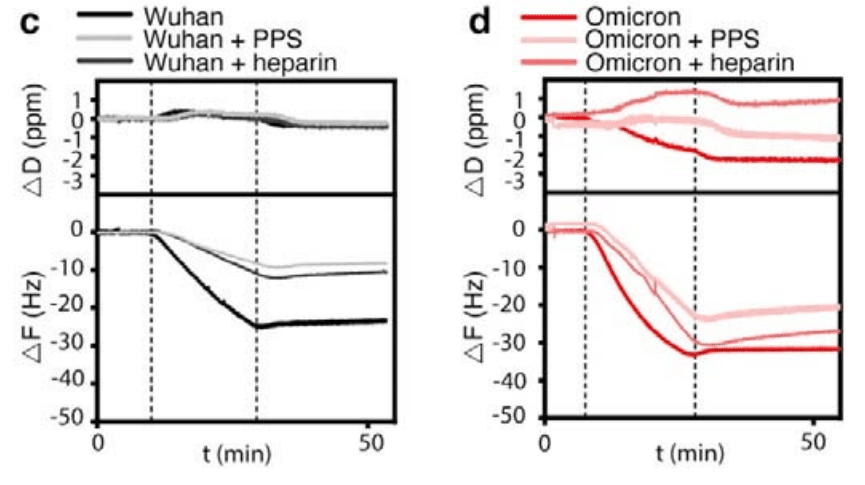
Therefore, preincubation with heparin or PPS is a potential pharmaceutical approach to reduce cellular infection by decreasing the likelihood of virus binding to the cells.
したがって、ヘパリンまたはPPSによるプレインキュベーションは、ウイルスが細胞に結合する可能性を減らすことで細胞感染を減らすための潜在的な薬理学的アプローチです。
The largely unchanged ΔD suggests that the Wuhan RBD does not stiffen the matrix much, presumably due to the reduced cross-linking of the preincubated RBD, as it is already glycan-associated.
ΔD がほとんど変化していないことから、Wuhan RBD はマトリックスをあまり硬くしないことが示唆されます。これは、すでにグリカン結合しているため、プレインキュベートされた RBD の架橋が減少したためと考えられます。
In contrast, heparin and PPS preincubation with Omicron RBD altered the surface-binding to a lesser extent, and the capacity of the pretreated Omicron RBD to cross-link surface-bound heparin was strongly reduced (Figure 4d).
対照的に、ヘパリンと PPS を Omicron RBD とプレインキュベートした場合、表面結合はそれほど変化せず、前処理された Omicron RBD が表面に結合したヘパリンを架橋する能力は大幅に低下しました (図 4d)。
These results suggest that PPS preincubation inhibits the association of the Omicron RBD with the sensor surface to a greater extent than heparin, which is only moderately effective, while both heparin and PPS more effectively inhibit the association of the Wuhan variant RBD.
これらの結果は、PPS プレインキュベーションが、中程度にしか効果がないヘパリンよりも、オミクロン RBD とセンサー表面の結合をより強く阻害することを示唆している。 一方、ヘパリンと PPS はどちらも、武漢変異体 RBD の結合をより効果的に阻害する。
These results are consistent with a previous study showing that PPS inhibits most S-protein RBD mutants better than heparin.
これらの結果は、PPS がほとんどの S タンパク質 RBD 変異体をヘパリンよりも効果的に阻害することを示す以前の研究と一致している。
[46]. The results also support the idea that the S-protein RBD does not recognize a specific HS binding motif, and relies on non-selective electrostatic interactions with the HS in the ECM.
この結果は、S タンパク質 RBD が特定の HS 結合モチーフを認識せず、ECM 内の HS との非選択的な静電相互作用に依存しているという考えも裏付けています。
This, in turn, highlights the importance of increasing charge differences between the S-proteins and the ECM during viral evolution to maintain the initial contact and to enhance the chances of subsequent cellular infection.
これは、ウイルスの進化中に S タンパク質と ECM 間の電荷差を増やすことが、最初の接触を維持し、その後の細胞感染の可能性を高めるために重要であることを強調しています。
Trimeric SARS-CoV-2 VOC S-proteins have evolved towards increased capability for intersegmental transfer between HS
三量体SARS-CoV-2 VOC Sタンパク質は、HS間の分節間伝達能力の向上に向けて進化した
The results so far supported the contention that negatively charged glycans, particularly HS, act as attachment factors for SARS-CoV2 to the ECM, and that maintaining the interaction has been optimized during the evolution of the Omicron RBD.
これまでの結果は、負に帯電したグリカン、特にHSがSARS-CoV2のECMへの付着因子として機能し、相互作用の維持がオミクロンRBDの進化の過程で最適化されてきたという主張を裏付けている。
However, diffusion of SARS-CoV-2 through the mucous layer of the airway epithelium (viral surfing) is also essential for binding to ACE2, cellular entry, and infection.
しかし、気道上皮の粘液層を介したSARS-CoV-2の拡散(ウイルスサーフィン)も、ACE2への結合、細胞侵入、感染に不可欠です。
In this regard, our results suggest a detrimental effect of increased HS interaction half-life, since the newer SARS-CoV-2 variants are more likely to be trapped in the ECM.
この点に関して、私たちの結果は、新しい SARS-CoV-2 変異体が ECM に閉じ込められる可能性が高いため、HS 相互作用の半減期の延長が悪影響を及ぼすことを示唆しています。
This would reduce their viral surfing capabilities by intermittent free diffusion, which would stand in contradiction to the observed increase in infectivity and transmissibility of the VOCs.
