免疫学の新たな視点を探る
Exploring new perspectives in immunology
免疫学の新たな視点を探る
元→Exploring new perspectives in immunology: Cell
Summary
Several conceptual pillars form the foundation of modern immunology, including the clonal selection theory, antigen receptor diversity, immune memory, and innate control of adaptive immunity.
クローン選択理論、抗原受容体の多様性、免疫記憶、適応免疫の自然制御など、いくつかの概念的な柱が現代免疫学の基礎を形成しています。
However, some immunological phenomena cannot be explained by the current framework.
しかし、免疫学的現象の中には現在の枠組みでは説明できないものもあります。
Thus, we still do not know how to design vaccines that would provide long-lasting protective immunity against certain pathogens, why autoimmune responses target some antigens and not others, or why the immune response to infection sometimes does more harm than good.
したがって、特定の病原体に対して長期持続する防御免疫を提供するワクチンをどのように設計するか、なぜ自己免疫応答が一部の抗原を標的とし他の抗原を標的としないのか、なぜ感染に対する免疫応答が時々善よりも害を及ぼすのか、私たちはまだわかっていません。
Understanding some of these mysteries may require that we question existing assumptions to develop and test alternative explanations.
これらの謎の一部を理解するには、既存の仮定に疑問を投げかけ、別の説明を開発し、テストする必要があるかもしれません。
Immunology is increasingly at a point when, once again, exploring new perspectives becomes a necessity.
免疫学は、再び新たな視点を探求することがますます必要になる時期にきています。
Introduction
The immune system provides critical defense against pathogens, and in vertebrates, it consists of two major components—innate and adaptive.
免疫系は病原体に対する重要な防御を提供します。脊椎動物では、免疫系は 2 つの主要な要素、つまり先天的要素と適応的要素で構成されます。
The innate immune system is present in prokaryotic to eukaryotic life forms and even in giant viruses.
自然免疫システムは、原核生物から真核生物、さらには巨大ウイルスにも存在します。
The innate immune system detects various cues indicative of the pathogenic threat, including molecular structures of uniquely microbial origin.In addition, two types of lymphocytes evolved independently in jawless and jawed vertebrates, which ultimately gave rise to the adaptive immune systems.
自然免疫系は、微生物特有の起源の分子構造など、病原性の脅威を示すさまざまな合図を検出します。さらに、2 種類のリンパ球が無顎脊椎動物と有顎脊椎動物で独立して進化し、最終的に適応免疫系を生み出しました。
A major evolutionary innovation brought about by lymphocyte emergence is a distinct mode of immune recognition, which is based on antigen receptors.
リンパ球の出現によってもたらされる主要な進化的革新は、抗原受容体に基づく独特の免疫認識モードです。
This allowed lymphocytes to recognize specific microbial antigens and leverage the innate immune effector functions to eliminate pathogens through specificity, amplification, and the development of memory.
これにより、リンパ球が特定の微生物抗原を認識し、自然免疫エフェクター機能を活用して、特異性、増幅、記憶の発達を通じて病原体を排除できるようになりました。
A distinct characteristic of antigen receptors is that their specificities are not genetically determined but rather generated by several random processes, including VDJ recombination, gene conversion, base editing, and random pairing of antigen receptor subunits.
抗原受容体の明確な特徴は、その特異性が遺伝的に決定されるのではなく、VDJ 組換え、遺伝子変換、塩基編集、抗原受容体サブユニットのランダムなペアリングなどのいくつかのランダムなプロセスによって生成されることです。
The degree to which these random processes contribute to the receptor specificity dictates the degree of receptor diversity that can be achieved.
これらのランダムなプロセスが受容体の特異性にどの程度寄与するかによって、達成できる受容体の多様性の程度が決まります。
However, the diversity of antigen receptors comes at the cost of information these receptors can interpret.
ただし、抗原受容体の多様性には、これらの受容体が解釈できる情報が犠牲になります。
Thus, lymphocytes with the highest antigen receptor diversity (most αβT cells and the majority of B cells—see Box 1 for a glossary of terminology) cannot determine the source of the antigen they are specific for (including whether the antigen is self or microbial), nor can they predict where in the body they might encounter their cognate antigens.
したがって、抗原受容体の多様性が最も高いリンパ球(ほとんどのαβT細胞と大部分のB細胞。用語集についてはボックス1を参照)は、それらが特異的な抗原の起源を決定することができません(抗原が自己抗原か微生物抗原かを含む)。 また、体内のどこで同種の抗原に遭遇するかを予測することもできません。
These limitations are partially solved by several mechanisms.
これらの制限は、いくつかのメカニズムによって部分的に解決されます。
Some T and B lymphocytes specific for self-antigens are deleted in the thymus and bone marrow, respectively, during their development.
自己抗原に特異的な一部の T リンパ球と B リンパ球は、発生中にそれぞれ胸腺と骨髄で削除されます。
However, this pruning process is incomplete, allowing a subset of self-reactive lymphocytes to complete their development into mature cells.
ただし、この剪定プロセスは不完全であるため、自己反応性リンパ球のサブセットが成熟細胞への発達を完了することができます。
Activation of potentially destructive autoreactive lymphocytes is normally avoided by two mechanisms.
潜在的に破壊的な自己反応性リンパ球の活性化は、通常、2 つのメカニズムによって回避されます。
First, lymphocyte activation depends on signals generated upon microbial recognition by the innate immune system.
第一に、リンパ球の活性化は、自然免疫系による微生物の認識に応じて生成されるシグナルに依存します。
Second, activation of deleterious immune responses against self-antigens, as well as commensal microbes and non-microbial environmental antigens, is normally prevented by T regulatory (Treg) cells.
第二に、自己抗原、共生微生物および非微生物環境抗原に対する有害な免疫応答の活性化は、通常、制御性 T (Treg) 細胞によって阻止されます。
Another consequence of antigen receptor diversity is that each receptor specificity is expressed on a tiny fraction of naive lymphocytes.
抗原受容体の多様性のもう 1 つの結果は、各受容体特異性がナイーブリンパ球のごく一部で発現されることです。
This limitation leads to a need for continuous sampling of the “antigenic space” through recirculation between blood and secondary lymphoid organs—a process enabled by the lymphatic drainage system.
この制限により、血液と二次リンパ器官の間の再循環による「抗原性空間」の継続的なサンプリングの必要性が生じます。このプロセスはリンパドレナージシステムによって可能になります。
Once naive lymphocytes encounter a cognate antigen combined with instructive signals from the innate immune system (and with Treg’s blessing), they undergo clonal selection, expansion, and differentiation into effector cells.
ナイーブリンパ球は、自然免疫系からの指令シグナル(および Treg の祝福)と組み合わされた同族抗原に遭遇すると、クローン選択、増殖、およびエフェクター細胞への分化を受けます。
Additionally, a small subset of thus activated lymphocytes becomes memory cells and prepares the host for future encounters with the same pathogen.
さらに、このように活性化されたリンパ球の小さなサブセットが記憶細胞となり、宿主が将来同じ病原体に遭遇する準備をします。
Although this paradigm of how the immune system works has served the field for the past 30 years, new discoveries are increasingly hard to fit into the current framework.
免疫システムがどのように機能するかに関するこのパラダイムは、過去 30 年間この分野で役に立ってきましたが、新しい発見は現在の枠組みに適合させることがますます困難になっています。
This raises questions about some of the foundational assumptions, exceptions to the rules, and inconsistencies that would benefit from exploring new ideas and points of view.
これにより、新しいアイデアや観点を探求することで得られるいくつかの基本的な前提、ルールの例外、矛盾について疑問が生じます。
Here, we will discuss some of these issues and suggest possible perspectives to reconcile immunological phenomena that cannot be explained by the current immunological paradigm.
ここでは、これらの問題のいくつかについて議論し、現在の免疫学的パラダイムでは説明できない免疫学的現象を調和させるための可能な視点を提案します。
We will first review the requirements for immune response activation—the requisite signals and information they carry that are necessary or sufficient for the activation of different arms of immunity.
まず、免疫応答の活性化の要件、つまりさまざまな免疫部門の活性化に必要または十分な、必要なシグナルとそれらが運ぶ情報を確認します。
Next, we will discuss immune memory and suggest possible extensions of the concept based on the types of information relevant to immunity that may be stored following infections.
次に、免疫記憶について説明し、感染後に保存される可能性のある免疫に関連する情報の種類に基づいて、概念の拡張の可能性を提案します。
We then consider the current limitations of our understanding of protective immunity in the context of the basic stages of the infection cycle and the implications for vaccine development.
そして、感染サイクルの基本段階とワクチン開発への影響という観点から、防御免疫に関する理解の現在の限界を検討します。
Finally, we will explore the extended view of the immune system functions due to exaptations and suggest immune interoception as a concept in homeostasis and immunopathology.
最後に、摘出による免疫系機能の拡張された視点を探求し、恒常性と免疫病理学における概念としての免疫相互受容を提案します。
This selection of topics is certainly not comprehensive.
このトピックの選択は確かに包括的ではありません。
The ideas discussed here are our attempts to address inconsistencies in the current paradigms of immunology.
ここで議論するアイデアは、免疫学の現在のパラダイムにおける矛盾に対処するための私たちの試みです。
Our goal here is to provoke discussion that would ultimately resolve these inconsistencies.
ここでの私たちの目標は、最終的にこれらの矛盾を解決するための議論を引き起こすことです。
For, to quote Richard Feynman, it is better to “have questions that can’t be answered than answers that can’t be questioned.”
リチャード・ファインマンの言葉を借りれば、「質問できない答えがあるよりも、答えられない質問があるほうがよい」からです。
Immunogenicity
免疫原性
One of the key questions in immunology concerns the requirements for immune response activation.
免疫学における重要な問題の 1 つは、免疫応答の活性化の要件に関するものです。
What signals are necessary or sufficient to initiate the response?
応答を開始するにはどのような信号が必要または十分ですか?
The question has to be parsed by the type of immune response, as the requirements for antigen-specific versus innate immune responses are clearly different, as are the requirements for the primary versus memory responses.
抗原特異的免疫応答と自然免疫応答の要件は明らかに異なるため、この質問は免疫応答のタイプによって解析する必要があります。 一次応答と記憶応答の要件も同様です。
There are also less obvious and ill-defined differences in requirements; thus, there appear to be many more signals that are sufficient to induce T helper type 2 (Th2), compared with Th1 and Th17 responses, making the identification of the necessary signals for the former challenging.
要件には、それほど明白ではない不明確な違いもあります;したがって、Th1 および Th17 応答と比較して、T ヘルパー 2 型(Th2)を誘導するのに十分なシグナルがより多く存在すると考えられ、前者に必要なシグナルの同定が困難となっています。
Another layer of complexity is whether a given type of immune response (based on current definitions) can have different qualitative characteristics, depending on the signals that initiated it.
複雑さのもう 1 つの層は、特定のタイプの免疫応答(現在の定義に基づく) が、それを開始したシグナルに応じて異なる定性的特性を持つ可能性があるかどうかです。
For example, do we expect immune responses (against a given pathogen) to be equivalent whether they are induced by infection or live-attenuated, inactivated, mRNA, or subunit vaccines, assuming all four vaccines induce comparable antibody titers?
たとえば、(特定の病原体に対する)免疫応答が、感染によって誘導されたものであっても、生弱毒化ワクチン・不活化ワクチン・mRNAワクチン・サブユニットワクチンによって誘導されたものであっても( 4 つのワクチンすべてが同等の抗体力価を誘導すると仮定)、同等であると期待できるでしょうか?
The answer is presumably no in most cases, but we do not fully understand why and in what ways the responses are different and which characteristics of the five challenges account for these differences.
ほとんどの場合、答えはおそらくノーですが、なぜ、どのように反応が異なるのか、また 5 つの課題のどの特性がこれらの違いを説明しているのかは完全には理解できません。
One can go further and include additional variables, such as the site of infection/immunization, the antigen dose or replication rate of a pathogen, and the physical characteristics of the adjuvant.
さらに進んで、感染/免疫の部位、抗原の用量または病原体の複製速度、アジュバントの物理的特性などの追加の変数を含めることもできます。
All of these parameters have in fact been studied over the past decades, but we still lack a coherent picture of what the “rules” are relating the quantitative and qualitative characteristics of the necessary and sufficient signals with the quality of the responses they induce.
実際、これらのパラメーターはすべて過去数十年にわたって研究されてきましたが、必要かつ十分な信号の量的および定性的特性と、それらが引き起こす応答の質とをどのような「規則」が関連付けているのかについて、一貫した全体像がまだわかっていません。
This problem is especially relevant to the question of protective immunity, where our understanding is particularly limited, as discussed below.
この問題は、以下で説明するように、私たちの理解がとりわけ限られている防御免疫の問題に特に関連しています。
Before we define this problem formally, let’s consider an analogy from sensory physiology: we receive information about the outside world through several information channels, including visual, olfactory, gustatory, and auditory pathways.
この問題を正式に定義する前に、感覚生理学からの類推を考えてみましょう:私たちは、視覚、嗅覚、味覚、聴覚の経路を含むいくつかの情報チャネルを通じて外界に関する情報を受け取ります。
Each provides a different type of information, and our response is based on processing and integrating these inputs.
それぞれが異なる種類の情報を提供し、私たちの対応はこれらの入力の処理と統合に基づいています。
Thus, we perceive food flavor as a combination of olfactory and gustatory inputs.
したがって、私たちは食べ物の風味を嗅覚と味覚の組み合わせとして認識します。
Notably, the information provided through different channels is not equivalent: if something looks good and smells good, we will eat it, but if it looks good and smells bad, then we won’t. If it looks and smells good but tastes bad, we will not eat it either.
特に、異なるチャネルを通じて提供される情報は同等ではありません:見た目が良くて匂いが良ければ、私たちはそれを食べますが、見た目が良くて匂いが悪ければ食べません。見た目や匂いは良くても味が悪い場合も、私たちは食べません。
This is because some information channels are more reliable than others (here, taste is a better indicator of quality than appearance).
これは、一部の情報チャネルが他の情報チャネルよりも信頼できるためです (ここでは、外観よりも味の方が品質を示す優れた指標です)。
Additionally, information paucity in one channel can be compensated to some degree by the information in another channel: if food looks suspicious but we are told it is supposed to look that way, we might go for it.
さらに、あるチャネルの情報不足は、別のチャネルの情報によってある程度補うことができます:食べ物が疑わしいように見えても、そのような見た目のものだと言われれば、私たちはそれを食べるかもしれません。
The point is that in order to make a decision, we need a complete packet of information.
重要なのは、決定を下すためには完全な情報が必要であるということです。
Whether we do act based on incomplete information depends on the cost of a mistake—the higher the cost, the less adventurous we are with our decisions under uncertainty.
私たちが不完全な情報に基づいて行動するかどうかは、間違いのコストによって決まります。コストが高ければ高いほど、不確実性の下での意思決定において冒険心がなくなります。
We can frame the problem of requisite signals for immune response initiation in a similar manner—as a complete packet of information that can be composed of inputs coming from different sensory channels.
同様の方法で、免疫応答開始に必要な信号の問題を、さまざまな感覚チャネルからの入力で構成できる完全な情報のパケットとして組み立てることができます。
These inputs carry different types of information, and some are more reliable than others.
