ヒト感染症における XBB と JN.1 の体液性免疫原性の比較
Humoral immunogenicity comparison of XBB and JN.1 in human infections
ヒト感染症における XBB と JN.1 の体液性免疫原性の比較
元→2024.04.19.590276v1.full.pdf (biorxiv.org)
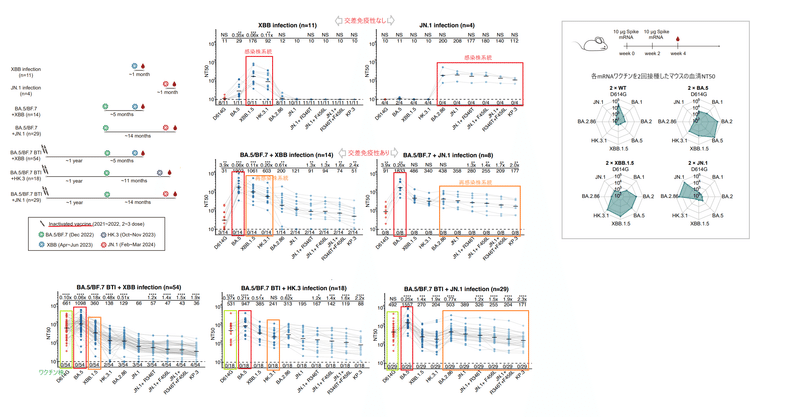
Abstract
The ongoing evolution of SARS-CoV-2 continues to challenge the global immune barrier established by infections and vaccine boosters.
SARS-CoV-2 の進行中の進化は、感染とワクチンブースターによって確立された世界的な免疫障壁に挑戦し続けています。
Recently, the emergence and dominance of the JN.1 lineage over XBB variants have prompted a reevaluation of current vaccine strategies.
最近、JN.1 系統の出現と XBB 変異体に対する優勢性により、現在のワクチン戦略の再評価が促されています。
Despite the demonstrated effectiveness of XBB-based vaccines against JN.1, concerns persist regarding the durability of neutralizing antibody (NAb) responses against evolving JN.1 subvariants.
JN.1 に対する XBB ベースのワクチンの有効性が実証されているにもかかわらず、進化する JN.1 亜変異体に対する中和抗体 (NAb) 応答の持続性に関する懸念は依然として残っています。
In this study, we compared the humoral immunogenicity of XBB and JN.1 lineage infections in human subjects with diverse immune histories to understand the antigenic and immunogenic distinctions between these variants.
この研究では、これらの変異体の抗原性と免疫原性の違いを理解するために、多様な免疫歴を持つヒト被験者における XBB および JN.1 系統感染の体液性免疫原性を比較しました。
Similar to observations in naïve mice, priming with XBB and JN.1 in humans without prior SARS-CoV-2 exposure results in distinct NAb responses, exhibiting minimal cross-reactivity.
ナイーブマウスでの観察と同様に、事前に SARS-CoV-2 に曝露されていないヒトで XBB および JN.1 でプライミングすると、明確な NAb 応答が生じ、最小限の交差反応性が示されます。
Importantly, breakthrough infections (BTI) with the JN.1 lineage induce 5.9-fold higher neutralization titers against JN.1 compared to those induced by XBB BTI.
重要なことに、JN.1 系統によるブレイクスルー感染 (BTI) は、XBB BTI によって誘導されるものと比較して、JN.1 に対して 5.9 倍高い中和力価を誘導します。
We also observed notable immune evasion of recently emerged JN.1 sublineages, including JN.1+R346T+F456L, with KP.3 showing the most pronounced decrease in neutralization titers by both XBB and JN.1 BTI sera.
また、JN.1+R346T+F456L など、最近出現した JN.1 亜系統の顕著な免疫回避も観察され、KP.3 は XBB 血清と JN.1 BTI 血清の両方による中和力価の最も顕著な低下を示しました。
These results underscore the challenge posed by the continuously evolving SARS-CoV-2 JN.1 and support the consideration of switching the focus of future SARS-CoV-2 vaccine updates to the JN.1 lineage.