これにより、断続的な自由拡散によってウイルスサーフィン能力が低下しますが、これは VOC の感染性と伝染性の観察された増加と矛盾します。
We hypothesized that this discrepancy may possibly be explained by different HS binding of the VOC RBDs and the trimeric S-proteins that decorate the viral surface.
この矛盾は、VOC RBDとウイルス表面を飾る三量体Sタンパク質のHS結合が異なることで説明できる可能性があると仮説を立てました。
In fact, the avidity to HS is likely to be higher for the trimeric S-protein than for the monomeric RBD, due to the cooperative effect provided by its multimeric state (Figure 1c-e), and is supported by the observation that for the Wuhan variant the RBD domain binds to heparin with moderate affinity (KD ∼1 μM), whereas the trimeric S-protein binds with much higher affinity (KD = 64 nM).
実際、HSに対する親和性は、多量体状態によってもたらされる協同効果により、三量体Sタンパク質の方が単量体RBDよりも高くなる可能性があり(図1c-e)、武漢変異体の場合、RBDドメインは中程度の親和性(KD 約1μM)でヘパリンに結合するのに対し、三量体Sタンパク質ははるかに高い親和性(KD = 64 nM)で結合するという観察によって裏付けられています。
We also observed that the acquired mutations of the trimeric Omicron S-proteins make a sizeable contribution to the affinity of the protein/heparin interaction, decreasing the dissociation constant (Kd) from 2.65 ± 0.4 nM for the Wuhan variant to 1.5 ± 0.2 nM for the Omicron variant, as determined by the established QCM-D set-up (Supplementary Figure 6a).
また、三量体オミクロンSタンパク質の獲得変異がタンパク質/ヘパリン相互作用の親和性に大きく寄与し、確立されたQCM-Dセットアップで測定された解離定数(Kd)が武漢変異体の2.65±0.4nMからオミクロン変異体の1.5±0.2nMに低下していることも観察されました(補足図6a)。
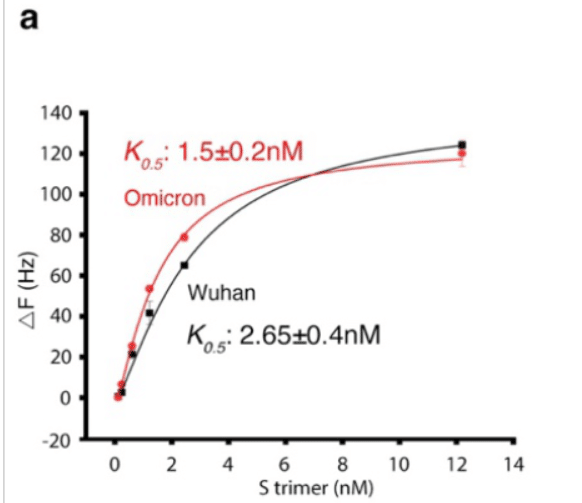
However, we again note that such increased S-protein interactions with negatively charged HS would be expected to interfere with, rather than enhance, the rapid movement of SARS-CoV-2 Omicron through the ECM and across the surfaces of epithelial cells.
しかし、負に帯電したHSとのSタンパク質の相互作用の増加は、SARS-CoV-2オミクロンのECMおよび上皮細胞の表面を介した急速な移動を促進するのではなく、妨げると予想されることに再度留意する。
To resolve the potential paradox of increased binding affinity increasing viral surfing, we investigated the binding of trimeric S-proteins of the Wuhan and Omicron variants to the heparin functionalized QCM-D chips, followed by injection of increasing amounts of soluble heparin.
結合親和性の増加がウイルスサーフィンを増加させるという潜在的なパラドックスを解決するために、武漢およびオミクロン変異体の三量体Sタンパク質のヘパリン機能化QCM-Dチップへの結合、続いて増加する量の可溶性ヘパリンを注入することを調べました。
As shown in Figure 5a and Supplementary Figure 6b and 6d, soluble heparin served as a moderately effective acceptor for the Wuhan variant trimeric S-proteins, and a large fraction of the protein could not be displaced from the surface even at high soluble heparin concentrations.
図 5a および補足図 6b および 6d に示されているように、可溶性ヘパリンは武漢変異体の三量体 S タンパク質に対して中程度の有効性を持つ受容体として機能し、可溶性ヘパリン濃度が高くてもタンパク質の大部分は表面から除去できませんでした。
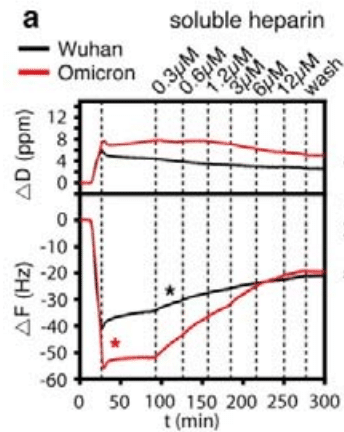
In remarkable contrast to the Wuhan trimeric S-protein and the Omicron RBD in the same experimental setup, the trimeric Omicron S-proteins desorbed much faster from the QCM-D sensor surface, even at low heparin concentrations of 0.3 μM (Figure 5a, Supplementary Figure 6c and 6e).
同じ実験設定での武漢三量体 S タンパク質とオミクロン RBD とは対照的に、三量体オミクロン S タンパク質は、0.3 μM という低いヘパリン濃度でも QCM-D センサー表面からはるかに速く脱着しました (図 5a、補足図 6c および 6e)。
Overall, a larger fraction of the Omicron trimeric S-protein could be displaced by heparin than for the Wuhan variant, despite the opposite behavior of the respective monomeric RBDs.