これらの入力にはさまざまな種類の情報が含まれており、一部のものは他のものよりも信頼性が高くなります。
What makes the packet of information complete depends on the type of response—some responses require more information than others.
情報パケットを完成させるものは応答のタイプによって異なります。一部の応答は他の応答よりも多くの情報を必要とします。
For example, most infections will induce a tumor necrosis factor (TNF) response, but the type of infection (e.g., viral versus fungal) needs to be specified to induce either type I interferon (IFN-I) or interleukin (IL)-17 responses, respectively. たとえば、ほとんどの感染症は腫瘍壊死因子 (TNF) 反応を誘発します。 ただし、I 型インターフェロン (IFN-I) またはインターロイキン (IL)-17 応答をそれぞれ誘導するには、感染の種類 (ウイルス対真菌など) を指定する必要があります。
Induction of wrong response results in a failure to clear the infection.
間違った反応を誘発すると、感染を除去できなくなります。
Loosely speaking, how much information is needed for an immune response depends on the ratio of the costs of false-positive and false-negative errors (the cost of making a response when it is not needed to the cost of not making a response when it is needed).
大まかに言うと、免疫反応にどの程度の情報が必要かは、偽陽性と偽陰性のエラーのコストの比率によって決まります。 (必要のないときに応答するコストと、必要なときに応答しないことのコスト)。
The question then is, what are the different types of information that are required to induce a given immune response, or alternatively, what kind of responses can be induced with a given amount of information available?
そこで問題は、特定の免疫反応を誘発するために必要なさまざまな種類の情報は何なのか、あるいは、入手可能な一定量の情報でどのような種類の反応を誘発できるのか、ということです。
This framing can lead to a more general perspective than the traditional division of antigen-specific “adaptive” and antigen-non-specific “innate” immune responses.
この枠組みは、抗原特異的な「適応」免疫応答と抗原非特異的な「自然」免疫応答の従来の分類よりも、より一般的な視点に導くことができます。
Although a detailed discussion of the necessary and sufficient signals will require more targeted studies and analyses, here we will use some familiar examples to suggest the concept of parsing the information perceived by the immune system into different semantic categories (Box 2).
必要かつ十分なシグナルについての詳細な議論には、より対象を絞った研究と分析が必要ですが、ここでは、いくつかの身近な例を使用して、免疫系によって認識される情報をさまざまな意味論的カテゴリーに解析する概念を提案します (ボックス 2)。
Similar to word meanings, these categories have a hierarchical structure (e.g., a microbe is a more general category than a pathogen).
単語の意味と同様に、これらのカテゴリは階層構造を持っています (たとえば、微生物は病原体よりも一般的なカテゴリです)。
The roles of some of these characteristics are well-defined, and we know how they are detected by either the innate or adaptive immune systems.
これらの特性の一部の役割は明確に定義されており、それらが自然免疫系または適応免疫系によってどのように検出されるかがわかっています。
Thus, the conserved structures of microbial origin are detected by pattern-recognition receptors (PRRs), some virulence factors are detected by inflammasomes, bacterial viability can be interpreted by recognition of their mRNA, and microbial quantity may be simply reported by the magnitude of inflammatory response they induce.
したがって、微生物起源の保存された構造はパターン認識受容体 (PRR) によって検出されます。 いくつかの病原性因子はインフラマソームによって検出されます。 細菌の生存能力は、mRNA の認識によって解釈できます。 そして、微生物の量は、それらが誘発する炎症反応の規模によって単純に報告される可能性があります。
The cellular location of a pathogen is relevant, as indicated by the distinct responses induced by extracellular (Th17), vacuolar (Th1), and cytosolic (cytotoxic T lymphocytes) pathogens.
細胞外 (Th17)、液胞 (Th1)、および細胞質 (細胞傷害性 T リンパ球) 病原体によって誘導される異なる応答によって示されるように、病原体の細胞内での位置は関連しています。
How the immune system distinguishes between these locations is incompletely understood, except that cytosolic viral and bacterial infections trigger the IFN-I response.
免疫系がこれらの場所をどのように区別するかは完全には理解されていません。ただし、細胞質のウイルスおよび細菌感染が IFN-I 応答を引き起こすことは理解されています。
Commensal and invasive bacteria can be distinguished, in part, by the apical expression of some Toll-like receptors (TLRs) on epithelial cells.
共生細菌と侵入細菌は、上皮細胞上のいくつかのToll様受容体(TLR)の頂端発現によって部分的に区別することができます。
We also know that the site of antigen exposure matters, as indicated by the phenomenon of oral tolerance, where the introduction of antigens via the digestive system results in long-term unresponsiveness to the ingested antigen.
また、経口寛容現象によって示されるように、抗原曝露の部位が重要であることもわかっています。 消化器系を介して抗原が導入されると、摂取した抗原に対して長期間無反応になります。
On the antigen recognition side, it is known that antigen structure can be a relevant factor for B cells: high-density repetitive epitopes, characteristic of microbial surface antigens, for example, can engage T-independent type II B cell responses (Figure 1).
抗原認識の面では、抗原の構造が B 細胞に関連する因子である可能性があることが知られています: たとえば、微生物表面抗原の特徴である高密度反復エピトープは、T 非依存性 II 型 B 細胞応答に関与する可能性があります (図 1)。
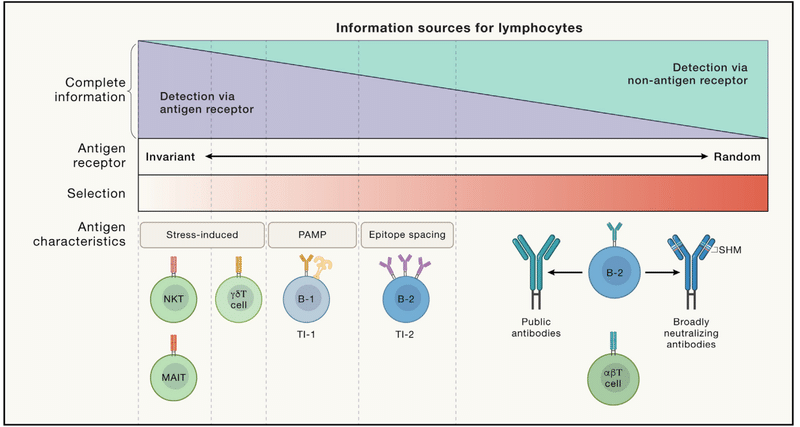
This is an example of how additional information (epitope arrangement) can compensate for pattern recognition and T cell help.
これは、追加情報 (エピトープ配置) がパターン認識と T 細胞の助けをどのように補うことができるかの例です。
Similarly, the chemical structure of antigens can be relevant, as indicated, for example, by the propensity of autoantibodies to target citrullinated antigens.
同様に、抗原の化学構造も関連する可能性があります。 これは、例えば、シトルリン化抗原を標的とする自己抗体の傾向によって示されます。
Whether and how this information is sensed and interpreted during the initiation of the response, however, is unclear.
しかし、この情報が対応の開始時に感知され解釈されるかどうか、またどのように解釈されるかは不明です。
Other characteristics listed above also appear relevant, but we don’t know whether and how they are detected and how their various combinations influence the immune responses.
上記に挙げた他の特徴も関連しているように見えますが、それらが検出されるかどうか、どのように検出されるか、またそれらのさまざまな組み合わせが免疫応答にどのように影響するかはわかっていません。
Information associated with some of these characteristics may well be the hidden variables that account for our lack of understanding of the rules of protective immunity against most infections, as well as autoimmunity and cancer immunity.
これらの特性の一部に関連する情報は、自己免疫やがん免疫だけでなく、ほとんどの感染症に対する防御免疫の規則に対する理解の欠如を説明する隠れた変数である可能性があります。
A systematic study of such input features in infections or vaccinations should reveal the patterns connecting them to the immune response outputs.
感染症やワクチン接種におけるそのような入力特徴の体系的な研究は、それらを免疫応答出力に結び付けるパターンを明らかにする必要があります。
As noted above, T and B lymphocytes with random antigen receptors cannot determine the origin of the antigen they are specific for—randomness allows for the receptors’ diversity but at the expense of information that can be interpreted by these receptors.
上で述べたように、ランダムな抗原受容体を持つ T リンパ球と B リンパ球は、それらが特異的な抗原の起源を決定することができません。 —ランダム性により受容体の多様性が許容されますが、受容体が解釈できる情報が犠牲になります。
The missing information is supplied by the innate immune system, and this is the reason for the dependence of adaptive immune response on innate immune recognition.
不足している情報は自然免疫系によって提供されます。 そしてこれが、適応免疫応答が自然免疫認識に依存する理由です。
However, the degree of randomness of antigen receptors varies from invariant receptors expressed by NKT and mucosal-associated invariant T (MAIT) cells to limited diversity of some γδT cells and B-1 B cells and marginal zone B cells to almost completely random receptors of most αβT cells and B-2 B cells.
ただし、抗原受容体のランダム性の程度は、NKT 細胞や粘膜関連不変 T (MAIT) 細胞によって発現される不変受容体から、一部の γδT 細胞や B-1 B 細胞の限られた多様性までさまざまです。 および辺縁帯B細胞は、ほとんどのαβT細胞およびB-2 B細胞のほぼ完全にランダムな受容体に反応します。
The less diverse and random the antigen receptors are, the less additional information is required by the lymphocytes that express them to determine the origin of the antigen they are specific for (Figure 1).
抗原受容体の多様性やランダム性が低いほど、それらを発現するリンパ球が特異的な抗原の起源を決定するために必要とする追加情報は少なくなります(図 1)。
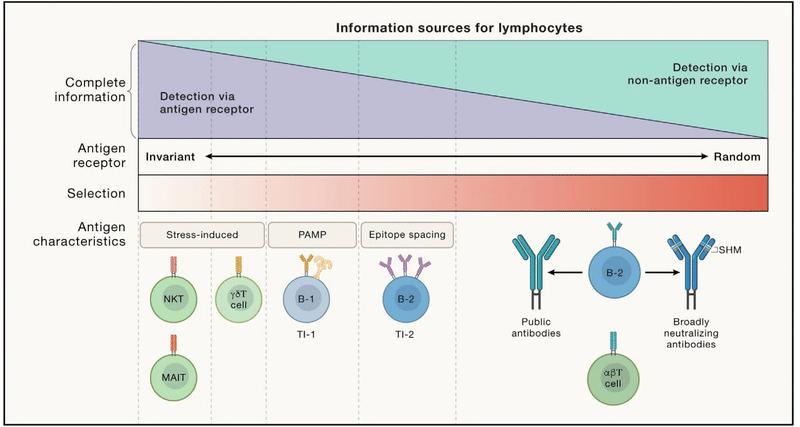
Thus, B-2 B cell activation requires T cell help, which selects specific B cell clones.
したがって、B-2 B 細胞の活性化には、特定の B 細胞クローンを選択する T 細胞の助けが必要です。
On the other hand, B-1 and marginal zone B cells can be activated in a polyclonal fashion, without T cell help, due to the reduced diversity of their antigen receptors.
一方、B-1 細胞と辺縁帯 B 細胞は、抗原受容体の多様性が低いため、T 細胞の助けなしでもポリクローナル様式で活性化できます。
Similarly, T cells with restricted T cell receptor (TCR) diversity require less information (compared with naive αβT cells with diverse TCR specificities) specifying their localization and response.
同様に、T 細胞受容体 (TCR) の多様性が制限された T 細胞では、その局在と応答を特定する情報が少なくて済みます (多様なTCR特異性を持つナイーブαβT細胞との比較)。
The reason is, again, that these cell populations are already preprogrammed to home to specific tissues and to respond in a certain way whenever their ligands are present.
その理由は、繰り返しになりますが、これらの細胞集団は、特定の組織にホーミングし、リガンドが存在する場合には常に特定の方法で反応するようにあらかじめプログラムされているためです。
So, the general principle is that the total amount of information required for the response remains more or less the same, but how it is partitioned between information that is genetically encoded versus the information that is received through other channels (e.g., instructions by the innate immune system and antigen structure) varies depending on the degree of antigen receptor diversity (Figure 1).
したがって、一般原則として、応答に必要な情報の総量はほぼ同じままです。 しかし、遺伝的にコード化された情報と他の経路(自然免疫系や抗原構造による指示など)を通じて受け取った情報との間で情報がどのように分割されるかは、抗原受容体の多様性の程度によって異なります(図1)。
An additional layer of information processing by lymphocytes with diverse antigen receptors is based on the principle related to the “crowd intelligence” implemented by quorum sensing.
多様な抗原受容体を持つリンパ球による情報処理の追加層は、クォーラム センシングによって実装される「群集インテリジェンス」に関連する原理に基づいています。
Quorum sensing can play two distinct roles: one is to ensure that there is a critical mass of cells necessary for a given function.
クォーラム センシングは 2 つの異なる役割を果たすことができます。1 つは、特定の機能に必要なセルの臨界量が確実に存在することです。
Another is to optimize decision-making: when individual cells have incomplete information, they can improve their decision-making accuracy by “pooling many imperfect estimates.”
もう 1 つは、意思決定を最適化することです。個々のセルが不完全な情報を持っている場合、「多くの不完全な推定値をプールする」ことで意思決定の精度を向上させることができます。
The principle is based on the production of a signal (autoinducer) by individual cells in amounts proportional to their degree of “confidence” in their estimate of the inputs they receive.
この原理は、個々の細胞が受け取る入力の推定における「信頼度」の程度に比例した量のシグナル(自己誘導物質)を生成することに基づいています。
The concentration of autoinducer then reflects the “collective wisdom” of the cell population, thereby reducing the error rate.
自己誘導物質の濃度は細胞集団の「集合知」を反映し、それによってエラー率が減少します。
The quorum-sensing phenomena have been described in the immune system, where secreted IL-2 plays the role of autoinducer in CD4+ T cells, while IL-2-consuming Tregs may play the role of an “arbiter” that can control the threshold required for activation of the response.
クオラムセンシング現象は免疫系で説明されており、分泌された IL-2 が CD4+ T 細胞の自己誘導物質の役割を果たします。 一方、IL-2を消費するTregは、応答の活性化に必要な閾値を制御できる「仲裁者」の役割を果たす可能性があります。
One should note that the downside of the strategy that leads to collective wisdom is that it can also create a “mob mentality” by increasing confidence in false information, as illustrated by the effect of “fake news” on public opinion.
集合知につながるこの戦略のマイナス面は、「フェイクニュース」が世論に及ぼす影響に見られるように、誤った情報への信頼を高めることで「群集心理」を生み出す可能性があることに注意すべきである。
In the context of the immune system, this may create a risk of autoimmune response if enough autoreactive T cells get randomly “agitated” based on incomplete information (e.g., in the absence of infection).
免疫系の文脈では、不完全な情報に基づいて十分な自己反応性 T 細胞がランダムに「興奮」した場合(感染がない場合など)、自己免疫反応のリスクが生じる可能性があります。
Such effects are normally prevented by Treg cells but may unfold in their absence.
このような影響は通常、Treg 細胞によって防止されますが、Treg 細胞が存在しない場合には発現する可能性があります。
In addition, just as a few strongly opinionated members of a population can have a disproportional impact on collective decision-making, a few highly reactive T cell “influencers” may sway “public opinion” by high production of IL-2.