これらの結果は、進化を続けるSARS-CoV-2 JN.1によってもたらされる課題を強調しており、将来のSARS-CoV-2ワクチン更新の焦点をJN.1系統に切り替える検討を裏付けるものである。
Main
Since the emergence of the SARS-CoV-2 BA.2.86 lineage in July 2023, its subvariants, especially JN.1, have continued to circulate and evolve rapidly, outcompeting the previously prevalent XBB subvariants.
2023 年 7 月に SARS-CoV-2 BA.2.86 系統が出現して以来、そのサブバリアント、特に JN.1 は循環し続け、急速に進化し、以前に流行していた XBB サブバリアントを上回りました。
By March 2024, the JN.1 lineage accounted for over 93% of newly observed sequences (Extended Data Fig. 1a).
2024 年 3 月までに、JN.1 系統は新たに観察された配列の 93% 以上を占めました (拡張データ図 1a)。
BA.2.86 and JN.1 have convergently accumulated mutations on the receptor-binding domain (RBD) of the viral spike glycoprotein, including R346S/T, F456L/V, and A475V/S (Extended Data Fig. 1b).
BA.2.86およびJN.1は、R346S/T、F456L/V、およびA475V/Sを含む、ウイルススパイク糖タンパク質の受容体結合ドメイン(RBD)上に集中的に蓄積した突然変異を持っています(拡張データ図1b)。
These subvariants, represented by JN.1+R346T (such as JN.1.18, JN.1.13.1, and JN.1.24), JN.1+F456L (such as JN.1.16 and JN.1.11.1), and their combination, JN.1+R346T+F456L (socalled “FLiRT” variants), exhibit a remarkable growth advantage during their recent circulation (Extended Data Fig. 1a-b).
これらのサブバリアントは、JN.1+R346T (JN.1.18、JN.1.13.1、JN.1.24 など)、JN.1+F456L (JN.1.16 や JN.1.11.1 など) およびその組み合わせ、JN.1+R346T+F456L (いわゆる「FLiRT」亜種)で表されます。 最近の流通中に顕著な成長利点を示しました (拡張データ図 1a-b)。
A newly detected subvariant, designated as KP.3, even carries a Q493E mutation, likely exhibiting distinct behaviors compared to the previously well-known Q493R, whose reversion increases the receptor-binding affinity.
KP.3 と名付けられた新たに検出された亜変異体は Q493E 変異さえ持っており、以前によく知られていた Q493R(その復帰は受容体結合親和性が増加) と比較して異なる挙動を示す可能性があります。
Most of these sites mutated in JN.1 subvariants are located near the receptor-binding motif (RBM) and are known to be related to antibody escape (Extended Data Fig. 1c).
JN.1 サブバリアントで変異したこれらの部位のほとんどは、受容体結合モチーフ (RBM) の近くに位置しています。 抗体エスケープに関連することが知られています (拡張データ図 1c)。
This makes it crucial to investigate their capabilities of evading the current immune barrier established by SARS-CoV-2 infections and vaccines.
このため、SARS-CoV-2感染症とワクチンによって確立された現在の免疫障壁を回避する能力を調査することが重要になっています。
Previous studies demonstrated the satisfactory capability of eliciting JN.1-effective neutralizing antibodies of the currently recommended XBB-based vaccine boosters.
以前の研究では、現在推奨されている XBB ベースのワクチン追加免疫の JN.1 に有効な中和抗体を誘発する満足のいく能力が実証されました。
However, it is important to investigate whether JN.1 immunization performs substantially better against these novel subvariants than XBB.
ただし、これらの新規亜変異体に対して JN.1 免疫化が XBB よりも実質的に優れた効果を発揮するかどうかを調査することが重要です。
Here in this manuscript, we systematically compared the humoral immune response of XBB* and JN.1 in human infections and mice vaccination against JN.1 sublineages.
この原稿では、ヒト感染および JN.1 亜系統に対するマウスのワクチン接種における XBB* と JN.1 の体液性免疫応答を系統的に比較しました。
Results suggest that future SARS-CoV-2 vaccine efforts might benefit from focusing on the JN.1 lineage instead of XBB to better counter current and emerging variants.