全体として、それぞれの単量体 RBD の挙動が逆であるにもかかわらず、オミクロン三量体 S タンパク質のより大きな割合が、武漢変異体よりもヘパリンによって置換される可能性がある。
A second important observation was that the association of the trimeric Omicron S-protein with the heparin film on the sensor was more effective, as evidenced by the decreased Kd (Supplementary Figure 6a) and by the larger -ΔF of the trimeric Omicron S-protein compared to that of the Wuhan variant (20% more protein binding), despite the same concentrations and loading times used for both proteins (Figure 5a).
2 つ目の重要な観察結果は、両方のタンパク質に同じ濃度とロード時間を使用したにもかかわらず (図 5a)、Kd の減少 (補足図 6a) と武漢変異体と比較して三量体オミクロン S タンパク質の -ΔF が大きい (タンパク質結合が 20% 多い) ことからわかるように、センサー上のヘパリン膜とオミクロン三量体 S タンパク質の結合がより効果的であったことである。
Possibly, this observation can be explained by increased electrostatic potential of the Omicron S-protein that attracts the diffusing protein close to the heparin layer prior to binding (a process called “electrostatic steering”).
おそらく、この観察結果は、結合前に拡散タンパク質をヘパリン層の近くに引き寄せるオミクロンSタンパク質の静電ポテンシャルの増加によって説明できる(「静電ステアリング」と呼ばれるプロセス)。
Finally, trimeric S-protein association increased ΔD, which may have resulted from the larger molecular size of the trimers (410.1 kDa) if compared to the monomeric RBDs (26.5 kDa).
最後に、三量体 S タンパク質の会合により ΔD が増加しましたが、これは三量体の分子サイズ (410.1 kDa) が単量体 RBD (26.5 kDa) よりも大きいことが原因である可能性があります。
Indeed, complementary fluorescence recovery after photobleaching (FRAP) assays suggest that the RBDs and the trimeric S-proteins share the ability to cross-link heparin, as evidenced by a reduced 2D diffusion rate of fluorescently labeled heparin-coupled streptavidin on the SLB by the trimeric S-proteins (Figure 5b).
実際、光退色後の相補的蛍光回復 (FRAP) アッセイでは、三量体 S タンパク質による SLB 上の蛍光標識ヘパリン結合ストレプトアビジンの 2D 拡散速度の低下によって証明されるように、RBD と三量体 S タンパク質はヘパリンを架橋する能力を共有していることが示唆されています (図 5b)。
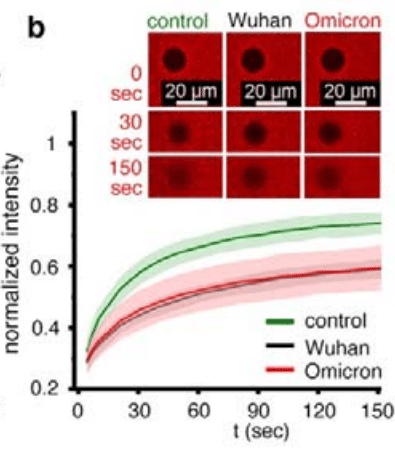
Next, we passed increasing amounts of soluble PPS on top of the heparin-associated trimeric S-proteins of the Wuhan and Omicron variants.
次に、武漢およびオミクロン変異体のヘパリン結合三量体 S タンパク質の上に可溶性 PPS を徐々に流しました。
As shown in Figure 5c, soluble PPS served as a more effective acceptor of the trimeric Wuhan and Omicron S-proteins than heparin at the same concentration, leading to much faster detachment from the sensor surface (Supplementary Figure 6b and 6d).
図 5c に示すように、可溶性 PPS は、同じ濃度のヘパリンよりも武漢およびオミクロン三量体 S タンパク質のより効果的な受容体として機能し、センサー表面からの剥離がはるかに速くなりました (補足図 6b および 6d)。
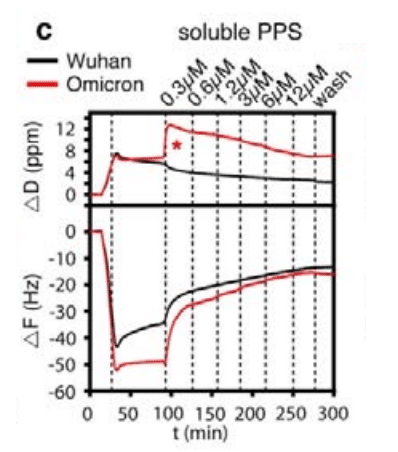
Notably, even the lowest concentrations of PPS rapidly increased F as a result of trimeric S-protein desorption from the sensor surface and its association with the highly negatively charged soluble acceptor.
注目すべきは、PPS の最低濃度でも、三量体 S タンパク質がセンサー表面から脱離し、非常に負に帯電した可溶性受容体と結合した結果として、F が急速に増加したことです。
However, we also found that trimeric S-protein desorption remained incomplete even in the presence of increased amounts of PPS, as observed before for heparin (Figure 5a).
しかし、我々はまた、以前にヘパリンで観察されたように、PPS の量が増加しても三量体 S タンパク質の脱着が不完全なままであることも発見しました (図 5a)。
This result suggests that the trimeric S-protein associates with the heparin film in two distinct modes, one mode allowing direct switching to soluble acceptors and the other mode representing less reversible binding to the sensor surface.