さらに、集団内の少数の強い意見を持つメンバーが集団の意思決定に不均衡な影響を与える可能性があるのと同様に、少数の高反応性 T 細胞「影響力者」が IL-2 の高産生によって「世論」を揺さぶる可能性があります。
This could be beneficial if the response is desired and detrimental if it is not (e.g., if the response is directed to self-antigens).
これは、応答が望ましい場合には有益ですが、そうでない場合(たとえば、応答が自己抗原に向けられている場合)には有害である可能性があります。
The extreme cases of the latter scenario are presumably prevented by the negative selection of T cells with a particularly strong affinity to self-antigens.
後者のシナリオの極端なケースは、おそらく、自己抗原に対して特に強い親和性を持つ T 細胞のネガティブ選択によって防止されると考えられます。
Sensory systems are often designed to detect the change in the input rather than its absolute value.
感覚システムは多くの場合、入力の絶対値ではなく、入力の変化を検出するように設計されています。
A particularly prevalent feature of the sensory system is fold-change detection.
感覚系の特に一般的な機能は、倍数変化の検出です。
This and other forms of sensory adaptation are common in biology, and the immune system is no exception.
このような感覚適応と他の形態の感覚適応は生物学では一般的であり、免疫系も例外ではありません。
Indeed, change detection, or more generally, detection of specific patterns of change, has been proposed to be an essential feature of immune recognition.
実際、変化の検出、より一般的には特定の変化パターンの検出は、免疫認識の必須の特徴であると提案されています。
This mechanism can operate at the level of individual lymphocytes or cell populations, as it is orthogonal, rather than alternative, to quorum sensing and related mechanisms.
このメカニズムは個々のリンパ球または細胞集団のレベルで機能します。 これは、クォーラム センシングおよび関連メカニズムに代わるものではなく、直交しているためです。
The rate of change of input may be a particularly important feature that distinguishes “replicating” antigens, associated with bacteria and viruses, and non-replicating ones associated with allergens and parasitic worms (where the replication rate is effectively zero on the timescale of an immune response).
入力の変化率は、細菌やウイルスに関連する「複製」抗原を区別する特に重要な特徴である可能性があります。 もう1つは、アレルゲンや寄生虫に関連する非複製型(免疫応答の時間スケールでは複製率が事実上ゼロ)です。
Inactivated and subunit vaccines also provide non-replicating antigens, which may affect the quality of the response they elicit, as we discuss below.
不活化ワクチンとサブユニットワクチンは非複製抗原も提供するため、以下で説明するように、ワクチンが引き起こす反応の質に影響を与える可能性があります。
In summary, the canonical distinction between innate and adaptive immune recognition needs to be complemented with the full spectrum of the information relevant to the decision-making by the immune system.
要約すると、自然免疫認識と適応免疫認識の間の標準的な区別は、免疫系による意思決定に関連するあらゆる情報で補完される必要があります。
A lack of information within one semantic category can be compensated by synonymous signals from another category, as seen with T-independent type II B cell responses, where the detection of an epitope arrangement that is characteristic of microbial antigens compensates for the lack of pattern recognition by the innate immune system.
ある意味カテゴリ内の情報の欠如は、T 非依存性 II 型 B 細胞応答に見られるように、別のカテゴリからの同義のシグナルによって補うことができます。 ここでは、微生物抗原に特徴的なエピトープ配置の検出により、自然免疫系によるパターン認識の欠如が補われます。
In the absence of complete instructions, the immune system only makes stereotypical responses that match available information.
完全な指示がない場合、免疫系は入手可能な情報に一致する定型的な反応のみを行います。
The more information is available, the more qualified responses are generated.
利用可能な情報が多ければ多いほど、より適切な応答が生成されます。
In addition, quorum sensing and other mechanisms involving communications between lymphocytes may explain some phenomena not accounted for by the current paradigms.
さらに、クオラムセンシングやリンパ球間のコミュニケーションに関わるその他のメカニズムは、現在のパラダイムでは説明できないいくつかの現象を説明できる可能性があります。
Memory
Memory is one of the key characteristics of adaptive immunity.
記憶は適応免疫の重要な特性の 1 つです。
Memory can be defined as the long-term storage of information.
メモリは、情報の長期保存として定義できます。
From this basic perspective, three questions require clarification: what information is stored in immune memory? How is it stored and retrieved? How long does the “long-term” storage have to be to qualify as memory?
この基本的な観点から、次の 3 つの質問を明確にする必要があります:免疫記憶にはどのような情報が保存されているのでしょうか?どのように保存され、取得されるのでしょうか?「長期」ストレージがメモリとして認定されるには、どれくらいの期間が必要ですか?
Traditionally, immune memory has been considered from the perspective of antigen-specificity: clonal selection and expansion of antigen-selected lymphocytes, followed by differentiation into long-lived memory cells, is a hallmark of adaptive immunity.
伝統的に、免疫記憶は抗原特異性の観点から考慮されてきました: 抗原選択されたリンパ球のクローン選択と増殖、それに続く長命の記憶細胞への分化は、適応免疫の特徴です。
Through this process, the information about antigen identity is stored in the form of expanded numbers of antigen-specific memory cells.
このプロセスを通じて、抗原の同一性に関する情報は、拡張された数の抗原特異的記憶細胞の形で保存されます。
In addition, immune memory stores information about the type and route of infection.
さらに、免疫記憶には感染の種類と経路に関する情報が保存されます。
This information is stored in the form of an effector class-specific differentiation state of the memory lymphocytes and their location of residency.
この情報は、記憶リンパ球のエフェクタークラス特異的な分化状態およびその常駐位置の形で保存されます。
Beyond that, however, we do not know if any other information about a given infection might be stored in the immune memory.
しかし、それ以上に、特定の感染症に関する他の情報が免疫メモリに保存されているかどうかはわかりません。
As noted above, several types of information related to an infecting pathogen and its antigens are relevant for the induction of immune responses, raising the question of whether any of it is also stored in immune memory and, if so, how?
上で述べたように、感染する病原体とその抗原に関連するいくつかの種類の情報が免疫応答の誘導に関連します。 そのどれかが免疫記憶にも保存されているのか、もし保存されている場合はどのように保存されるのかという疑問が生じます。
Detecting information relevant to the initiation of the response and storing it in immune memory is a process that is formally analogous to associative learning—signals reporting on the microbial origin, type of pathogen, route of entry, etc., are learned by association with the antigen identity, as they control clonal selection and differentiation of lymphocytes, including memory cells.
反応の開始に関連する情報を検出し、免疫記憶に保存することは、形式的には連想学習に似たプロセスです。 -微生物の起源、病原体の種類、侵入経路などを報告するシグナルは、記憶細胞を含むリンパ球のクローン選択と分化を制御するために、抗原の同一性と関連付けることによって学習されます。
One might wonder whether the immune system also takes advantage of reinforcement learning, whereby the information about the consequence of the immune response is also evaluated and recorded.
免疫システムも強化学習を利用しており、それによって免疫応答の結果に関する情報も評価および記録されるのではないかと思う人もいるかもしれません。
If a response is successful in clearing the infection, are the parameters of the response (effector type, localization, magnitude, etc.) reinforced; and if the response is unsuccessful in some way, are the relevant parameters tuned down?
反応が感染の除去に成功した場合、反応のパラメーター (エフェクターの種類、局在化、大きさなど) は強化されますか;また、応答が何らかの形で失敗した場合、関連するパラメータは調整されていますか?
This is exactly what happens on the evolutionary timescale, but does it also happen at the level of individual organisms?
これはまさに進化の時間スケールで起こっていることですが、個々の生物のレベルでも起こるのでしょうか?
A trivial case of reinforcement learning is based on the clonal selection of appropriate lymphocytes (either from a naive pool or during affinity maturation): if the correct clones are selected and the response clears the pathogen, that very clone would give rise to memory cells.
強化学習の平凡なケースは、適切なリンパ球のクローン選択に基づいています (ナイーブ プールから、またはアフィニティ成熟中のいずれか): 正しいクローンが選択され、その反応によって病原体が除去されれば、まさにそのクローンが記憶細胞を生み出すことになります。
Less trivial examples may involve the calibration of the response efficiency based on the time it takes to clear an infection.
それほど平凡ではない例としては、感染を除去するのにかかる時間に基づいた応答効率の調整が含まれる場合があります。
Elegant studies suggestive of this principle showed that the duration of a pathogen or a specific antigen negatively correlates with the efficiency of memory generation; thus, acute infections generate better memory than chronic infections, perhaps because a pathogen’s persistence implies failure of the response.
この原理を示唆するエレガントな研究では、病原体または特定の抗原の持続時間が記憶生成の効率と負の相関があることが示されました; したがって、急性感染症は慢性感染症よりも優れた記憶を生成します。これは、おそらく病原体の持続性が反応の失敗を意味しているためと考えられます。
Mechanistically, chronic infections suppress memory formation via the effect of inflammatory cytokines that favor effector over memory T cell differentiation.
メカニズム的には、慢性感染症は、記憶 T 細胞の分化よりもエフェクターに有利な炎症性サイトカインの影響を介して記憶形成を抑制します。
The success of a response is a function of two variables—the efficiency of pathogen clearance and the fitness cost of the response.
応答の成功は、病原体除去の効率と応答の適応コストという 2 つの変数の関数です。
If the pathogen is cleared at the cost of extensive immunopathology, the parameters of the response may not be reinforced.
広範な免疫病理を犠牲にして病原体を除去した場合、反応のパラメーターは強化されない可能性があります。
Whether and how the fitness costs of the immune response are recorded is unknown, but if the magnitude of inflammatory response is used as a proxy for fitness cost, then a mechanism of translating fitness cost to memory generation would be the same as the one used to evaluate a pathogen’s persistence.
免疫反応の適応コストが記録されるかどうか、またどのように記録されるかは不明です。 しかし、炎症反応の大きさが適応度コストの代用として使用される場合、適応度コストを記憶生成に変換するメカニズムは、病原体の持続性を評価するために使用されるメカニズムと同じになるでしょう。
Beyond the canonical memory mechanisms that are based on clonal selection, accumulating evidence points to additional types of immune memory, including stable changes in gene expression enabled by chromatin remodeling demonstrated in both macrophages and epithelial stem cells, selective expansion of NK cells expressing particular combinations of activating receptors, and tissue-specific retention of NK cells after primary infection.
クローン選択に基づく標準的な記憶メカニズムを超えて、追加の種類の免疫記憶が示されている証拠が蓄積されています。これには、マクロファージと上皮幹細胞の両方で実証されたクロマチンリモデリングによって可能になる遺伝子発現の安定した変化、活性化受容体の特定の組み合わせを発現するNK細胞の選択的増殖、および一次感染後のNK細胞の組織特異的保持が含まれます。
These mechanisms operate on different timescales, but they can all contribute to various aspects of immune memory.
これらのメカニズムは異なるタイムスケールで動作しますが、それらはすべて免疫記憶のさまざまな側面に寄与する可能性があります。
It is conceivable that these additional memory mechanisms may retain the information complementary to the one stored in memory lymphocytes.
これらの追加の記憶機構は、記憶リンパ球に保存されている情報と相補的な情報を保持している可能性があると考えられます。
Immunological memory may also be recorded and stored in the central nervous system: the neuronal circuits in the insular cortex were demonstrated to store and retrieve information about organ-specific inflammatory responses.
免疫学的記憶は、中枢神経系にも記録され、保存される場合があります: 島皮質の神経回路は、臓器固有の炎症反応に関する情報を保存および検索することが実証されました。
This example suggests a much broader potential for the involvement of the nervous system in detecting, interpreting, and storing the information associated with infections.
この例は、感染症に関連する情報の検出、解釈、保存に神経系が関与する可能性がはるかに広いことを示唆しています。
How this information is transmitted back to the immune system through the descending motor pathways is an area of enormous interest for future studies.
この情報が下行性運動経路を介して免疫系にどのように伝達されるかは、今後の研究にとって非常に興味深い分野です。
Ultimately, learning and memory by the immune system, whether through evolution or individual life history, underlie the currently elusive principles of protective immunity, which we discuss next.
結局のところ、免疫系による学習と記憶は、進化を通じてであれ、個人の生活史を通じてであれ、現在はとらえどころのない防御免疫の原理の基礎となっており、これについては次に説明します。
Host-pathogen interactions: Some ground rules
宿主と病原体の相互作用: いくつかの基本原則
What ultimately matters to pathogens is to be able to successfully establish colonization within the host, replicate, and transmit to the next host.
病原体にとって最終的に重要なのは、宿主内でうまく定着し、複製し、次の宿主に伝達できるかどうかです。
These are the pathogens’ life history traits—the traits that are directly acted on by natural selection.
これらは病原体の生活史特性、つまり自然選択によって直接作用する特性です。
Each of these goals can be achieved in a variety of ways, and their specific combinations define the pathogen’s life history strategy.
これらの目標はそれぞれさまざまな方法で達成でき、その特定の組み合わせによって病原体の生活史戦略が決まります。
For example, some pathogens maximize the replication and transmission rate, have a short residence time in the host, and therefore may not evolve elaborate adaptations to the host’s niches.
たとえば、一部の病原体は複製と伝達速度を最大化し、宿主内での滞留時間が短いため、宿主のニッチに精巧な適応を進化させない可能性があります。
Examples include seasonal respiratory viruses, such as influenza viruses and coronaviruses.
例には、インフルエンザウイルスやコロナウイルスなどの季節性呼吸器ウイルスが含まれます。
They typically cause acute infections that either result in pathogen clearance or host death.
これらは通常、病原体の排除または宿主の死をもたらす急性感染症を引き起こします。
Other pathogens may optimize adaptation to the host, which is often associated with a slow replication rate and transmission mechanisms that do not pose a severe burden on the host’s fitness.
他の病原体は宿主への適応を最適化する可能性があります。 これは、多くの場合、遅い複製速度と、宿主の適応度に深刻な負担を与えない伝達メカニズムに関連しています。
These pathogens, including hepatitis B and C viruses, Mycobacterium tuberculosis, and Helicobacter pylori, cause chronic infections.
B 型および C 型肝炎ウイルス、結核菌、ヘリコバクター ピロリなどのこれらの病原体は、慢性感染症を引き起こします。
The extreme version of this strategy is the latent infections with a prolonged halt on replication and transmission, exemplified by large double-stranded DNA (dsDNA) viruses of the Herpesviridae, including herpes simplex virus, varicella-zoster virus, and Epstein-Barr virus.
この戦略の極端なバージョンは、単純ヘルペス ウイルス、水痘帯状疱疹ウイルス、エプスタイン バー ウイルスなどのヘルペスウイルス科の大型二本鎖 DNA (dsDNA) ウイルスに代表される、複製と伝達の長期停止を伴う潜伏感染です。
In addition, from the pathogens’ perspective, the host consists of three compartments that may or may not overlap: these are the sites for entry, replication, and transmission (some pathogens may have an additional compartment for long-term residence).
さらに、病原体の観点から見ると、宿主は 3 つのコンパートメントで構成されており、これらは重なっている場合もあればそうでない場合もあります。 これらは、侵入、複製、および伝播の場所です(一部の病原体は、長期滞留するための追加の区画を持っている場合があります)。
This is because the site of pathogen entry may not be the best site for replication, residence, or transmission.