結果は、将来のSARS-CoV-2ワクチンの取り組みでは、XBBではなくJN.1系統に焦点を当て、より良い逆流および新興変異種に焦点を当てることが有益である可能性があることを示唆している。
Results
We collected plasma samples from seven cohorts, including individuals infected by XBB* (n=11) or JN.1 (n=4) without known previous exposure to SARS-CoV-2, those who experienced sequential infections of BA.5/BF.7 and XBB* (n=14), or BA.5/BF.7 and JN.1 (n=29), and those who received 3-dose inactivated vaccines followed by BA.5/BF.7 breakthrough infection (BTI) and then reinfected by XBB (mainly XBB+S486P), HK.3, or JN.1 (n=54, 18, 29, respectively) (Fig. 1a).
私たちは、SARS-CoV-2 への曝露歴が知られていない XBB* (n=11) または JN.1 (n=4) に感染した個人を含む 7 つのコホートから血漿サンプルを収集しました。BA.5/BF.7とXBB*の連続感染を経験した人(n=14)、またはBA.5/BF.7とJN.1(n=29)、不活化ワクチンを 3 回接種した後に BA.5/BF.7 画期的感染症(BTI)を受けた患者とその後、XBB (主に XBB+S486P)、HK.3、または JN.1 に再感染 (それぞれ n=54、18、29)した人です(図1a)。
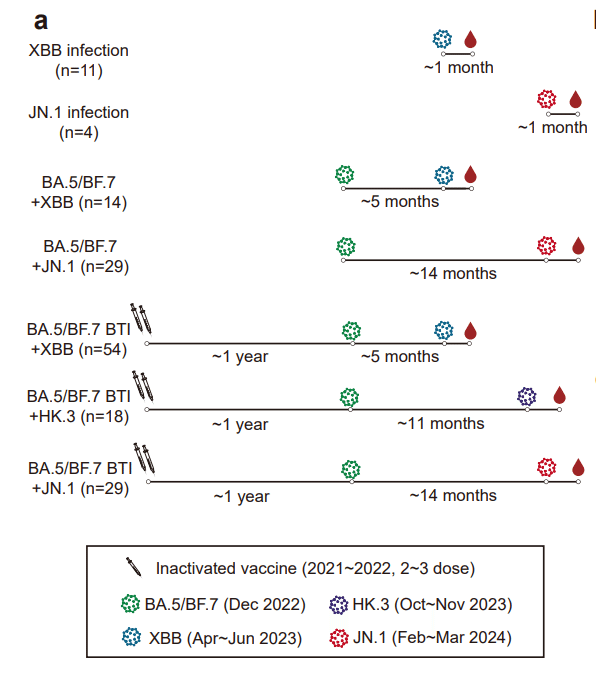
Infection strains were inferred based on the sampling time when the corresponding strain was the majority of detected sequences in the region of sample collection.
感染株は、対応する株がサンプル収集領域で検出された配列の大部分であった場合のサンプリング時間に基づいて推定されました。
SARS-CoV-2 Infection was confirmed by either antigen or PCR tests.
SARS-CoV-2 感染は、抗原検査または PCR 検査によって確認されました。
Priming with XBB and JN.1 in humans elicited distinct neutralizing antibodies without observable cross-lineage reactivity (Fig. 1b).
ヒトにおけるXBBおよびJN.1によるプライミングは、観察可能な交差系統反応性を伴わずに、明確な中和抗体を誘発した(図1b)。
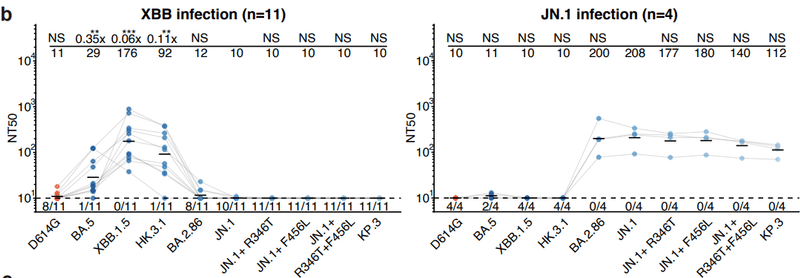
Such distinct antigenicity between XBB and JN.1 was also confirmed in mice, showing remarkable antigenic distances between these lineages (Extended Data Fig. 2a-c).