この結果は、三量体Sタンパク質がヘパリンフィルムと2つの異なるモードで結合することを示唆しています。1つのモードでは可溶性受容体への直接切り替えが可能で、もう1つのモードではセンサー表面への可逆性が低い結合が表されます。
We found that the evolution of the trimeric Omicron S-protein promoted the former binding mode over the latter (more static) binding mode, as the desorption of the trimeric Omicron S-protein by soluble PPS was accelerated more than that of the trimeric Wuhan S-protein (Figure 5c, Supplementary Figure 6c and 6e).
我々は、三量体オミクロン S タンパク質の進化により、後者の (より静的な) 結合モードよりも前者の結合モードが促進されたことを発見しました。可溶性 PPS による三量体オミクロン S タンパク質の脱着は、三量体武漢 S タンパク質の脱着よりも加速されました (図 5c、補足図 6c および 6e)。
Taken together, trimeric VOC S-proteins have evolved to improve their ability to directly switch between HS chains, i.e. to surf the chains without the need to first detach from them, despite their isolated RBDs evolving in the opposite direction - possibly because there is no evolutionary pressure on the RBD alone, only on the functional surface trimer.
まとめると、三量体 VOC S タンパク質は、HS 鎖間を直接切り替える能力、つまり、最初に鎖から分離する必要なく鎖をサーフィンする能力を向上させるように進化しました。これは、分離された RBD が反対方向に進化しているにもかかわらずです。これは、RBD のみに進化圧力がかからず、機能的な表面三量体にのみ影響するためと考えられます。
We propose that this allows for persistent ECM binding of trimeric S-proteins and their ability to move within the ECM, allowing the virus to effectively search the cell surface for the ACE2 receptor.
これにより、三量体 S タンパク質の持続的な ECM 結合と ECM 内での移動が可能になり、ウイルスが細胞表面で ACE2 受容体を効果的に検索できるようになると私たちは考えています。
In support of this concept, we also found that preincubation of the trimeric Omicron S-protein with 100 nM heparin or PPS greatly delayed its association with the heparin functionalized sensor surface, as evidenced by a greatly reduced -ΔF.
この概念を裏付けるものとして、三量体オミクロンSタンパク質を100 nMヘパリンまたはPPSでプレインキュベートすると、ヘパリン機能化センサー表面との結合が大幅に遅れることもわかりました。これは、-ΔFが大幅に減少したことからも明らかです。
As shown in Figure 5d, preincubation with PPS inhibited the binding of trimeric Omicron S-proteins to surface-bound heparin more than heparin, and the effect was stronger than that seen for the trimeric Wuhan S-proteins (Supplementary Figure 6f and 6g).
図 5d に示すように、PPS でプレインキュベートすると、表面に結合したヘパリンに対する三量体オミクロン S タンパク質の結合がヘパリンよりも阻害され、その効果は三量体武漢 S タンパク質で見られたものよりも強力でした (補足図 6f および 6g)。
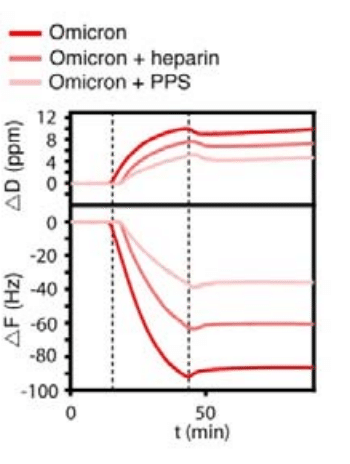
Inspired by these results, suggesting that soluble PPS may have the potential to desorb SARS-CoV-2 from the ECM (Figure 5c) and then remain associated with the viral surface to inhibit rebinding to cellular surfaces (Figure 5d), we tested next whether PPS treatment is a potential pharmaceutical approach to reduce cellular infection specifically by the Omicron VOC.
これらの結果から、可溶性PPSはSARS-CoV-2をECMから脱着させる可能性があり(図5c)、その後ウイルス表面と結合したまま細胞表面への再結合を阻害する可能性があることが示唆されました(図5d)。 次に、PPS処理がオミクロンVOCによる細胞感染を減らすための潜在的な薬理学的アプローチであるかどうかをテストしました。
PPS is the most potent inhibitor of Omicron in SARS-CoV-2 plaque-forming assays
PPSはSARS-CoV-2プラーク形成アッセイにおいてオミクロンの最も強力な阻害剤である
We have previously shown that both heparin and PPS inhibit SARS-CoV-2 infection in Vero cells.
我々は以前、ヘパリンとPPSの両方がVero細胞におけるSARS-CoV-2感染を阻害することを示した。
However, these studies were performed with the very first variant of SARS-CoV-2 that was predominant in Europe in early 2020 (this strain is characterized by a D614G mutation) in the S-protein.
しかし、これらの研究は、2020年初頭にヨーロッパで優勢だったSARS-CoV-2の最初の変異体(この株はSタンパク質のD614G変異を特徴とする)で行われた。
We have now extended the study of heparin and PPS activity against the Omicron variant by testing both compounds side-by-side in a plaque reduction assay.
我々は現在、プラーク減少アッセイで両化合物を並行して試験することにより、オミクロン変異体に対するヘパリンとPPSの活性の研究を拡大しています。
For this purpose, heparin and PPS were tested against a fixed amount of virus, i.e. 50 plaque forming units (PFU), previously titered in Vero cells.
この目的のために、ヘパリンとPPSを、Vero細胞で以前に力価測定された一定量のウイルス、すなわち50プラーク形成単位(PFU)に対して試験した。
As shown in Figure 6a and 6d, heparin, and, even more so, PPS, significantly inhibited D614G and Omicron plaque formation by reducing the number of plaques to 50% or more when cells were pretreated.