これは、病原体の侵入部位が複製、居住、または伝播に最適な部位ではない可能性があるためです。
When this is the case, the pathogens evolve an additional step in their infection cycle—the dissemination within the host to allow the transition between these compartments.
この場合、病原体は感染サイクルの追加のステップ、つまり宿主内での播種を進化させて、これらの区画間の移行を可能にします。
Depending on the presence or absence of internal barriers between compartments and tissue tropism of the pathogen, transitions between these sites can either occur passively (for example, when virions are carried by circulation), or they may require elaborate adaptations, as is common for complex parasites.
コンパートメント間の内部障壁の有無と病原体の組織指向性に応じて、これらの部位間の移行は受動的に起こる可能性があります (例えば、ウイルス粒子が循環によって運ばれる場合)。 あるいは、複雑な寄生虫によく見られるように、精巧な適応が必要な場合もあります。
Finally, additional variations and elaborations on these themes take place in pathogens that have intermediate hosts, carried by different types of vectors, or manipulate the host’s physiology or behavior to optimize the infection cycle.
最後に、これらのテーマに関する追加のバリエーションと精緻化は、さまざまな種類のベクターによって運ばれる中間宿主を持つ病原体、または感染サイクルを最適化するために宿主の生理機能や行動を操作する病原体で行われます。
The impact of an infection on the host fitness is typically a byproduct of pathogens’ replication, adaptation, and transmission strategies and can vary from life-threatening, to mild, to beneficial.
宿主の体力に対する感染の影響は通常、病原体の複製、適応、伝播戦略の副産物であり、生命を脅かすものから軽度のもの、有益なものまでさまざまです。
In the latter case, the terms “infection” and “pathogen” are replaced with “colonization” and “commensal,” but all these scenarios represent variations on the same theme—optimization of reproductive success through specific combinations of different “entry, replication, adaptation, and exit” strategies.
後者の場合、「感染」と「病原体」という用語は「定着」と「共生」に置き換えられます。 しかし、これらのシナリオはすべて、異なる「入口、複製、適応、出口」戦略の特定の組み合わせによる生殖成功の最適化という同じテーマのバリエーションを表しています。
Conversely, what ultimately matters to the host is surviving the infection and minimizing its negative effects on fitness.
逆に、宿主にとって最終的に重要なのは、感染から生き残ることと、フィットネスへの悪影響を最小限に抑えることです。
In principle, this can be achieved by combinations of different defense strategies that broadly fall into two categories: resistance and tolerance.
原則として、これは、抵抗と寛容の 2 つのカテゴリに大別されるさまざまな防御戦略を組み合わせることによって達成できます。
In this context, tolerance refers to the host’s ability to adapt and survive infection without targeting the pathogen, while resistance aims to eliminate the pathogen through immune defenses.
この文脈において、寛容とは、病原体を標的にすることなく感染に適応して生き残る宿主の能力を指します。 一方、抵抗は免疫防御によって病原体を排除することを目的としています。
An important point of intersection of these strategies has to do with minimizing the costs of resistance—the collateral damage and other fitness costs of immune defenses.
これらの戦略が交わる重要な点は、抵抗力のコスト、つまり付随的損害や免疫防御のその他の適応コストを最小限に抑えることと関係しています。
These costs can be substantial enough to be the major drivers of morbidity and mortality and, as such, have to be taken into account when considering the overall quality of the immune defense.
これらのコストは、罹患率と死亡率の主な要因となる可能性があります。 したがって、免疫防御の全体的な質を考慮する際には考慮する必要があります。
Because survival with minimal fitness costs is the ultimate “readout” of natural selection acting on the host population, it follows that defense efficiency has to be evaluated with these outcomes in mind.
最小の適応度コストでの生存は、宿主集団に作用する自然選択の究極の「読み出し」であるため、防御効率はこれらの結果を念頭に置いて評価する必要があるということになります。
This brings up the notion of “protective immunity”—perhaps the least understood but most important aspect of immune defenses.
ここで「防御免疫」という概念が浮上します。 —おそらく最も理解されていないが、免疫防御の最も重要な側面です。
Protective immunity
防御免疫
Unlike other characteristics of immune responses, such as magnitude, effector class, kinetics, etc., protective immunity is defined by the outcome of the response—whether it succeeded in protecting the host from illness and death that would otherwise be caused by an infection.
免疫応答の他の特性(大きさ、エフェクタークラス、動態など)とは異なり、防御免疫は応答の結果によって定義されます。 —感染によって引き起こされるであろう病気や死から宿主を守ることに成功したかどうか。
As such, protective immunity refers to the ultimate performance of the defenses, taking into account their effectiveness, coordination, costs, and outcomes.
したがって、防御免疫とは、防御の有効性、調整、コスト、結果を考慮した防御の最終的なパフォーマンスを指します。
The desired outcome (as defined above) may or may not involve pathogen clearance—destroying some pathogens may be more costly than tolerating their presence.
望ましい結果 (上記で定義したとおり) には、病原体の除去が含まれる場合と含まれない場合があります。一部の病原体を破壊することは、その存在を許容するよりもコストがかかる場合があります。
Here, for simplicity, we will focus on the common scenarios of acute infections where pathogen clearance is an essential outcome of protective immunity.
ここでは、話を簡単にするために、病原体の除去が防御免疫の重要な結果である急性感染症の一般的なシナリオに焦点を当てます。
In principle, immune defenses can target every step of a pathogen’s infection cycle: entry, replication, and transition between different compartments.
原則として、免疫防御は病原体の感染サイクルのあらゆる段階(侵入、複製、異なる区画間の移行)を標的にすることができます。
The transmission step would not be expected to be targeted explicitly, as that would not benefit the infected host, but it can happen as a byproduct of targeting replication.
感染したホストに利益をもたらさないため、送信ステップは明示的にターゲットにされることは期待されません。 ただし、複製段階を標的にした場合の副産物として発生する可能性があります。
Each of the stages of a pathogen’s life cycle can negatively impact the host to different degrees, depending on the pathogen.
病原体のライフサイクルの各段階は、病原体に応じてさまざまな程度で宿主に悪影響を及ぼす可能性があります。
Conversely, the fitness costs of the defense (the degree of immunopathology) can vary depending on the stage targeted by the defenses.
逆に、防御 (免疫病理の段階) の適応コストは、防御の対象となる段階に応じて変化する可能性があります。
Thus, blocking the pathogen’s entry at barrier tissues would be expected to be less damaging to the host than killing it in the liver or the brain.
したがって、障壁組織で病原体の侵入をブロックすると、宿主へのダメージが 肝臓や脳で殺すよりも少なくなることが期待されます。
To account for the differential sensitivity of tissues to collateral damage, the defenses with high fitness costs are generally not deployed in the vulnerable tissues where the cost of these defenses would be too high.
付随的損傷に対する組織の感受性の違いを考慮して、適応コストの高い防御は、通常、防御のコストが高すぎる脆弱な組織には導入されません。
The extreme version of this principle is the so-called immunoprivileged sites, where highly destructive immune responses seem to be prohibited.
この原則の極端なバージョンは、いわゆる免疫特権部位であり、高度に破壊的な免疫反応が禁止されているように見えます。
It thus appears that in general, the parameters of protective immunity can be defined by the choices of appropriate defense modules deployed to target pathogen’s entry, replication, or dissemination, such that the outcome would be most effective in terms of both pathogen control and minimal immunopathology.
したがって、一般に、防御免疫のパラメーターは、標的となる病原体の侵入、複製、または蔓延に配備される適切な防御モジュールの選択によって定義できるようです。 その結果、病原体制御と最小限の免疫病理学の両方の観点から最も効果的な結果が得られます。
With this general perspective in mind, we will now discuss possible reasons for the successes and failures of different vaccines to elicit protective immunity.
この一般的な観点を念頭に置いて、さまざまなワクチンが防御免疫を誘発する成功と失敗の考えられる理由について説明します。
Vaccines and protective immunity
ワクチンと防御免疫
A pivotal achievement in immunology is the creation of vaccines against a variety of infectious agents.
免疫学における極めて重要な成果は、さまざまな感染因子に対するワクチンの作成です。
The advent of new technologies that enable precise measurements of cellular and molecular features of the immune system has led to an unprecedented level of characterization of immune cell subsets and effector functions.
免疫系の細胞および分子の特徴の正確な測定を可能にする新技術の出現により、免疫細胞サブセットおよびエフェクター機能の前例のないレベルの特性評価が可能になりました。
Yet, as discussed above, we still do not understand the rules of protective immunity against a given pathogen or how we can reliably establish it through vaccination.
しかし、上で議論したように、特定の病原体に対する防御免疫の規則や、ワクチン接種によってそれを確実に確立する方法はまだ理解されていません。
Much of the remarkable success of vaccines is the result of trial and error before our understanding of the immune system.
ワクチンの目覚ましい成功の多くは、免疫システムを理解する前の試行錯誤の結果です。
The smallpox vaccine was developed in the late 1700s based on the inoculation of closely related cowpox virus.
天然痘ワクチンは、近縁の牛痘ウイルスの接種に基づいて 1700 年代後半に開発されました。
The widely used and highly effective childhood vaccines against measles, mumps, and rubella (MMR) rely on live-attenuation techniques developed before the understanding of innate and adaptive immunity.
麻疹、おたふく風邪、風疹(MMR)に対する広く使用されており非常に効果的な小児ワクチンは、自然免疫と適応免疫が理解される前に開発された生弱毒化技術に依存しています。
It turns out that much of the success of these historical vaccines stems from the combination of the fact that (1) live vaccines and live-attenuated vaccines elicit robust and long-lasting antibody responses, (2) protection relies on circulating IgG, and (3) these pathogens do not undergo rapid mutations or rapid transmission and mostly consist of a single serotype.
これらの歴史的なワクチンの成功の多くは、以下の事実の組み合わせによるものであることが判明しています。①生ワクチンと弱毒化生ワクチンは強力で持続的な抗体反応を誘発、② 保護は循環 IgG に依存、③これらの病原体は急速な突然変異や急速な伝播を起こさず、ほとんどが単一の血清型で構成されている。
For example, measles, mumps, rubella, and smallpox first replicate in the respiratory tract with slow replication kinetics, and the disease is caused by viremia followed by seeding of various organs.
たとえば、麻疹、おたふく風邪、風疹、天然痘は、まず気道内でゆっくりとした複製速度で増殖し、ウイルス血症とその後のさまざまな器官への播種によって病気が引き起こされます。
This enables circulating antibodies elicited by vaccines and the anamnestic responses elicited by memory lymphocytes to intersect with the virus before they cause disease (Figure 2).
これにより、ワクチンによって誘発された循環抗体と記憶リンパ球によって誘発された既往反応が、病気を引き起こす前にウイルスと交差することが可能になります(図2)。
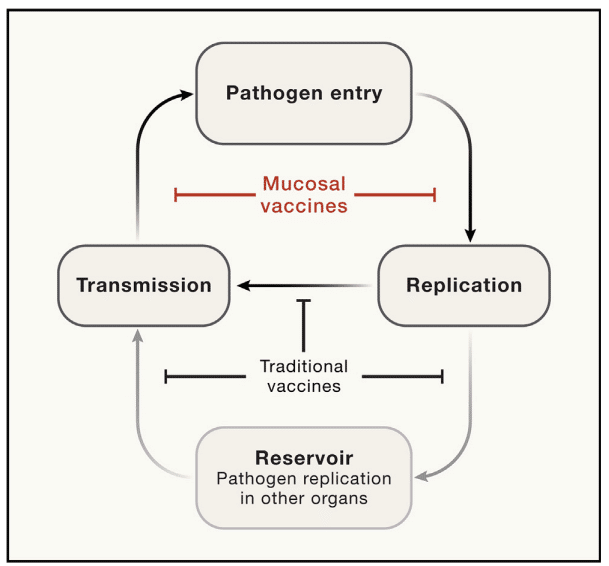
By contrast, the COVID-19 pandemic highlighted our gap in understanding how to develop vaccines against rapidly mutating respiratory pathogens that have a short incubation period.
対照的に、COVID-19パンデミックは、潜伏期間が短く急速に変異する呼吸器病原体に対するワクチンを開発する方法についての理解のギャップを浮き彫りにしました。
These rapidly replicating and transmitting respiratory viruses include the pandemic-causing and seasonal coronaviruses, influenza viruses, and respiratory syncytial virus (RSV).
これらの急速に複製および伝染する呼吸器ウイルスには、パンデミックとなるコロナウイルスや季節性コロナウイルス、インフルエンザ ウイルス、呼吸器合胞体ウイルス (RSV) が含まれます。
Natural infections with this class of respiratory viruses do not prevent reinfection, either due to the emergence of immune escape mutants or short-lived resistance.
このクラスの呼吸器ウイルスによる自然感染は、免疫逃避変異体の出現または短命の耐性のいずれかにより、再感染を防ぐことはできません。
Thus, vaccines have to do better than natural infection if they are to confer protective immunity.
したがって、ワクチンが防御免疫を与えるためには、自然感染よりも優れた効果を発揮する必要があります。
The fundamental problem rests in our inability to pinpoint what constitutes protective immunity against this class of viruses.
根本的な問題は、このクラスのウイルスに対する防御免疫を構成するものを正確に特定できないことにあります。
Based on our experience with SARS-CoV-2, even with the potent mRNA vaccines, vaccine-induced antibodies may not be sustained at high enough levels to provide protection for more than a few months.
SARS-CoV-2 に関する私たちの経験に基づくと、たとえ強力な mRNA ワクチンを使用したとしても、ワクチンによって誘導される抗体は数か月以上防御できるほど十分なレベルに維持されない可能性があります。
Further, the delay between reinfection and the emergence of an anamnestic response might be too long to control the replication of these respiratory viruses.
さらに、再感染してから既往反応が現れるまでの遅延が長すぎて、これらの呼吸器ウイルスの複製を制御できない可能性があります。
However, if a vaccine were to develop local plasma cells that produce long-lasting IgG or secretory IgA (S-IgA) secreted into the nasal and respiratory mucosal surfaces at high enough levels or tissue-resident antibody-secreting cells to quickly elevate these antibodies in response to the incoming virus, protective immunity against these rapidly replicating and transmitting viruses may be possible.
しかし、 ワクチンが、侵入ウイルスに反応してこれらの抗体を迅速に上昇させるのに十分に高いレベルで鼻および呼吸器粘膜表面に分泌される長期持続型 IgG または分泌型 IgA (S-IgA) を産生する局所形質細胞、または組織常駐抗体分泌細胞を生成する場合、これらの急速に複製および伝播するウイルスに対する防御免疫が可能である可能性があります。
Promptly inducible anamnestic secretory-IgA response is key to preventing onward transmission of SARS-CoV-2.
速やかに誘発される既往歴のある分泌型 IgA 応答は、SARS-CoV-2 のさらなる伝播を防ぐ鍵となります。
Such desirable immune responses can be induced by a combination of mRNA vaccine priming followed by nasal administration of recombinant spike protein in the absence of adjuvants or adenoviral-vectored spike vaccine.