XBB と JN.1 の間のこのような明確な抗原性はマウスでも確認され、これらの系統間の顕著な抗原性の距離が示されました (拡張データ図 2a-c)。
In contrast, a prior BA.5 or BF.7 infection improved the cross-lineage reactivity of antibodies induced by XBB or JN.1 reinfection.
対照的に、以前の BA.5 または BF.7 感染は、XBB または JN.1 の再感染によって誘導された抗体の交差系統反応性を改善しました。
This suggests that BA.5/BF.7 priming could induce Omicron cross-reactive neutralizing antibodies that are effective against both XBB and JN.1 lineages (Fig. 1c).
これは、BA.5/BF.7プライミングが、XBBおよびJN.1系統の両方に対して有効なOmicron交差反応性中和抗体を誘導できることを示唆しています(図1c)。
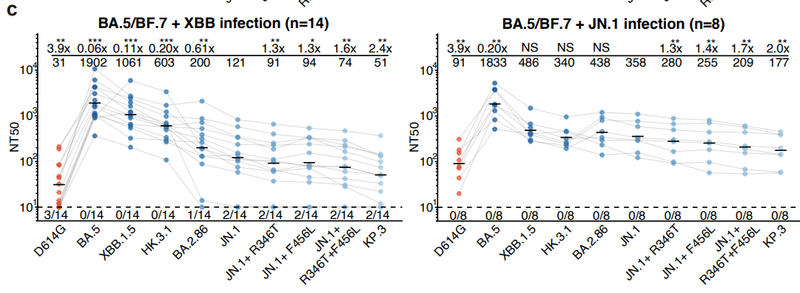
In the three BTI with reinfection cohorts, “BA.5/BF.7 BTI + XBB” elicited the lowest NT50 against JN.1 lineage variants (Fig. 1d).
再感染コホートを伴う 3 つの BTI では、「BA.5/BF.7 BTI + XBB」が JN.1 系統変異体に対して最も低い NT50 を誘発しました(図 1d)。
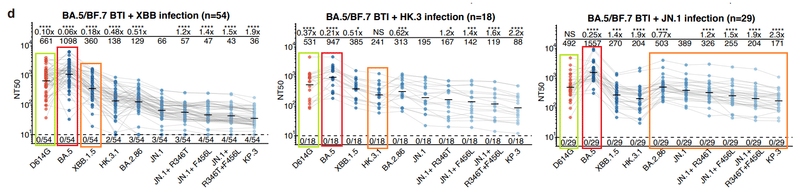
On average, JN.1 reinfection induced a 5.9-fold higher NT50 against JN.1, and a 4.7-fold higher NT50 against JN.1+R346T+F456L (“FLiRT”), compared with XBB reinfection (Fig. 1e).
平均して、JN.1再感染は、XBB再感染と比較して、JN.1に対して5.9倍高いNT50、JN.1+R346T+F456L(「FLiRT」)に対して4.7倍高いNT50を誘導しました(図1e)。
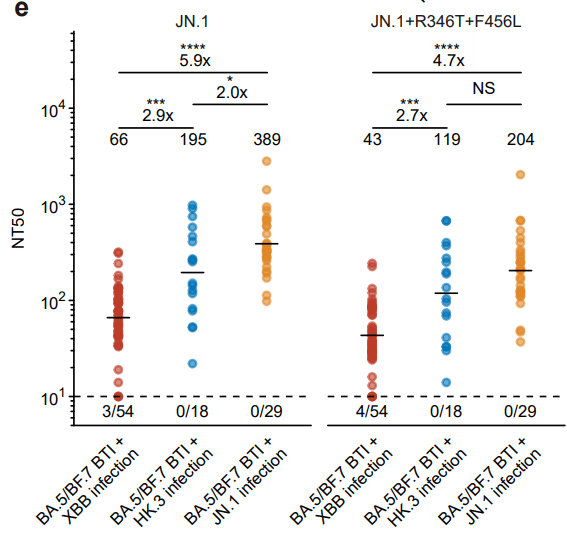
The improvement of JN.1 BTI over HK.3 BTI was less significant, possibly due to the shorter interval between infections in the XBB cohort and the immunogenicity drift from HK.3’s “FLip” mutation.