図6aと6dに示すように、細胞を前処理すると、ヘパリン、さらにはPPSが、プラークの数を50%以上に減らすことで、D614Gとオミクロンのプラーク形成を著しく阻害した。
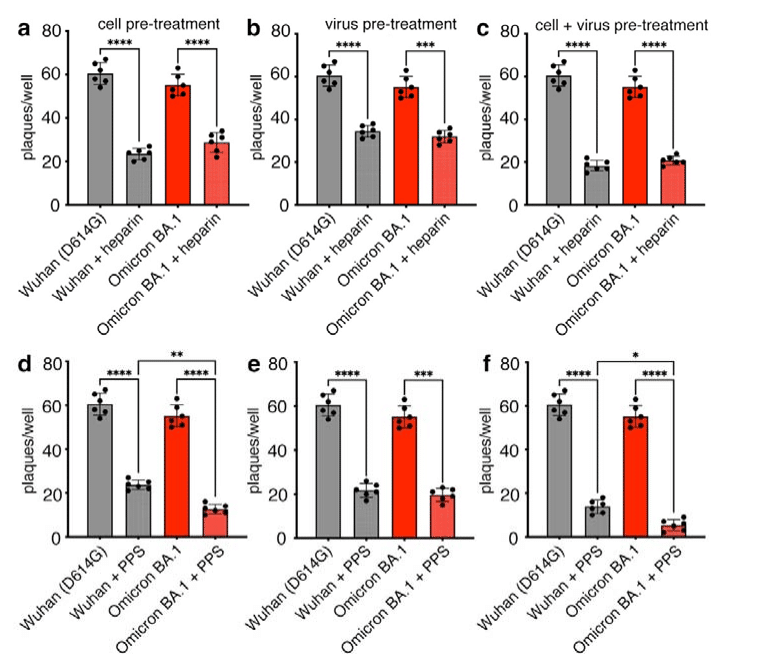
The same effect was reproduced by pre-treating the virus with both heparin and PPS (Figure 6b and 6e).
ウイルスをヘパリンとPPSの両方で前処理した場合も同じ効果が再現されました(図6bと6e)。
However, treating both the virus and cells (cell + virus pre-treatment) with either heparin or PPS was more efficient than single treatment, with PPS reducing plaque formation to almost 90% (Figure 6c and 6f).
しかし、ウイルスと細胞の両方をヘパリンまたはPPSで処理した場合(細胞+ウイルス前処理)は、単独処理よりも効率的で、PPSはプラーク形成をほぼ90%減少させました(図6cと6f)。
These results show that the higher number of mutations in the RBD of the Omicron S-protein than D614G maintain or even increase the inhibitory effect of heparin and PPS on viral infection; PPS showing highest efficiency in the inhibition of cellular infection by the Omicron BA.1 variant.
これらの結果は、D614G よりも Omicron S タンパク質の RBD の変異数が多いと、ヘパリンと PPS によるウイルス感染の阻害効果が維持されるか、またはさらに増加することを示しています。PPS は、Omicron BA.1 変異体による細胞感染の阻害において最も高い効率を示しています。
Discussion:
Rapid and specific interactions between the SARS-CoV-2 S-protein and largely negatively charged ACE2 receptors are fundamental to cellular infection.
SARS-CoV-2 Sタンパク質と主に負に帯電したACE2受容体との間の迅速かつ特異的な相互作用は、細胞感染の基本です。
It is therefore not surprising that the S-protein, and in particular the RBD, showed a steady increase in positive charge to increase binding that was remarkably accelerated when the Delta variant was leading the pandemic.
したがって、Sタンパク質、特にRBDが正電荷の着実な増加を示して結合を増強し、デルタ変異体がパンデミックを主導していたときにそれが著しく加速したことは驚くべきことではありません。
It was quickly found that with this increase in charge, the binding affinity between spike VOCs and the negatively charged cell surface HS increased as well.
この電荷の増加に伴い、スパイク VOC と負に帯電した細胞表面 HS 間の結合親和性も増加することがすぐに判明しました。
Remapping the positive charge and optimizing the electrostatic S-protein interactions with both HS and ACE2 was therefore a major driver in the evolution of SARS-CoV-2 into one of the most infectious viruses in human history.
したがって、正電荷の再マッピングとHSおよびACE2との静電的Sタンパク質相互作用の最適化は、SARS-CoV-2が人類史上最も感染力の強いウイルスの1つに進化する主な要因でした。
In our experimental work, we used QCM-D to characterize the effect of VOC RBD and trimeric S-protein evolution on the binding of the cellular glycocalyx using a heparin-functionalized lipid bilayer as a proxy.
私たちの実験では、QCM-Dを使用して、ヘパリン機能化脂質二重層をプロキシとして使用し、VOC RBDと三量体Sタンパク質の進化が細胞グリコカリックスの結合に与える影響を特徴付けました。
The first important result of our QCM-D analyses is that the RBDs of the Wuhan, Delta and Omicron variants share the ability to cross-link at least two heparin chains on the functionalized surface, and the RBDs of the latest SARS-CoV-2 variants have a stronger propensity to cross-link heparin.
QCM-D 分析の最初の重要な結果は、武漢、デルタ、オミクロン変異体の RBD は、機能化された表面で少なくとも 2 つのヘパリン鎖を架橋する能力を共有しており、最新の SARS-CoV-2 変異体の RBD はヘパリンを架橋する傾向がより強いことです。
Consistent with this, mean binding energies with a hexasaccharide of -18 kcal/mol (Wuhan) increased to -27 kcal/mol (Delta) and to -37 kcal/mol (Omicron).