このような望ましい免疫応答は、mRNAワクチンプライミングとそれに続くアジュバントまたはアデノウイルスベクター化スパイクワクチンの非存在下での組換えスパイクタンパク質の経鼻投与の組み合わせによって誘導することができます。
Recent studies demonstrated that nasal or inhaled vaccine and booster strategies can indeed achieve near-sterilizing immunity against SARS-CoV-2 challenge in non-human primates.
最近の研究では、経鼻または吸入ワクチンおよび追加免疫戦略が、ヒト以外の霊長類において実際にSARS-CoV-2攻撃に対してほぼ滅菌免疫を達成できることが実証されました。
Based on these promising mucosal vaccine strategies, correlates of protection can be established in the future.
これらの有望な粘膜ワクチン戦略に基づいて、将来的には防御の相関関係を確立できる可能性があります。
A better definition of correlates of protection coupled with a potent mucosal vaccine strategy has the promise of achieving population-based immunity.
防御の相関関係をより正確に定義し、強力な粘膜ワクチン戦略と組み合わせることで、集団ベースの免疫を達成できる可能性があります。
As noted above, mechanisms that block transmission to protect a population would not be evolutionarily stable, unless they also protect the infected individual.
上で述べたように、集団を保護するために感染を阻止するメカニズムは、感染した個人も保護しない限り、進化的に安定していません。
However, public health measures can bring about population-based immunity through vaccines that interfere with the transmission phase.
しかし、公衆衛生対策は、伝染段階を妨げるワクチンを通じて集団ベースの免疫をもたらすことができます。
Another gap in our understanding of protective immunity is the mechanism for generating long-lived plasma cells (LLPCs) that can provide sufficient levels of circulating antibodies against pathogens.
防御免疫に関する私たちの理解におけるもう 1 つのギャップは、病原体に対する十分なレベルの循環抗体を提供できる長命形質細胞 (LLPC) を生成するメカニズムです。
LLPCs have been demonstrated for many live-attenuated vaccines and even for some respiratory infections such as the 1918 influenza virus.
LLPC は、多くの弱毒化生ワクチン、さらには 1918 年インフルエンザ ウイルスなどの一部の呼吸器感染症でも実証されています。
However, while natural SARS-CoV-2 infections and mRNA vaccines have been shown to induce plasma cells in the bone marrow, whether these cells are established in sufficient numbers and whether they contribute to the secretion of protective neutralizing antibodies for many years remains unclear.
ただし、自然の SARS-CoV-2 感染症と mRNA ワクチンは骨髄で形質細胞を誘導することが示されていますが、 これらの細胞が十分な数で確立されているかどうか、またそれらが長年にわたって防御中和抗体の分泌に寄与しているかどうかは不明のままです。
The mechanism by which some infections and vaccines elicit LLPCs while others do not is also unclear.
一部の感染症やワクチンが LLPC を誘発するメカニズムと、そうでないものがあるメカニズムも不明です。
The antigen load, duration of antigen presence, route of administration, dendritic cells, pathogen-associated molecular patterns (PAMPs), extent of inflammation, germinal center (GC) engagement, T cell help, and other factors are likely to contribute to the establishment of LLPCs.
抗原量、抗原存在期間、投与経路、樹状細胞、病原体関連分子パターン(PAMP)、炎症の程度、胚中心(GC)の関与、T細胞の助け、または他の要因が LLPC の設立に寄与する可能性があります。
Slow release of escalating doses of antigens induces long-term GC responses, resulting in enhanced antibody responses.
漸増用量の抗原をゆっくりと放出すると、長期的な GC 応答が誘導され、その結果、抗体応答が強化されます。
Solving the puzzle of inducing better LLPC responses is a critical challenge and warrants significant efforts for future vaccine development.
より良いLLPC反応を誘導するというパズルを解くことは重要な課題であり、将来のワクチン開発には多大な努力が必要です。
The discussion so far has focused on the canonical function of the immune system in host defense from infections.
これまでの議論は、感染からの宿主防御における免疫系の標準的な機能に焦点を当ててきました。
We now turn to possible non-canonical functions that take advantage of the same core operating procedures but applied to “domestic” issues.
ここで、同じコア操作手順を利用するものの、「ドメスティック」問題に適用される可能性のある非正規な機能に目を向けます。
Immune system: What is it good for?
免疫システム: それは何に良いのですか?
Biological traits that evolved to perform one function may later evolve to acquire additional functions, which may even become more prominent than the original ones.
1 つの機能を実行するために進化した生物学的形質は、後に追加の機能を獲得するために進化する可能性があります。 元のものよりも卓越している可能性さえあります。
This phenomenon is termed exaptation, and a classical example of it is the evolutionary history of feathers that evolved initially for thermoregulation and only later acquired the characteristics making them usable for flight.
この現象は外適応と呼ばれ、その古典的な例は、最初は体温調節のために進化し、後に初めて飛行に使用できる特性を獲得した羽毛の進化の歴史です。
Exaptations are common in the case of complex, multifunctional systems.
複雑な多機能システムの場合、外適応が一般的です。
Determining which functions were original and which are due to exaptations is not always possible, but the analysis of the characteristics of the system that enable exaptations can be illuminating.
どの機能がオリジナルで、どの機能が拡張によるものであるかを判断することは常に可能であるとは限りませんが、拡張を可能にするシステムの特性を分析することで明らかになる可能性があります。
This requires that the functions we are interested in are defined from first principles, in the most general way, rather than through specific, even if most prominent, examples.
これには、関心のある機能が、たとえ最も顕著な例であっても、特定の例を通じてではなく、最も一般的な方法で、第一原理に基づいて定義されることが必要です。
Here, we will briefly discuss how some of the basic functions of the immune system’s components can be viewed in a more general way to highlight their utilities beyond host defense.
ここでは、免疫系の構成要素の基本的な機能の一部を、宿主防御を超えたその有用性を強調するために、より一般的な方法でどのように見ることができるかについて簡単に説明します。
Although defense from infections is a critical and well-established function of the immune system, it is but one of the manifestations of its functional repertoire.
感染症からの防御は免疫系の重要かつ十分に確立された機能ですが、それは免疫系の機能レパートリーの発現の 1 つにすぎません。
Indeed, different components of the immune system can and do perform additional functions that are unrelated to anti-microbial defenses.
実際、免疫系のさまざまな構成要素は、抗菌防御とは関係のない追加の機能を実行する可能性があり、実際に実行しています。
This has been best appreciated for macrophages, which perform a plethora of functions in tissue homeostasis and maintenance, including the removal of apoptotic cells, remodeling of the extracellular matrix, and provision of growth factors for other cell types within tissues.
これはマクロファージにとって最もよく評価されています。マクロファージは、アポトーシス細胞の除去、細胞外マトリックスの再構築、組織内の他の細胞型への成長因子の提供など、組織の恒常性と維持において多くの機能を果たします。
These homeostatic functions are, in some sense, as important as the functions of macrophages in host defense from infections.
これらの恒常性維持機能は、ある意味、感染からの宿主防御におけるマクロファージの機能と同じくらい重要です。
The core functions of macrophages fall into four main categories: sensing of tissue micro-environment (oxygen, osmolarity, pH, extracellular matrix (ECM), presence of pathogens, etc.); phagocytosis and lysosomal degradation; communication through paracrine signals; and secretion of enzymes for extracellular degradation, including for ECM remodeling.
マクロファージの中核となる機能は、次の 4 つの主要なカテゴリに分類されます:組織微小環境のセンシング (酸素、浸透圧、pH、細胞外マトリックス (ECM)、病原体の存在など);食作用とリソソーム分解; 傍分泌信号を介したコミュニケーション;ECMリモデリングを含む細胞外分解のための酵素の分泌。
Through these core functions, macrophages promote host defense, tissue repair, remodeling, and development.
これらの中核機能を通じて、マクロファージは宿主防御、組織修復、リモデリング、および発達を促進します。
Macrophages are multifunctional jacks of all trades.
マクロファージは多機能な何でも屋です。
Their siblings (dendritic cells) and cousins (granulocytes) are specialized in performing subsets of these functions: dendritic cells and mast cells focus on sensing and communication, while neutrophils specialize in phagocytosis, lysosomal, and extracellular degradation.
その兄弟 (樹状細胞) といとこ (顆粒球) は、次の機能のサブセットを実行することに特化しています:樹状細胞とマスト細胞はセンシングとコミュニケーションに焦点を当てますが、好中球は食作用、リソソーム、および細胞外分解に特化します。
Although the focus has been on studying these functions in the context of infections, many, and perhaps all, of them also play important roles in tissue biology.
感染症に関連してこれらの機能を研究することに焦点が当てられてきましたが、それらの多く、そしておそらくすべてが組織生物学においても重要な役割を果たしています。
To expand our perspective, we need to replace specific terms, like “pathogen” and “infection,” with more general terms, like “unwanted cargo” and “altered tissue state.”
視野を広げるには、「病原体」や「感染症」などの特定の用語を、「不要な貨物」や「組織の状態の変化」などのより一般的な用語に置き換える必要があります。
Thus, opsonins, including complement and antibodies, “tag” unwanted materials (regardless of the reason why they are unwanted), including microbes, dead or damaged cells, or synapses, for removal by phagocytosis.
したがって、補体や抗体を含むオプソニンは、微生物、死んだ細胞や損傷した細胞、またはシナプスなどの不要な物質(不要な理由に関係なく)を食作用による除去のために「タグ付け」します。
Similarly, dendritic cells, in addition to sensing pathogens within tissues, may well sense other aspects of altered tissue homeostasis whether they are caused by infection or not.
同様に、樹状細胞は、組織内の病原体を感知することに加えて、感染によって引き起こされるかどうかに関係なく、組織恒常性の変化の他の側面を感知する可能性があります。
Upon detecting relevant alterations in tissue states, dendritic cells would convey this information to lymphocytes (innate lymphoid cells [ILCs] and T cells).
組織状態の関連する変化を検出すると、樹状細胞はこの情報をリンパ球 (自然リンパ球 [ILC] および T 細胞) に伝えます。
What ILCs and T cells do with this information is again mostly studied in the context of infections, but the same principles apply to other functions as well.
ILC と T 細胞がこの情報を使って行うことは、ここでも主に感染症に関連して研究されていますが、同じ原理が他の機能にも当てはまります。
To see this, we just need to consider T cell functions from a more general perspective.
これを理解するには、より一般的な観点から T 細胞の機能を考慮する必要があります。
For example, cytotoxic lymphocytes induce apoptosis in unwanted cells.
たとえば、細胞傷害性リンパ球は、不要な細胞にアポトーシスを誘導します。
Again, cells can be unwanted for a variety of reasons: they may be infected, damaged, found in the wrong place, or simply produced in excess.
繰り返しますが、細胞はさまざまな理由で不要になる可能性があります。感染、損傷、間違った場所で見つかった、または単に過剰に生成された可能性があります。
These conditions can also engage cell-intrinsic death programs (apoptosis, necroptosis, etc.).
これらの状態は、細胞固有の死プログラム (アポトーシス、ネクロトーシスなど) を引き起こす可能性もあります。
Cytotoxic lymphocytes complement these programs when conditions for cell-intrinsic cell death are either not met or cannot be properly executed.
細胞傷害性リンパ球は、細胞固有の細胞死の条件が満たされない場合、または適切に実行できない場合に、これらのプログラムを補完します。
A key question then is what makes cells “unwanted” from the cytotoxic lymphocytes’ perspective, and how do they sense this status of the prospective targets for elimination?
したがって、重要な問題は、細胞傷害性リンパ球の観点から見て、何が細胞を「不要」にするのか、そして細胞傷害性リンパ球は排除の対象となる可能性のあるこの状態をどのように感知するのかということです。
Do some cancer cells meet these criteria and others do not?
一部の癌細胞はこれらの基準を満たし、他の癌細胞は満たさないのでしょうか?
From our perspective, any cancer cell is unwanted, but only some types of cancer cells seem to fulfill the criteria for elimination by cytotoxic lymphocytes.
私たちの観点からは、どのようながん細胞も不要なものですが、細胞傷害性リンパ球による排除の基準を満たすのは一部の種類のがん細胞のみであるようです。
Currently, we do not fully understand what these criteria are, and consequently, we do not understand why some cancers are targeted by the immune system, while others are not.
現時点では、これらの基準が何であるかを完全に理解していないため、なぜ一部のがんが免疫系の標的となり、他のがんが標的にならないのかも理解できません。
Thinking from this general perspective, a core function of lymphocytes can be defined as the local provision of secreted or membrane-bound signals (cytokines, growth factors, etc.) that act on tissue cells to alter their fate or behavior.
この一般的な観点から考えると、リンパ球の中核機能は、組織細胞に作用して細胞の運命や行動を変える分泌シグナルまたは膜結合シグナル (サイトカイン、成長因子など) の局所的な提供として定義できます。
In essence, lymphocytes operate as mobile paracrine/juxtracrine cells that perform tissue surveillance and enforce changes in tissue functional states.
本質的に、リンパ球は、組織監視を実行し、組織の機能状態の変化を強制する移動性パラクリン/ジュクストラクリン細胞として機能します。
This function of lymphocytes may be required when tissue-intrinsic homeostatic mechanisms need to be supplemented or superseded, analogous to the way signals from the endocrine and the autonomic nervous systems are superimposed on tissue homeostatic circuits to impact tissue functional states.
リンパ球のこの機能は、組織固有の恒常性維持機構を補足または置き換える必要がある場合に必要となる場合があります。 これは、内分泌系や自律神経系からの信号が組織の恒常性維持回路に重ね合わされて、組織の機能状態に影響を与えるのと似ています。
As the emergence and diversification of lymphocytes correlate with the increased complexity of vertebrates, one possibility is that tissue surveillance functions are particularly relevant for vertebrate-specific innovations.
リンパ球の出現と多様化は脊椎動物の複雑さの増加と相関しているため、可能性の 1 つは、組織監視機能が脊椎動物特有のイノベーションに特に関連しているということです。
The understanding of the homeostatic functions of lymphocytes is currently limited to examples involving primarily ILCs and innate-like lymphocyte (ILL; TCRs with limited diversity, including γδT cells) populations.
リンパ球の恒常性維持機能の理解は現在、主に ILC および自然様リンパ球 (ILL; γδT 細胞を含む多様性が限られた TCR) 集団を含む例に限定されています。
However, regardless of the lymphocyte type, their mode of operation follows the same principle: they collect information from cells endowed with sensory functions, including dendritic cells, macrophages, epithelial cells, and sensory neurons.
ただし、リンパ球の種類に関係なく、その動作モードは同じ原理に従います:つまり、樹状細胞、マクロファージ、上皮細胞、感覚ニューロンなど、感覚機能を備えた細胞から情報を収集します。
This information is encoded in signals of which best known are the cytokines, such as IL-1, IL-12, IL-33, IL-25, etc.
この情報はシグナルにエンコードされており、IL-1、IL-12、IL-33、IL-25 などのサイトカインが最もよく知られています。
Upon stimulation by these signals, ILCs produce their own set of cytokines (including IL-13, IL-17, IL-22, and IFN-γ) and growth factors (including amphiregulin, keratinocyte growth factor, IGF1, etc.) that orchestrate tissue adaptation to whatever challenge was reported by sensory cells.
これらのシグナルによる刺激を受けると、ILC は独自のサイトカインのセット (IL-13、IL-17、IL-22、および IFN-γ を含む)および成長因子(アンフィレグリン、ケラチノサイト成長因子、IGF1などを含む)を生成します。感覚細胞によって報告されたあらゆる課題に対する組織の適応を調整します。
This includes but is not limited to infections.