HK.3 BTI に対する JN.1 BTI の改善はあまり有意ではありませんでしたが、これはおそらく XBB コホートにおける感染間隔の短縮と、HK.3 の「FLip」変異による免疫原性の変動によるものと考えられます。
Among all five reinfection cohorts, all of the four JN.1 subvariants tested, including JN.1+R346T, JN.1+F456L, JN.1+R346T+F456L, and KP.3, exhibited notable immune evasion.
5 つの再感染コホートすべての中で、JN.1+R346T、JN.1+F456L、JN.1+R346T+F456L、および KP.3 を含む、テストした 4 つの JN.1 サブバリアントのすべてが、顕著な免疫回避を示しました。
KP.3 consistently acted as the strongest escaper, leading to a 1.9 to 2.4-fold reduction in NT50 compared to JN.1, likely due to the unprecedented Q493E mutation of KP.3.
KP.3 は一貫して最強のエスケープ剤として機能し、JN.1 と比較して NT50 が 1.9 ~ 2.4 倍減少しました。これはおそらく、KP.3 の前例のない Q493E 変異によるものと考えられます。
Antigenic cartography of our plasma neutralization data visualized the antigenic differences of SARS-CoV-2 variants.
血漿中和データの抗原地図作成により、SARS-CoV-2 変異体の抗原の違いが視覚化されました。
The antigenic map from single-exposure cohorts clearly depicted the intrinsic antigenic distances between XBB and JN.1 lineage, despite sample size limitations (Fig. 1f).
単回曝露コホートからの抗原マップは、サンプルサイズの制限にもかかわらず、XBBとJN.1系統の間の固有の抗原距離を明確に示しました(図1f)。
Samples from BTI with reinfection cohorts showed strong ancestral strain imprinting, indicated by the aggregation of points near the D614G strain (Fig. 1g).
再感染コホートを伴うBTIからのサンプルは、D614G株近くの点の集合によって示される、強力な祖先株インプリンティングを示しました(図1g)。
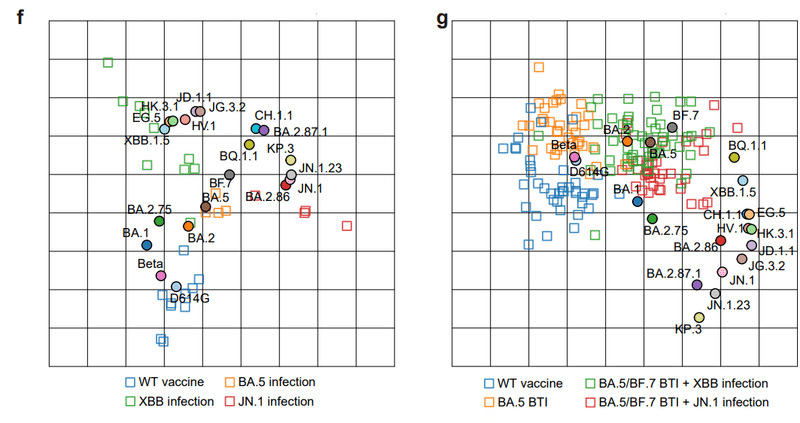
Nevertheless, the JN.1 BTI cohorts displayed closer distance to current circulating variants, supporting the idea of switching vaccine boosters to JN.1 lineages.
それにもかかわらず、JN.1 BTI コホートは現在循環している変異株との距離が近いことを示し、ワクチンブースターを JN.1 系統に切り替えるという考えを裏付けています。
Previous studies have highlighted the synergistic impact of RBD L455-F456 mutations on ACE2 receptor binding affinity.
これまでの研究では、RBD L455-F456 変異が ACE2 受容体結合親和性に及ぼす相乗的な影響が強調されています。
Given these sites are also convergently mutated in BA.2.86 lineages (especially JN.1), we tested the binding affinities of JN.1 subvariant RBD to human ACE2 (hACE2) using surface plasmon resonance (SPR).