これと一致して、六糖類との平均結合エネルギーは -18 kcal/mol (武漢) から -27 kcal/mol (デルタ) および -37 kcal/mol (オミクロン) に増加しました。
We show that one consequence of these evolved properties is a reduction in the off-rate of the RBD/HS interaction, as it is mediated by multivalent interactions at multiple sites that are unlikely to unbind simultaneously (a process called the multivalent effect, Figure 7a).
これらの進化した特性の 1 つの結果として、RBD/HS 相互作用のオフ レートが低下することが示されています。これは、同時に解離する可能性が低い複数のサイトでの多価相互作用によって媒介されるためです (多価効果と呼ばれるプロセス、図 7a)。
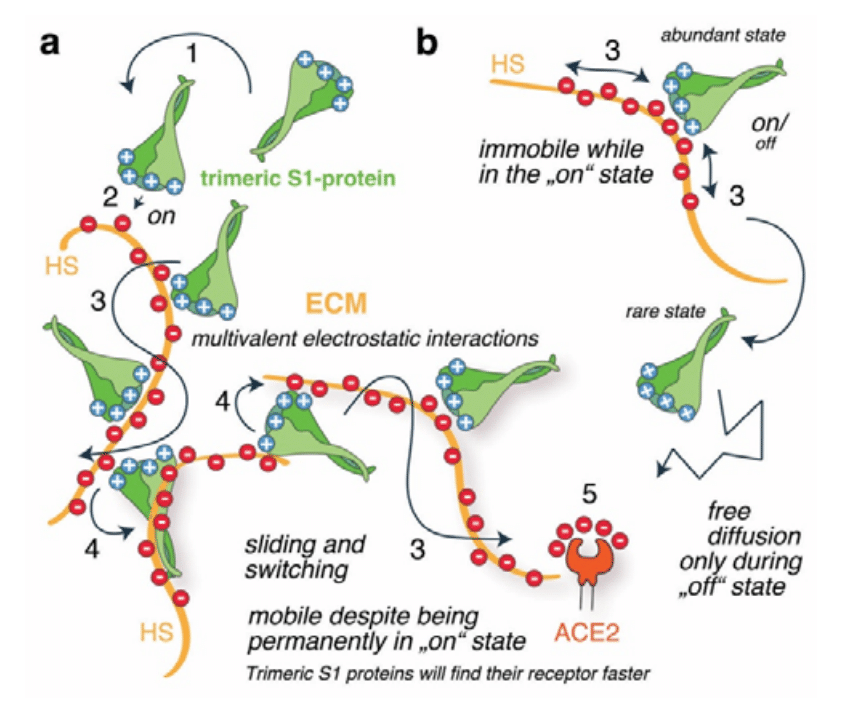
In the in vivo situation, such evolutionary optimization of multivalent HS binding could translate into more stable interactions, confining the virus to the site of initial ECM contact and reduce its spread by fluid flow and diffusion to more distant sites.
生体内では、多価 HS 結合のこのような進化的最適化により、より安定した相互作用が実現し、ウイルスは最初の ECM 接触部位に閉じ込められ、流体の流れと拡散によるウイルスの拡散が軽減されます。
This, in turn, may be a contributing factor to the altered pathophysiology of SARS-CoV-2, which began as lower respiratory tract infections with lower infectivity in the earlier variants and developed into a predominantly upper respiratory tract infection with higher infectivity in the later variants.
これは、初期の変異体では感染力の低い下気道感染症として始まり、後の変異体では感染力の高い主に上気道感染症に発展した SARS-CoV-2 の病態生理の変化の一因である可能性があります。
This evolution raises the question of whether the reduced koff of the S-protein/HS interaction conflicts with the requirement for viral movement at the cell surface and ACE2 binding.
この進化により、S タンパク質/HS 相互作用の koff の低下が、細胞表面でのウイルスの移動および ACE2 結合の必要性と矛盾するかどうかという疑問が生じます。
From the viral evolutionary perspective, an ideal situation would be enhanced binding near the cell surface, combined with an enhanced ability to migrate along and/or between HS chains to locate ACE2 receptors faster.
ウイルスの進化の観点から見ると、理想的な状況は、細胞表面近くでの結合が強化され、HS鎖に沿っておよび/またはHS鎖間を移動する能力が強化され、ACE2受容体をより早く見つけられるようになることです。
Therefore, the virus ideally evolves its S-protein surface to optimize the initial binding strength (affinity) and the half-life of the interaction such that it remains on the cell surface, and at the same time the ability to migrate along and between HS chains by keeping the interaction dynamic enough.
したがって、ウイルスは理想的にはSタンパク質表面を進化させ、初期の結合強度(親和性)と相互作用の半減期を最適化して細胞表面に留まると同時に、相互作用を十分に動的に保つことでHS鎖に沿っておよびHS鎖間を移動する能力も向上させます。
We show that, counterintuitively, these are not conflicting modes, since the increased positive charge of trimeric VOC S-proteins also enhances their ability to switch directly between heparin chains (Figure 7).