これには感染症が含まれますが、これに限定されません。
Such infection-independent functions have been better appreciated in the case of ILCs, which were found to be involved in tissue remodeling, metabolic control, and thermogenesis.
このような感染に依存しない機能は、組織リモデリング、代謝制御、および熱産生に関与していることが判明した ILC の場合によく評価されています。
Similarly, decidual NK cells have specialized functions in the fetal-maternal interface, where they control trophoblast invasion and placental development.
同様に、脱落膜 NK 細胞は胎児と母体の境界面で特殊な機能を持ち、そこで栄養膜浸潤と胎盤の発達を制御します。
Lymphocytes use two types of inputs: the cytokines and other signals produced by sensory cell types and cell surface signals that are typically detected by immunoreceptor tyrosine-based activation motif (ITAM)-coupled or related receptors.
リンパ球は 2 種類の入力を使用します:1 つは感覚細胞タイプによって生成されるサイトカインおよびその他のシグナル、もう 1 つは免疫受容体チロシンベースの活性化モチーフ (ITAM) 共役受容体または関連受容体によって通常検出される細胞表面シグナルです。
One variation on the theme of ITAM-based receptors is the TCRs.
ITAM ベースの受容体のテーマの 1 つのバリエーションは、TCR です。
T cells with restricted TCR diversity, including the γδT cells and NKT cells, have been found to play diverse roles in tissue surveillance and adaptation and in metabolic regulation.
γδT 細胞や NKT 細胞など、TCR の多様性が制限された T 細胞は、組織の監視と適応、および代謝調節において多様な役割を果たすことがわかっています。
Because TCRs of these T cell subsets have limited diversity and thus are specific to a defined set of non-classical major histocompatibility complex (MHC) ligands, they can be developmentally preprogrammed to perform specific tissue surveillance functions upon stimulation by cytokines and TCR ligands.
これらの T 細胞サブセットの TCR は多様性が限られており、したがって定義された一連の非古典的主要組織適合性複合体 (MHC) リガンドに特異的であるため、 これらは、サイトカインやTCRリガンドによる刺激時に特定の組織監視機能を実行するように、発生段階で事前にプログラムすることができます。
For αβT cells with diverse TCR repertoires, known examples of homeostatic functions are still limited and mostly concern Treg cells.
多様な TCR レパートリーを持つ αβT 細胞の場合、恒常性維持機能の既知の例は依然として限られており、ほとんどが Treg 細胞に関するものです。
Tissue-resident Tregs have been shown to play roles in tissue repair, stem cell maintenance, and metabolic regulation in adipose tissue.
組織常在性 Treg は、脂肪組織における組織修復、幹細胞維持、代謝調節において役割を果たすことが示されています。
Thus, a population of Tregs that infiltrate the lean adipose tissue secrete cytokines that directly affect the synthesis of inflammatory mediators and glucose uptake by adipocytes.
したがって、除脂肪組織に浸潤するTregの集団は、炎症性メディエーターの合成と脂肪細胞によるグルコース取り込みに直接影響を与えるサイトカインを分泌します。
Such clonally expanded Tregs express specific TCRs in the visceral adipose tissue (VAT), and Tregs bearing such TCRs are first primed in the spleen and then recruited to the VAT to acquire tissue-specific phenotype.
このようなクローン増殖したTregは内臓脂肪組織(VAT)で特異的なTCRを発現し、そのようなTCRを有するTregはまず脾臓でプライミングされ、次にVATに動員されて組織特異的な表現型を獲得します。
Similarly, Tregs within the injured muscle tissue secrete amphiregulin, which acts directly on muscle satellite cells to promote muscle repair.
同様に、損傷した筋肉組織内の Treg はアンフィレグリンを分泌し、アンフィレグリンは筋肉サテライト細胞に直接作用して筋肉の修復を促進します。
Tregs were also demonstrated to be important for hematopoietic stem cell maintenance and for the regulation of intestinal stem cell differentiation.
Treg は、造血幹細胞の維持と腸幹細胞の分化の調節にとって重要であることも実証されました。
Conventional T cells have also been shown to serve homeostatic functions.
従来の T 細胞も恒常性維持機能を果たすことが示されています。
The role of T cells in the regulation of neuronal development and function was illustrated by several studies.
ニューロンの発達と機能の調節における T 細胞の役割は、いくつかの研究によって説明されています。
In severe combined immunodeficiency and nude mice, adult neurogenesis in the hippocampus is significantly impaired.
重度の複合免疫不全マウスとヌードマウスでは、海馬における成体神経新生が著しく損なわれています。
Transfer of myelin basic protein (MBP)-specific CD4 T cells led to increased neurogenesis and enhanced spatial learning and memory tasks.
ミエリン塩基性タンパク質 (MBP) 特異的 CD4 T 細胞の移入により、神経新生が増加し、空間学習および記憶課題が強化されました。
CD4 T cells were also required for the secretion of the brain-derived neurotrophic factor in the dentate gyrus, implicating the role of these cells in the maintenance of hippocampal plasticity and renewal.
CD4 T細胞は、歯状回における脳由来の神経栄養因子の分泌にも必要でした。 これは、海馬の可塑性の維持と再生におけるこれらの細胞の役割に関係しています。
Another study corroborated these findings by showing that depletion of CD4 T cells, but not CD8 T cells or B cells, resulted in the reduction of hippocampal neurogenesis in adult mice, leading to impaired learning.
別の研究では、成体マウスにおいて、CD8 T細胞やB細胞ではなく、CD4 T細胞の枯渇が海馬の神経新生の減少をもたらし、学習障害につながることを示し、これらの発見を裏付けた。
T cells also play a key role in maintaining normal social behavior and proper neural connectivity within the frontal cortical brain regions.
T細胞は正常な社会的行動の維持、そして前頭皮質脳領域内の適切な神経接続する上でも重要な役割を果たします。
T cells in the meninges secrete IFN-γ, which acts on inhibitory neurons to induce GABAergic currents.
髄膜の T 細胞は IFN-γ を分泌し、抑制性ニューロンに作用して GABA 作動性電流を誘導します。
In the absence of IFN-γ, mice develop social deficits and exhibit hyper-connectivity of frontal cortical brain regions.
IFN-γ が存在しない場合、マウスは社会的欠陥を発症し、前頭皮質脳領域の過剰な接続性を示します。
By contrast, IL-4 secreted from meningeal T cells contributes to learning and memory.
対照的に、髄膜 T 細胞から分泌される IL-4 は学習と記憶に寄与します。
The specificity of T cells that regulate these neurocognitive functions is unknown.
これらの神経認知機能を調節する T 細胞の特異性は不明です。
Beyond the nervous system, CD4 T cells were shown to regulate post-natal organogenesis.
神経系を超えて、CD4 T 細胞が出生後の器官形成を調節することが示されました。
Within the mammary gland, local dendritic cells present antigenic peptides on MHC class II, which induces IFN-γ secretion from CD4 T cells.
乳腺内では、局所の樹状細胞が MHC クラス II 上の抗原ペプチドを提示し、CD4 T 細胞からの IFN-γ 分泌を誘導します。
IFN-γ acts on the mammary epithelial layer to inhibit luminal progenitor differentiation, leading to reduced growth and branching of the mammary gland.
IFN-γは乳腺上皮層に作用して管腔前駆細胞の分化を阻害し、乳腺の成長と分枝の減少につながります。
By contrast, Th2 cell interaction with mammary gland APCs had the opposite outcome, resulting in increased branching.
対照的に、Th2 細胞と乳腺 APC の相互作用は逆の結果をもたらし、分岐が増加しました。
Conventional CD4 T cells may also maintain vascular functions at a steady state because, in the absence of CD4 T cells, the average diameter of the lymph node feed arteriole was constitutively larger than in the wild-type mice.
CD4 T細胞が存在しない場合、リンパ節栄養細動脈の平均直径が野生型マウスよりも構成的に大きかったため、従来のCD4 T細胞も血管機能を定常状態に維持している可能性があります。
Examples of these types of homeostatic functions of αβT cells will likely accumulate in the coming years, but what is becoming clear already is that T cells with diverse TCR repertoires are capable of performing TCR-dependent homeostatic functions that are presumably based on the recognition of self-antigens.
αβT 細胞のこの種の恒常性維持機能の例は、今後数年間で蓄積される可能性があります。 しかし、すでに明らかになりつつあるのは、多様なTCRレパートリーを持つT細胞が、おそらく自己抗原の認識に基づいてTCR依存性の恒常性維持機能を実行できるということです。
This raises several important questions: what is the nature of self-antigens detected by self-reactive T cells and Tregs?
これにより、いくつかの重要な疑問が生じます。自己反応性 T 細胞および Treg によって検出される自己抗原の性質は何でしょうか。
What are the requirements for the activation of self-reactive T cells?
自己反応性 T 細胞を活性化するための要件は何ですか?
Are they distinct from the activation of naive T cells that perform anti-microbial functions?
これらは、抗菌機能を実行するナイーブ T 細胞の活性化とは区別されますか?
Finally, given that both T effector and Treg cells participate in homeostatic processes, do they control distinct or related functions?
最後に、エフェクター T 細胞と Treg 細胞の両方が恒常性維持プロセスに関与しているとすると、それらは別個の機能または関連する機能を制御しているのでしょうか?
Interoception of the immune system
免疫系の内受容感覚
The examples of homeostatic functions of self-reactive T cells, including Tregs, suggest that in addition to the well-appreciated function of the adaptive immune system in sensing the external environment, it may have interoceptive functions that are directed at sensing and regulating some aspects of the internal environment.
Treg を含む自己反応性 T 細胞の恒常性維持機能の例は、外部環境を感知する適応免疫系の十分に評価されている機能に加えて、 内部環境のいくつかの側面を感知して調節することを目的とした内受容機能を備えている可能性があります。
A key question arising from these observations is how is the self-reactivity of these T cells related to their mode of function?
これらの観察から生じる重要な疑問は、これらの T 細胞の自己反応性がその機能様式にどのように関連しているのかということです。
Although it is easy enough to envision how ILCs and γδT cells are involved in internal affairs, how and why would T cells with diverse TCR repertoires be engaged in such functions?
ILC と γδT 細胞が内部事情にどのように関与しているかを想像するのは容易ですが、多様な TCR レパートリーを持つ T 細胞はどのように、そしてなぜそのような機能に関与するのでしょうか?
There is still too much unknown about this aspect of lymphocyte biology, but here we will suggest a hypothetical scenario in the hope that, if nothing else, it could provoke some debate.
リンパ球生物学のこの側面についてはまだ不明な点が多すぎますが、ここでは、少なくとも何らかの議論を引き起こす可能性があることを期待して、仮説のシナリオを提案します。
We propose that in addition to the effector T cells involved in canonical defense functions, there is a class of autoreactive T cells that avoid negative selection by design, in order to perform homeostatic, interoceptive functions.
私たちは、標準的な防御機能に関与するエフェクター T 細胞に加えて、恒常性の内部受容機能を実行するために、設計により負の選択を回避する自己反応性 T 細胞のクラスが存在すると提案します。
To distinguish them from conventional effector T cells and Tregs, we will refer to them here as Tx cells.
従来のエフェクター T 細胞や Treg と区別するために、ここではそれらを Tx 細胞と呼びます。
This designation is only for convenience at this point, as it is currently unknown if they represent a distinct T cell population, although the arguments we present below suggest that they might.
これらが別個の T 細胞集団を表すかどうかは現時点では不明であるため、この指定は現時点では便宜上のものに過ぎませんが、以下に示す議論はその可能性を示唆しています。
We suggest that Tx cells are specific for a subset of self-antigens derived from proteins expressed in cell-type- or cell-state-specific manner, as this could allow Tx cells to monitor cellular and tissue homeostatic and functional states.
私たちは、Tx細胞が細胞型または細胞状態特異的に発現するタンパク質に由来する自己抗原のサブセットに特異的であることを示唆しています。これにより、Tx細胞は細胞および組織の恒常性および機能状態をモニタリングできる可能性があります。
Expression of tissue-specific antigens in the thymic epithelial cells is likely enabled by AIRE, and in addition, tissue-mimetic cells in the thymus may select Tx cells specific for antigens expressed during specific stages of development and differentiation (Figure 3).
胸腺上皮細胞における組織特異的抗原の発現は、AIRE によって可能になる可能性が高く、さらに、胸腺の組織模倣細胞は、発生および分化の特定の段階で発現される抗原に特異的な Tx 細胞を選択する可能性があります (図 3)。
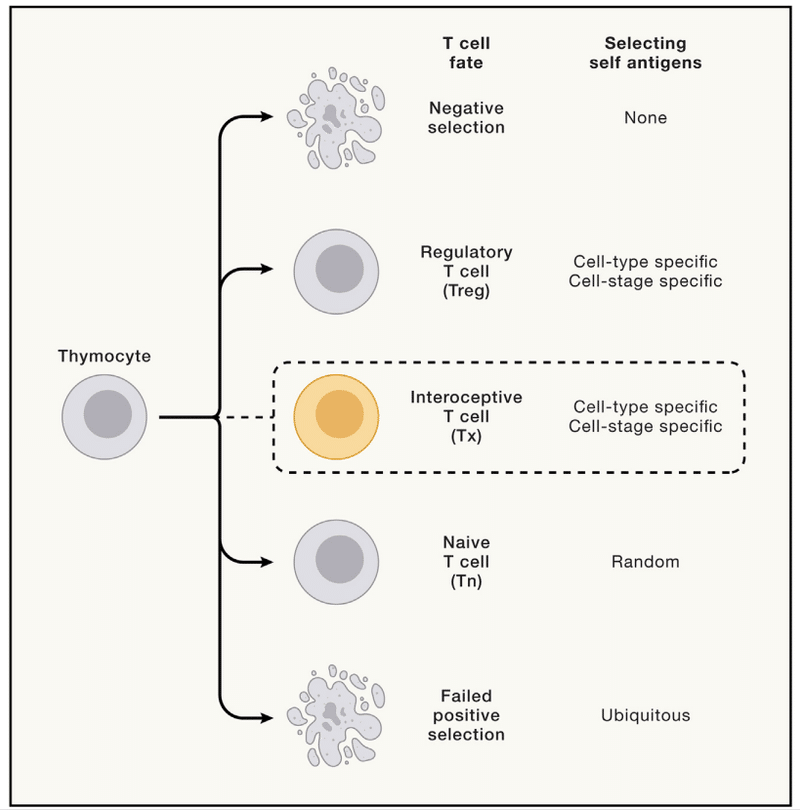
Consistent with this notion, autoreactive T cells are present in circulation in healthy humans.
この概念と一致して、自己反応性 T 細胞は健康な人間の循環中に存在します。
At least some of these self-reactive T cells might be Tx cells.
これらの自己反応性 T 細胞の少なくとも一部は Tx 細胞である可能性があります。
Tregs may have distinct or related self-antigen specificities, as they are presumably selected for their relatively high TCR affinity to cell-type-specific self-antigens.