これらの部位も BA.2.86 系統 (特に JN.1) で集中的に変異していることを考慮して、表面プラズモン共鳴 (SPR) を使用して、ヒト ACE2 (hACE2) に対する JN.1 サブバリアント RBD の結合親和性をテストしました。
Interestingly, F456L (KD=12 nM) and R346T+F456L (KD=11 nM) did not largely affect the hACE2-binding affinity of JN.1 (KD=13 nM), indicating that unlike L455F in HK.3, the dampened ACE2 affinity of JN.1 due to L455S could not be compensated by F456L.
興味深いことに、F456L (KD=12 nM) および R346T+F456L (KD=11 nM) は、JN.1 (KD=13 nM) の hACE2 結合親和性に大きな影響を与えませんでした。 これは、HK.3 の L455F とは異なり、L455S による JN.1 の ACE2 親和性の低下が F456L によって補償できないことを示しています。
Notably, another JN.1 subvariant designated as JN.1.23, which harbors K444R+Y453F mutation, showed substantially higher affinity to ACE2, potentially allowing for more immune evasive mutations in the future (Extended Data Fig. 3).
注目すべきことに、K444R+Y453F変異を保有するJN.1.23と呼ばれる別のJN.1サブバリアントは、ACE2に対して実質的に高い親和性を示し、将来的により多くの免疫回避性変異を可能にする可能性がある(拡張データ図3)。
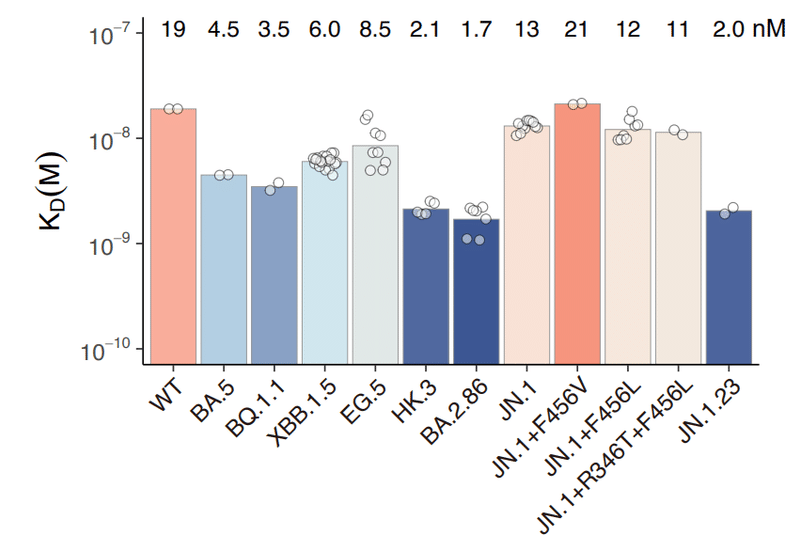
Together, our results underscore the significant antigenic distinctions between the SARS-CoV-2 XBB and JN.1 lineages and provide compelling evidence to shift the focus of vaccine booster strategies from XBB to the JN.1 lineage.
まとめると、我々の結果は、SARS-CoV-2 XBB 系統と JN.1 系統の間の抗原の重要な違いを強調し、ワクチン追加免疫戦略の焦点を XBB から JN.1 系統に移す説得力のある証拠を提供する。
Despite the notable cross-lineage reactivity elicited by XBB as a booster, boosters based on JN.1 may offer superior protection against current and forthcoming JN.1 subvariants.
ブースターとして XBB によって引き起こされる顕著な系統間反応性にもかかわらず、JN.1 に基づくブースターは、現在および今後の JN.1 サブバリアントに対して優れた保護を提供する可能性があります。
Furthermore, the ongoing evolution of JN.1 subvariants, particularly those with enhanced receptor-binding capabilities, warrants vigilant monitoring.
さらに、JN.1 サブバリアントの進行中の進化、特に受容体結合能力が強化されたサブバリアントは、注意深く監視する必要があります。
以下省略。
この記事が気に入ったらサポートをしてみませんか?