直感に反して、これらは矛盾するモードではないことが示されています。なぜなら、三量体 VOC S タンパク質の正電荷の増加により、ヘパリン鎖間で直接切り替える能力も強化されるからです (図 7)。
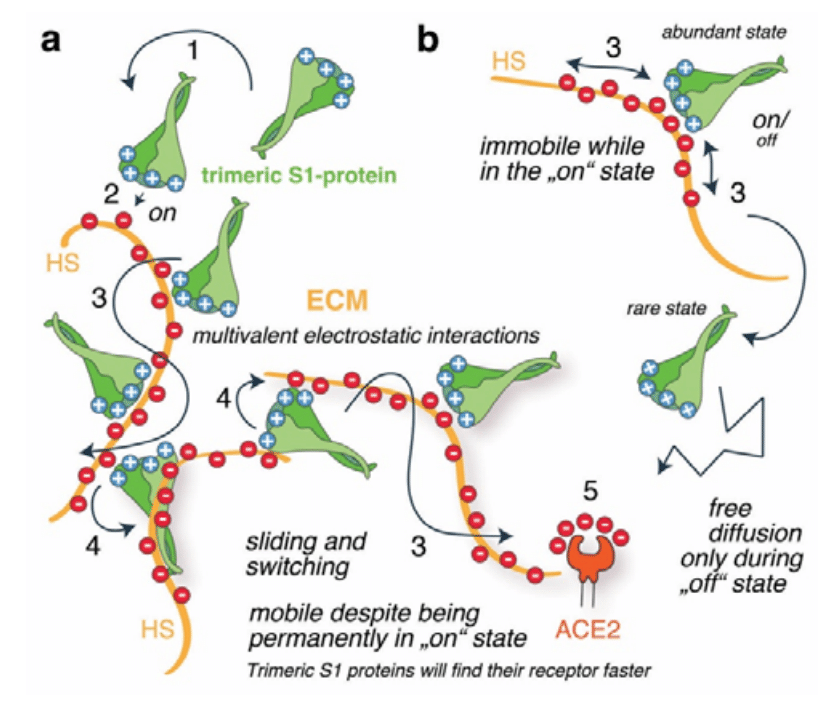
This important aspect of dynamic HS interactions is fundamentally different between the RBDs and the trimeric S-proteins and has apparently undergone evolutionary optimization.
HS の動的相互作用のこの重要な側面は、RBD と三量体 S タンパク質の間で根本的に異なり、進化の最適化を受けているようです。
In contrast to the RBD and the trimeric S-proteins of the Wuhan variant, which show similar rates of (low) detachment from the heparin film, regardless of the presence or absence of soluble acceptors, the trimeric Omicron S-protein – in contrast to the RBD – desorbs much faster in the presence of soluble acceptors.
可溶性受容体の有無にかかわらず、ヘパリン膜からの(低い)剥離速度が同程度であるRBDと武漢変異株の三量体Sタンパク質とは対照的に、 三量体オミクロンSタンパク質は、RBDとは対照的に、可溶性受容体が存在する場合、はるかに速く脱着します。
We explain this by the multivalency of the S-protein/heparin interaction possibly combined with conformational switching of the trimeric S-protein.
私たちはこれを、Sタンパク質/ヘパリン相互作用の多価性と、三量体Sタンパク質の立体配座の切り替えとの組み合わせによって説明しています。
Indeed, the multivalent effect posits that trimeric S-protein/HS interactions consist of the sum of many binding modes and sites that are linked and that can partially – but not completely – unbind in order to engage a new interaction.
実際、多価効果は、三量体 S タンパク質/HS 相互作用が、連結され、新しい相互作用を開始するために部分的に(ただし完全には)解離できる多くの結合モードと結合部位の合計から構成されていると仮定しています。
This requires that there must be at least one of several possible interactions between the two molecules at any time, and that the situation is dynamic both in binding terms and conformational terms.
これには、2 つの分子の間には常に複数の可能な相互作用のうち少なくとも 1 つが存在し、結合の面でも構造の面でも状況が動的であることが必要です。
In vivo, this mechanism could translate into faster mucosal diffusion of the VOCs within the upper airway to the ACE2 target on the epithelium to facilitate efficient infection in the upper airway epithelial cells, whereas the delayed ACE2 association of the Wuhan variant may have facilitated subsequent passive transport by diffusion or fluid and mucus flow into the lower airway.
生体内では、このメカニズムにより、上気道内の VOC が粘膜上皮の ACE2 標的に速く拡散し、上気道上皮細胞への効率的な感染が促進される可能性があります。 一方、武漢変異株の ACE2 との結合が遅れたことにより、拡散または体液と粘液の下気道への流入によるその後の受動輸送が促進された可能性があります。
Indeed, trimeric S-protein desorption of the Omicron VOC is further enhanced when the negative charge of the soluble acceptor molecule (PPS) is increased.
実際、可溶性受容体分子(PPS)の負電荷が増加すると、オミクロンVOCの三量体Sタンパク質の脱着がさらに促進されます。
This result indicates that the interaction between trimeric S-proteins and the HS is mainly charge-based and non-specific, i.e. predominantly electrostatic in nature.
この結果は、三量体Sタンパク質とHSの相互作用が主に電荷ベースで非特異的、つまり主に静電気の性質であることを示しています。
This raises the interesting possibility that viral surfing may be directed from sites of low HS charge to sites where HS sulfation is increased, and that different extents of lung HS biosynthesis and sulfation may influence individual susceptibility to SARS-CoV-2 infection.
このことから、ウイルスサーフィンがHS電荷の低い部位からHS硫酸化が増加した部位に向けられる可能性があり、肺HS生合成と硫酸化の程度の違いがSARS-CoV-2感染に対する個人の感受性に影響を与える可能性があるという興味深い可能性が浮かび上がります。
Indeed, an important recent publication describes that the composition of human lung HS varies widely among individuals depending on sex, age, and health status, and that compositional differences among samples affected chemokine binding affinities to varying degrees.