Treg はおそらく細胞型特異的自己抗原に対する比較的高い TCR 親和性によって選択されるため、異なる自己抗原特異性または関連する自己抗原特異性を有する可能性があります。
The homeostatic functions of Tx cells appear to be based, at least in part, on the same effector mechanisms utilized by the canonical T cells.
Tx 細胞の恒常性機能は、標準的な T 細胞によって利用されるのと同じエフェクター機構に少なくとも部分的に基づいているようです。
Indeed, in the examples cited above, Tx functions are mediated by IL-4 and IFN-γ, and cytotoxic Tx cells presumably perform homeostatic pruning of unwanted cells.
実際、上で引用した例では、Tx 機能は IL-4 および IFN-γ によって媒介されており、細胞傷害性 Tx 細胞はおそらく不要な細胞の恒常的な剪定を行っていると考えられます。
Indeed, T cell-derived cytokines can control a multitude of tissue functions, and cell-type- or state-specific antigen-dependent production of these cytokines would conceivably allow Tx and Treg cells to make their effect contingent on tissue functional states.
実際、T 細胞由来のサイトカインは多数の組織機能を制御することができ、細胞型または状態特異的な抗原依存性のこれらのサイトカインの産生により、Tx 細胞と Treg 細胞がその効果を組織の機能状態に依存させることができると考えられます。
Given that both Tx and Treg cells perform tissue regulatory functions, what is the difference between them?
Tx 細胞と Treg 細胞の両方が組織調節機能を実行するとすると、それらの違いは何ですか?
We suggest that the functional relation between these cells is analogous to the relation of Tregs and canonical T effector cells involved in host defense.
これらの細胞間の機能的関係は、宿主防御に関与するTregと標準的なTエフェクター細胞の関係に類似していると考えられます。
Specifically, cytokines produced by Tx cells enforce some functional changes in tissues, and Tregs oppose these changes (Figure 4).
具体的には、Tx 細胞によって産生されるサイトカインは組織に何らかの機能変化を強制し、Treg はこれらの変化に反対します (図 4)。
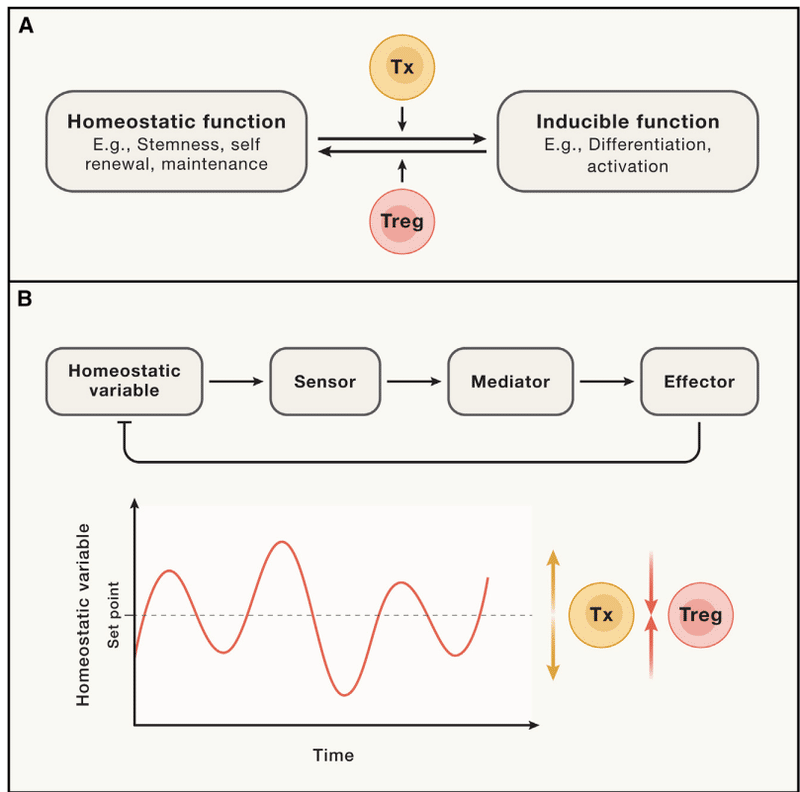
So, for example, if Tx-derived cytokines promote stem cells’ transition to differentiation, Treg cells oppose that effect, thus preserving the stem cell pool.
したがって、たとえば、Tx 由来のサイトカインが幹細胞の分化への移行を促進する場合、Treg 細胞はその効果に対抗し、幹細胞プールを維持します。
Whatever Tx cells do, whether it is promoting collagen production, inducing metabolic changes, tissue remodeling, etc., Treg cells simply suppress, or oppose, these functions, thus maintaining a balance, just as they do with effector T cells in the context of anti-microbial defense.
Tx細胞が何をするとしても、それがコラーゲン産生の促進、代謝変化の誘導、組織再構築などであっても、Treg細胞は単にこれらの機能を抑制または反対するだけです。 したがって、抗菌防御の文脈でエフェクター T 細胞が行うのと同じように、バランスを維持します。
Such functions would exactly parallel the effects of inflammatory and anti-inflammatory signals on homeostasis in general.
このような機能は、一般に恒常性に対する炎症性および抗炎症性シグナルの影響とまったく同じであると考えられます。
Furthermore, we suggest that dysregulation of Tx functions results in a cluster of immune-mediated diseases, including autoimmune diseases.
さらに、Tx 機能の調節不全が自己免疫疾患を含む免疫介在性疾患のクラスターを引き起こすことを示唆します。
Indeed, autoimmune diseases can be thought of as exaggerated versions of normal developmental and homeostatic functions.
実際、自己免疫疾患は、正常な発達および恒常性維持機能の誇張されたバージョンと考えることができます。
For example, synovial macrophages normally differentiate into osteoclasts under the control of RANKL and colony-stimulating factor-1 produced by fibroblasts.
たとえば、滑膜マクロファージは通常、RANKL および線維芽細胞によって産生されるコロニー刺激因子 1 の制御下で破骨細胞に分化します。
This normally helps maintain the balance of matrix deposition and erosion.
これは通常、マトリックスの堆積と侵食のバランスを維持するのに役立ちます。
Autoreactive T cells can promote osteoclast differentiation by stimulating synovial fibroblasts with TNF and IL-1β.
自己反応性 T 細胞は、TNF と IL-1β で滑膜線維芽細胞を刺激することにより、破骨細胞の分化を促進します。
This mechanism is only appreciated when it is exaggerated in the context of rheumatoid arthritis, but we would posit that it takes place under some normal conditions, on a much smaller scale, when it is less conspicuous.
このメカニズムは、関節リウマチの文脈で誇張された場合にのみ理解されますが、それがあまり目立たない通常の条件下では、はるかに小規模で起こっていると考えられます。
Thus, autoreactive T cells may just be a dysregulated version of Tx cells, and homeostatic Tregs may normally constrain their activity.
したがって、自己反応性 T 細胞は単に Tx 細胞の調節不全バージョンである可能性があり、通常は恒常性 Treg がその活性を抑制している可能性があります。
Similarly, autoreactive, pathological CD4 T cells in the context of multiple sclerosis, may be a dysregulated version of Tx cells that control the normal process of demyelination mediated by microglial cells.
同様に、多発性硬化症における自己反応性の病的 CD4 T 細胞は、ミクログリア細胞によって媒介される正常な脱髄プロセスを制御する Tx 細胞の調節不全バージョンである可能性があります。
Indeed, MBP-reactive CD4 T cells are found in the cerebrospinal fluid of healthy humans at similar frequencies to patients with multiple sclerosis.
実際、MBP 反応性 CD4 T 細胞は、多発性硬化症患者と同様の頻度で健康なヒトの脳脊髄液中に存在します。
Recognition of myelin antigens by Tx cells may be a way to monitor the requirements for a homeostatic balance of formation and removal of myelin sheaths formed by oligodendrocytes.
Tx 細胞によるミエリン抗原の認識は、希突起膠細胞によって形成されるミエリン鞘の形成と除去の恒常性バランスの要件を監視する方法である可能性があります。
It may be an excessive performance of this function that leads to pathological demyelination.
この機能の過剰なパフォーマンスが病的な脱髄につながる可能性があります。
From this perspective, some autoantigens are the self-antigens recognized by Tx cells for homeostatic functions, and it is a dysregulated activity of Tx cells that results in pathological outcomes of autoimmune diseases.
この観点から、一部の自己抗原は恒常性維持機能のために Tx 細胞によって認識される自己抗原であり、自己免疫疾患の病理学的結果をもたらす Tx 細胞の活性調節異常です。
This would explain why autoimmune diseases target some and spare other organs.
これは、自己免疫疾患が一部の臓器を標的にし、他の臓器を温存する理由を説明するでしょう。
This perspective may also ultimately explain why the immune system attacks some cancers and not others.
この観点は、免疫系が一部のがんを攻撃し、他のがんを攻撃しない理由を最終的に説明する可能性もあります。
A related scenario involves the detection of mutated self-antigens that report on the secretory function of endocrine cells, aimed at eliminating hyper-secreting mutant clones.
関連するシナリオには、内分泌細胞の分泌機能を報告する変異自己抗原の検出が含まれ、過剰分泌変異体クローンの排除を目的としています。
An important aspect of this mechanism is that it explains why some but not other endocrine tissues are targeted in autoimmune diseases, based on the presence or absence of control mechanisms that would allow expansion of hyper-secreting mutant clones.
このメカニズムの重要な側面は、過剰分泌変異体クローンの増殖を可能にする制御機構の有無に基づいて、なぜ一部の内分泌組織が自己免疫疾患の標的となるのか、他の内分泌組織が標的にならないのかを説明しているということです。
Other autoantigens may be targeted by unrelated mechanisms, including cross-reactivity with microbial antigens derived from pathogens or commensals, or mimicking microbial repetitive epitope arrangement by cell surface protein complexes, as may be the case with acetylcholine receptor targeting by antibodies in myasthenia gravis.
他の自己抗原は、病原体または共生生物に由来する微生物抗原との交差反応性、または細胞表面タンパク質複合体による微生物の反復エピトープ配列の模倣など、無関係なメカニズムによって標的化される可能性があります。 重症筋無力症における抗体によるアセチルコリン受容体標的化の場合も同様です。
The Bx cells, what we would propose as the B cell counterpart of Tx cells, are also self-reactive and perform homeostatic functions, such as the clearance of apoptotic cells and other unwanted materials.
Tx 細胞の B 細胞相当物として我々が提案する Bx 細胞も自己反応性であり、アポトーシス細胞やその他の不要な物質の除去などの恒常性維持機能を実行します。
An example of Bx cells could be the B-1 cells (or a subset thereof), which are selected for self-reactivity, including reactivity toward antigens expressed by apoptotic cells such as Annexin IV and phosphatidylcholine, and participate in the clearance of dead cells.
Bx 細胞の例としては、B-1 細胞 (またはそのサブセット) が挙げられます。 これらは、アネキシン IV やホスファチジルコリンなどのアポトーシス細胞によって発現される抗原に対する反応性を含む自己反応性によって選択され、死んだ細胞のクリアランスに関与します。
Natural IgM that recognizes dsDNA or cardiolipin in lupus patients correlates with a lack of nephritis, suggestive of a protective role of such antibodies.
狼瘡患者のdsDNAまたはカルジオリピンを認識する天然IgMは、腎炎の欠如と相関しています。 このような抗体の保護的役割を示唆しています。
On the other hand, IgG antibodies with the same specificities are hallmark pathological autoantibodies in systemic lupus erythematosus.
一方、同じ特異性を持つ IgG 抗体は、全身性エリテマトーデスにおける特徴的な病理学的自己抗体です。
Thus, antibody-mediated autoimmunity might be an aberrant version of the homeostatic functions of self-reactive antibodies, which in some cases may result from a class switch to a “wrong” isotype.
したがって、抗体媒介自己免疫は自己反応性抗体の恒常性維持機能の異常版である可能性があり、場合によっては「間違った」アイソタイプへのクラススイッチが原因である可能性があります。
Although some unexplained phenomena could be interpreted from the perspective presented here, it requires direct experimental testing.
一部の説明不能な現象は、ここで示した観点から解釈できますが、直接的な実験的テストが必要です。
While emerging technologies that allow analyses of rare tissue-resident T cells and their TCRs will help to define unique features of autoreactive T cells, the ultimate “deletion test” would require exploring the consequences of specific elimination of Tx cells, as the mutant animals with a total absence of lymphocytes are confounded by severe susceptibility to infections, which makes them unsuitable for the studies of most homeostatic functions.
希少な組織常在性 T 細胞とその TCR の分析を可能にする新興技術は、自己反応性 T 細胞のユニークな特徴を明らかにするのに役立ちますが、 究極の「欠失試験」では、Tx 細胞の特異的除去の結果を調査する必要があるでしょう。 リンパ球が完全に欠如した変異動物は、感染症に対する重篤な感受性によって混乱しており、ほとんどの恒常性機能の研究には適さないためです。
Conclusions and future outlook
The immune system continues to be fraught with many mysteries.
免疫システムには依然として多くの謎が残されています。
Not all of them can be solved by the traditional reductionist approaches because some of these mysteries are undoubtedly reflections of emergent properties arising from nonlinear phenomena operating at the level of cell populations.
これらの謎の一部は間違いなく、細胞集団のレベルで作用する非線形現象から生じる創発的な特性を反映しているため、それらのすべてが従来の還元主義的なアプローチで解決できるわけではありません。
It is not possible to understand these properties by studying individual components in isolation, just as it is impossible to understand how termites build their mounds by studying the individual insects.
個々の昆虫を研究することによってシロアリがどのように塚を築くのかを理解することが不可能であるのと同様に、個々のコンポーネントを個別に研究することによってこれらの特性を理解することは不可能です。
Indeed, the immune system can be viewed as a system with crowd intelligence, where the collective actions and interactions of individual cells contribute to the system’s overall adaptability and effectiveness in recognizing and combating threats (microbial or otherwise) and maintaining internal order.
実際、免疫システムは群集知性を備えたシステムとみなすことができます。 ここでは、個々の細胞の集団的な行動と相互作用が、脅威(微生物など)を認識してこれと闘い、内部秩序を維持する際のシステム全体の適応性と有効性に貢献します。
The transition from the traditional cross-sectional “one cell at a time” approaches to collective cell behavior studies, such as through longitudinal live imaging techniques coupled with machine learning analyses, will transform our ability to appreciate the immunological design principles further.
従来の「一度に1細胞」の断面アプローチから、縦断ライブイメージング技術と機械学習解析を組み合わせたような集合的な細胞挙動研究への移行により、免疫学的設計原理を理解する能力がさらに変化するでしょう。
Another important consideration is individual variation of the immune responses.
もう 1 つの重要な考慮事項は、免疫応答の個人差です。
The enigma of individual susceptibility to disease outcomes resulting from a specific infection remains a medically significant puzzle.
特定の感染症に起因する疾患結果に対する個人の感受性の不可解さは、医学的に重要な謎のままです。
Systems immunology analyses have revealed that the heterogeneity of human immune responses to vaccines is largely driven by the baseline immune signatures of an individual.
システム免疫学の分析により、ワクチンに対するヒトの免疫応答の不均一性は、主に個人のベースライン免疫サインによって左右されることが明らかになりました。
Based on studies of twins, genetics alone cannot explain this heterogeneity in vaccination outcomes in adults.
双子の研究に基づくと、成人のワクチン接種結果におけるこの不均一性は遺伝学だけでは説明できません。
A goal is to understand the key parameters of baseline immunological tone and to leverage this information in forecasting disease susceptibility and anticipating health outcomes after medical intervention.
目標は、ベースラインの免疫学的調子の重要なパラメーターを理解し、病気の感受性を予測し、医療介入後の健康状態を予測する際にこの情報を活用することです。
Furthermore, emerging areas of intersection between immunology and artificial intelligence, metabolism, nutrition, nervous system, and microbiome promise to deliver new insights into how the immune system operates; what causes it to malfunction; and how it can be manipulated to combat diseases including infections, autoimmunity, and neurodegenerative diseases.
さらに、免疫学と人工知能、代謝、栄養、神経系、マイクロバイオームが交わる新たな分野は、免疫システムがどのように機能するかについて新たな洞察をもたらすと期待されています; 誤作動の原因は何か;そして感染症、自己免疫、神経変性疾患などの病気と闘うためにそれをどのように操作できるのか。
Finally, the COVID-19 pandemic has taught us that a so-called acute respiratory pathogen can do so much more—invade multiple organs, establish viral reservoirs, induce latent herpesvirus reactivation, induce hypercoagulability, damage organs, trigger hormonal imbalances, and increase the risk for neurological, neurodegenerative, pulmonary, cardiovascular, and autoimmune diseases.
最後に、COVID-19パンデミックは、いわゆる急性呼吸器病原体がさらに多くのことを引き起こす可能性があることを私たちに教えてくれました—複数の臓器に侵入し、ウイルスの貯蔵庫を確立し、潜伏ヘルペスウイルスの再活性化を誘発し、凝固亢進を誘発し、臓器に損傷を与え、ホルモンの不均衡を引き起こし、神経疾患、神経変性疾患、肺疾患、心血管疾患、および自己免疫疾患のリスクを高めます。
About 10% of the infected develop long-term health problems known as long COVID.
感染者の約 10% はロングCOVIDとして知られる長期的な健康上の問題を発症します。
For centuries, other pathogens have caused post-acute infection illnesses resembling long COVID, but these medically unexplained syndromes have not been well accepted or widely studied.
何世紀にもわたって、他の病原体がロングCOVIDに似た急性感染症後の疾患を引き起こしてきましたが、これらの医学的に説明のつかない症候群は十分に受け入れられておらず、広く研究されていませんでした。
Long COVID is a pandemic after the pandemic, and with over 65 million suffering, it remains an unresolved medical mystery that needs to be studied from every angle, including immunology.
ロングCOVIDはパンデミックに次ぐパンデミックであり、6,500万人以上が苦しんでおり、依然として未解決の医学的謎であり、免疫学を含むあらゆる角度から研究する必要があります。
Testing the assumptions and refining the conceptual foundations is how immunology can take on these urgent and persistent challenges.
仮説を検証し、概念的な基礎を洗練することは、免疫学がこれらの緊急かつ永続的な課題に取り組む方法です。
以下省略。
///////////////////////////////////////////////////////////////////////////
Box 1. Glossary
用語集
BCR:
B cell receptor—the antigen receptor of B cells—is a transmembrane complex composed of two heavy and two light chains. Antibodies are the secreted version of BCR.
B 細胞受容体 (B 細胞の抗原受容体) は、2 つの重鎖と 2 つの軽鎖で構成される膜貫通複合体です。抗体はBCRの分泌型です。
B-1 cells:
these B cells belong to a distinct lineage of fetal-derived B cells that play a role in the early immune response to pathogens. B-1 cells express IgM, reside in various body cavities, and spontaneously produce natural antibodies. Natural antibodies have limited diversity and have broad reactivity to classes of bacterial and viral pathogens, as well as self-antigens.
これらの B 細胞は、病原体に対する初期の免疫応答に役割を果たす胎児由来 B 細胞の異なる系統に属しています。 B-1 細胞は IgM を発現し、さまざまな体腔に存在し、自発的に天然抗体を産生します。天然の抗体は多様性が限られており、細菌性およびウイルス性の病原体、さらには自己抗原のクラスに対して幅広い反応性を持っています。
B-2 cells:
B-2 cells are the ‘‘conventional’’ B cells that develop in the bone marrow postnatally, express highly diverse B cell receptors, and are responsible for infection- and vaccine-induced antibody responses. With T cell help, B-2 cells can undergo somatic hypermutation to further diversify BCR sequences. High-affinity B cell receptor-bearing B-2 cells are selected within the germinal centers, resulting in affinity maturation.
B-2 細胞は「従来の」B 細胞で、出生後に骨髄で発生し、非常に多様な B 細胞受容体を発現し、感染症やワクチンによって誘導される抗体反応を担当します。 T 細胞の助けにより、B-2 細胞は体細胞超突然変異を起こし、BCR 配列をさらに多様化することができます。高親和性 B 細胞受容体を持つ B-2 細胞が胚中心内で選択され、親和性が成熟します。
Bx cells:
these are a hypothetical subset of B cells that are self-reactive by design and perform interoceptive homeostatic functions in various tissues. Subsets of B-1 cells are possible candidates for the Bx cells.
これらは、設計上自己反応性であり、さまざまな組織において内受容恒常性機能を実行する B 細胞の仮説的なサブセットです。 B-1 細胞のサブセットが Bx 細胞の候補となる可能性があります。
Conventional T cells: 従来の T 細胞
these T cells bear highly diverse TCRab chains that detect peptides presented by MHC molecules. CD8 T cells recognize peptides derived from intracellular proteins presented on MHC class I and kill virally infected or otherwise unwanted cells, while CD4 T cells recognize peptides presented on MHC class II derived from extracellular or transmembrane proteins. CD4 T cells come in many flavors, including T helper type 1 (Th1), Th2, and Th17 subsets that combat intracellular pathogens, extracellular parasites, and extracellular bacteria and fungi, respectively.
これらの T 細胞は、MHC 分子によって提示されるペプチドを検出する非常に多様な TCRab 鎖を持っています。 CD8 T細胞は、MHCクラスIに存在する細胞内タンパク質に由来するペプチドを認識し、ウイルス感染細胞やその他の不要な細胞を死滅させますが、CD4 T細胞は、MHCクラスIIに存在する細胞外タンパク質または膜貫通タンパク質に由来するペプチドを認識します。 CD4 T 細胞には、それぞれ細胞内病原体、細胞外寄生虫、細胞外細菌および真菌と戦う T ヘルパー 1 型 (Th1)、Th2、および Th17 サブセットなど、さまざまな種類があります。
Exaptation: 外適用
this trait is initially evolved for one function and may be later repurposed for a new function.
この特性は、最初は 1 つの機能のために進化し、後で新しい機能に再利用される可能性があります。
δγT cells:
these are innate-like lymphocytes that express TCRg and TCRd chains that detect phosphoantigens, lipid antigens, and stress-induced ligands.
これらは、リン酸化抗原、脂質抗原、およびストレス誘導性リガンドを検出する TCRg および TCRd 鎖を発現する自然様リンパ球です。
Innate lymphoid cells:
These lymphoid cells are devoid of antigen receptors that provide early immune responses and homeostatic functions in various organs. ILCs are classified into three subsets with the capacity to secrete distinct cytokines: group 1 ILC including ILC1 and NK cells (IFN-g); group 2 ILC, ILC2 (IL-4/IL-5/IL-13); and group 3 ILCs, ILC3 and LTi (IL-17/IL-22).
これらのリンパ細胞には、さまざまな臓器に初期の免疫応答と恒常性維持機能を提供する抗原受容体がありません。 ILC は、異なるサイトカインを分泌する能力を持つ 3 つのサブセットに分類されます: グループ 1 ILC=ILC1 および NK 細胞 (IFN-g) 。グループ 2 ILC=ILC2 (IL-4/IL-5/IL-13)。グループ 3 ILC=ILC3 および LTi (IL-17/IL-22)。
Innate-like lymphocytes: 自然様リンパ球
these group of lymphocytes express antigen receptors of invariant or limited diversity. These include NKT cells, MAIT cells, gdT cells, B-1 cells, and marginal zone B cells.
これらのリンパ球グループは、不変または限られた多様性の抗原受容体を発現します。これらには、NKT 細胞、MAIT 細胞、gdT 細胞、B-1 細胞、および辺縁帯 B 細胞が含まれます。
Interoception:
interoception denotes perceiving, interpreting, and acting on signals from within the body And plays a key role in maintaining homeostasis.
内受容は、体内からの信号を知覚、解釈し、作用することを意味し、恒常性の維持に重要な役割を果たします。
Mucosal-associated invariant T (MAIT) cells: 粘膜関連不変 T (MAIT) 細胞
these innate-like lymphocytes express semi-invariant TCRs that recognize vitamin B metabolites presented on the MHC class I-like molecule, MR1.
これらの自然様リンパ球は、MHC クラス I 様分子 MR1 上に存在するビタミン B 代謝産物を認識する半不変 TCR を発現します。
Naive lymphocytes:
these T and B lymphocytes have not yet undergone clonal selection, express specific antigen receptors, and circulate through various secondary lymphoid structures in search of their antigens.
これらの T および B リンパ球はまだクローン選択を受けておらず、特定の抗原受容体を発現し、抗原を求めてさまざまな二次リンパ球を循環します。
Natural killer T (NKT) cells:
these innate-like lymphocytes express TCRs with limited diversity and detect lipid antigens presented by the non-classical MHC class I molecule, CD1d.
これらの自然様リンパ球は、多様性が限られた TCR を発現し、非古典的な MHC クラス I 分子である CD1d によって提示される脂質抗原を検出します。
Pattern-recognition receptors: パターン認識受容体
these receptors recognize evolutionarily conserved pathogen-associated molecular patterns (PAMPs). Examples of PRRs include the transmembrane Toll-like receptors that detect extracellular and vacuolar PAMPs (lipopolysaccharide, peptidoglycan, and double-stranded RNA [dsRNA]) and intracellular nucleic acid sensors, including RIG-I and MDA5 that detect cytosolic viral RNA and cGAS that detects cytosolic DNA.
これらの受容体は、進化的に保存された病原体関連分子パターン (PAMP) を認識します。 PRR の例には、細胞外および空胞 PAMP (リポ多糖、ペプチドグリカン、および二本鎖 RNA [dsRNA]) を検出する膜貫通型の Toll 様受容体や細胞内核酸センサーが含まれます。 これには、サイトゾルのウイルス RNA を検出する RIG-I と MDA5、およびサイトゾルの DNA を検出する cGAS が含まれます。
Semantic feature:
here, ‘‘semantic features’’ are used to indicate parsing of information into distinct categories based on their meaning for the immune system.
ここで、「意味論的特徴」は、免疫システムにとっての意味に基づいて情報を個別のカテゴリーに解析することを示すために使用されます。
T help:
CD4 T cells in the secondary lymphoid tissues known as T follicular helper (TFH) cells provide ‘‘help’’ to B cells to become activated, proliferate, and differentiate. T help is needed for B cells to undergo class switching (e.g., going from IgM to IgG, IgA, or IgE) and affinity maturation (B cell receptors undergo somatic hypermutations and selection of higher affinity BCRs) and become memory B cells and antibody-secreting cells.
濾胞性ヘルパーT (TFH) 細胞として知られる二次リンパ組織内の CD4 T 細胞は、B 細胞が活性化、増殖、分化する「助け」を提供します。B 細胞がクラススイッチ (IgM から IgG、IgA、または IgE へ移行するなど) と親和性成熟 (B 細胞受容体は体細胞超突然変異とより高い親和性 BCR の選択を受ける) を受けるには、補助が必要です。 そして記憶B細胞と抗体分泌細胞になります。
Thymic selection: 胸腺の選択
this is a process by which developing T cells undergo a series of selection processes within the thymus to ensure that they possess functional T cell receptors capable of recognizing foreign antigens presented by MHC molecules (positive selection) while avoiding recognition of self-antigens (through negative selection).
これは、発生中の T 細胞が一連の選択プロセスを受けるプロセスです 胸腺内で自己抗原の認識を回避しながら(ネガティブ選択による)MHC分子によって提示される外来抗原を認識できる(ポジティブ選択)機能的なT細胞受容体を確実に保有するようにするためです。
Treg:
these are regulatory T cells that suppress excessive immune responses and maintain immune tolerance.
これらは過剰な免疫反応を抑制し、免疫寛容を維持する制御性 T 細胞です。
Tx cells:
these are a hypothetical subset of T cells that are self-reactive by design and perform interoceptive homeostatic functions in various tissues. Examples include control of tissue surveillance, pruning of unwanted cells, cell activation, differentiation, and tissue remodeling. this subset may comprise T cells of fetal origin.
これらは、設計により自己反応性であり、さまざまな組織において内受容恒常性機能を実行する、T 細胞の仮説的なサブセットです。例には、組織監視の制御、不要な細胞の刈り込み、細胞の活性化、分化、および組織のリモデリングが含まれます。このサブセットは胎児由来の T 細胞を含む可能性があります。
VDJ recombination:
a key process in generating antigen receptors during lymphocyte development, by rearranging gene segments encoding variable (V), diversity (D), and joining (J) regions of immunoglobulin (in B cells) and T cell receptor (in T cells). This random process of recombination enables the expression of diverse antigen receptors in lymphocytes.
免疫グロブリン(B細胞内)およびT細胞受容体(T細胞内)の可変(V)、多様性(D)、結合(J)領域をコードする遺伝子セグメントを再構成することによって、リンパ球の発生中に抗原受容体を生成する重要なプロセスです。 このランダムな組換えプロセスにより、リンパ球における多様な抗原受容体の発現が可能になります。
///////////////////////////////////////////////////////////////////////////
Box 2.
Examples of possible input variables for the innate and adaptive immune recognition that may matter for the generation of immune responses. These variables are divided based on their semantic features—each variable has a different meaning for the immune system. The asterisks mark characteristics unique to self-antigens.
免疫応答の生成に重要となる可能性のある、自然免疫認識および適応免疫認識のための可能な入力変数の例。これらの変数は、意味論的な特徴に基づいて分類されます。各変数は免疫システムにとって異なる意味を持ちます。アスタリスクは自己抗原に特有の特徴を示します。
INNATE IMMUNE RECOGNITION 自然免疫の認識
Microbial or not 微生物かどうか
Pathogenic or not 病原性があるかどうか
Type of pathogenicity 病原性の種類
Viability 生存可能性
Quantity 量
Site of entry エントリーの場所
Site of replication 複製の場所
Site of transmission 感染場所
Location—intra- or extra-cellular 場所 - 細胞内または細胞外
Replication rate レプリケーション速度
ANTIGEN-BASED RECOGNITION 抗原ベースの認識
Self or non-self 自己か非自己か
Valency 結合価
Epitope spacing and density エピトープの間隔と密度
Chemical nature 化学的性質
Abundance and stability 豊富さと安定性
Rate of change in quantity 数量の変化率
Ubiquitous or tissue-restricted* 遍在性または組織限定*
Expression dynamics (constitutive, inducible)
発現ダイナミクス (構成的、誘導的)
Factors that control expression level 発現レベルを制御する因子
Expression in the thymus* 胸腺における発現*
///////////////////////////////////////////////////////////////////////////
この記事が気に入ったらサポートをしてみませんか?