実際、最近の重要な出版物では、ヒト肺HSの組成は性別、年齢、健康状態に応じて個人間で大きく異なり、サンプル間の組成の違いがケモカイン結合親和性にさまざまな程度で影響を及ぼしたと説明されています。
It is therefore possible that the binding of the virus to the glycocalyx and its movement through the ECM varies between individuals, which may be a factor contributing to the observed differences in disease susceptibility and severity between patients.
したがって、ウイルスのグリコカリックスへの結合と ECM を介した移動は個人によって異なる可能性があり、これが患者間で観察された疾患感受性と重症度の差に寄与する要因である可能性があります。
We also note that the evolutionary optimization described here, and its possible consequences in vivo, may not be restricted to SARS-CoV-2 infection but may also apply to other HS-binding virus families.
また、ここで説明した進化的最適化と、その生体内での可能性のある結果は、SARS-CoV-2 感染に限定されず、他の HS 結合ウイルス ファミリーにも適用される可能性があることにも留意してください。
In a final set of experiments, we exploited the evolutionary optimized electrostatic S-protein/HS interactions as a potential druggable target.
最後の一連の実験では、進化的に最適化された静電的 S タンパク質/HS 相互作用を潜在的な薬物標的として利用しました。
It has been shown previously that heparin and PPS inhibit viral interaction with cellular HS and infection to a similar extent: The IC50 of PPS against the RBD of the Wuhan variant was determined to be ~35 nM and the IC50 of heparin against the RBD was determined to be 56 nM.
ヘパリンとPPSは、ウイルスと細胞HSとの相互作用および感染を同程度に阻害することが以前に示されています。武漢変異株のRBDに対するPPSのIC50は約35 nM、RBDに対するヘパリンのIC50は56 nMと決定されました。
Treatment of African green monkey Vero kidney epithelial cells (Vero E6 cells) with heparinases to reduce cell surface HS also reduced the percentage of infected cells by ~5-fold, demonstrating the importance of initial HS contact for SARS-CoV-2 infection.
アフリカミドリザルのベロ腎上皮細胞(ベロE6細胞)をヘパリナーゼで処理して細胞表面のHSを減らすと、感染細胞の割合が約5倍減少し、SARS-CoV-2感染における最初のHS接触の重要性が実証されました。
Extending these previous studies, we asked whether the accumulation of positive charge during S-protein evolution altered the ability of heparin or PPS to inhibit cell surface binding and infection.
これらの以前の研究を拡張して、Sタンパク質の進化中に正電荷が蓄積すると、ヘパリンまたはPPSの細胞表面への結合と感染を阻害する能力が変化するかどうかを調べた。
PPS has previously been shown to inhibit Wuhan variant invasion in experiments involving all three forms of addition; PPS added to cells, to virus, and when added to both before mixing.
PPSは、細胞、ウイルス、および混合前に両方に添加した場合の3つの添加形態すべてを含む実験で、武漢変異体の侵入を阻害することが以前に示された。
In these experiments, the inhibitory capacity of PPS was equal to or better than that of heparin.
これらの実験では、PPSの阻害能力はヘパリンと同等かそれ以上でした。
We confirmed this finding under all three conditions and also found that heparin inhibited the infection of Vero cells by SARS-CoV-2 Wuhan and Omicron variants to a similar extent.
我々はこの発見を3つの条件すべてで確認し、ヘパリンがSARS-CoV-2武漢変異体とオミクロン変異体によるVero細胞の感染を同程度に阻害することも発見した。
However, we also found that cell pretreatment and cell and virus pre-treatment with PPS inhibited infection by the Omicron variant significantly more than infection by the Wuhan variant, and cell and virus pre-treatment almost completely inhibited the Omicron variant.
しかし、我々はまた、PPSによる細胞前処理および細胞とウイルスの前処理は、武漢変異体による感染よりもオミクロン変異体による感染を著しく阻害し、細胞とウイルスの前処理はオミクロン変異体をほぼ完全に阻害したことも発見した。
This indicates that the accumulation of additional positive charge on the Omicron S-protein has increased its susceptibility to highly negatively charged soluble inhibitors, which are likely to act on three mechanisms of SARS-CoV-2 infection: allosteric or charge-based inhibition of virus binding to ACE2, enhanced competitive inhibition with binding to host HS coreceptors and, possibly, its desorption from cellular HS of lesser charge and prevention of spike cleavage at polybasic sites.
これは、オミクロンSタンパク質上の追加の正電荷の蓄積により、高度に負に帯電した可溶性阻害剤に対する感受性が高まったことを示しており、これはSARS-CoV-2感染の3つのメカニズム、すなわちACE2へのウイルス結合のアロステリックまたは電荷ベースの阻害、宿主HS共受容体への結合による競合阻害の強化、そしておそらく、より電荷の少ない細胞HSからの脱離と多塩基部位でのスパイク切断の防止に作用する可能性がある。
Together with the fact that PPS has about 10 times lower anticoagulant potency than heparin and no anti-factor Xa activity, the administration of PPS, even at high doses, may therefore allow its use as a preventive measure, i.e. in nasal sprays to inhibit viral attachment to the glycocalyx, to increase viral desorption and to decrease cellular rebinding, in particular of the Omicron VOCs and future variants.
PPS はヘパリンよりも抗凝固作用が約 10 倍低く、抗第 Xa 因子活性がないことと合わせて、高用量であっても PPS を投与すると、予防策として、つまり鼻スプレーでグリコカリックスへのウイルス付着を阻害し、ウイルス脱着を促進し、特にオミクロン VOC および将来の変異体の細胞再結合を減らすために使用できるようになる可能性があります。
以下省略。
この記事が気に入ったらサポートをしてみませんか?