JN.1由来の SLip、FLiRT、KP.2変異体の中和回避、感染性、膜融合
Neutralization escape, infectivity, and membrane fusion of JN.1-derived SARS-CoV-2 SLip, FLiRT, and KP.2 variants
JN.1由来のSARS-CoV-2 SLip、FLiRT、KP.2変異体の中和回避、感染性、膜融合
Highlights
SLip, FLiRT, and KP.2 are poorly neutralized by bivalent-vaccinated sera
SLip、FLiRT、および KP.2 は、二価ワクチンを接種した血清によって中和されにくい
XBB.1.5-vaccinated hamster and JN.1 patient sera neutralized SLip, FLiRT, and KP.2
XBB.1.5 を接種したハムスターおよび JN.1 患者の血清は、SLip、FLiRT、および KP.2 を中和した
S mutations R346T, L455S, and F456L alter ACE2 binding and neutralization epitopes
S 変異 R346T、L455S、および F456L は、ACE2 結合および中和エピトープを変化させる
SLip, FLiRT, and KP.2 spikes exhibit less fusion and processing relative to JN.1
SLip、FLiRT、および KP.2 スパイクは、JN.1 と比較して融合および処理が少ない
Summary
We investigate JN.1-derived subvariants SLip, FLiRT, and KP.2 for neutralization by antibodies in vaccinated individuals, severe acute respiratory syndrome coronavirus 2 (SARS-CoV-2)-infected patients, or class III monoclonal antibody S309.
ワクチン接種を受けた個人、重症急性呼吸器症候群コロナウイルス 2 (SARS-CoV-2) に感染した患者、またはクラス III モノクローナル抗体 S309 における抗体による中和について、JN.1 由来のサブバリアント SLip、FLiRT、および KP.2 を調査します。
Compared to JN.1, SLip, KP.2, and especially FLiRT exhibit increased resistance to bivalent-vaccinated and BA.2.86/JN.1-wave convalescent human sera.
JN.1 と比較して、SLip、KP.2、特に FLiRT は、二価ワクチン接種および BA.2.86/JN.1 波回復期ヒト血清に対する耐性が高まっています。
XBB.1.5 monovalent-vaccinated hamster sera robustly neutralize FLiRT and KP.2 but have reduced efficiency for SLip.
XBB.1.5 一価ワクチン接種ハムスター血清は、FLiRT および KP.2 を強力に中和しますが、SLip に対する効率は低下しています。
All subvariants are resistant to S309 and show decreased infectivity, cell-cell fusion, and spike processing relative to JN.1.
すべてのサブバリアントは S309 に対して耐性があり、JN.1 と比較して感染性、細胞間融合、およびスパイク処理が低下しています。
Modeling reveals that L455S and F456L in SLip reduce spike binding for ACE2, while R346T in FLiRT and KP.2 strengthens it.
モデル化により、SLip の L455S と F456L は ACE2 のスパイク結合を減少させ、FLiRT と KP.2 の R346T はスパイク結合を強化することが明らかになりました。
These three mutations, alongside D339H, alter key epitopes in spike, likely explaining the reduced sensitivity of these subvariants to neutralization.
これら 3 つの変異は、D339H とともにスパイクの重要なエピトープを変化させ、これらのサブバリアントの中和に対する感受性の低下を説明するものと考えられます。
Our findings highlight the increased neutralization resistance of JN.1 subvariants and suggest that future vaccine formulations should consider the JN.1 spike as an immunogen, although the current XBB.1.5 monovalent vaccine could still offer adequate protection.
私たちの調査結果は、JN.1 サブバリアントの中和耐性の増加を強調しており、現在の XBB.1.5 単価ワクチンでも十分な保護を提供できるものの、将来のワクチン製剤では JN.1 スパイクを免疫原として考慮する必要があることを示唆しています。
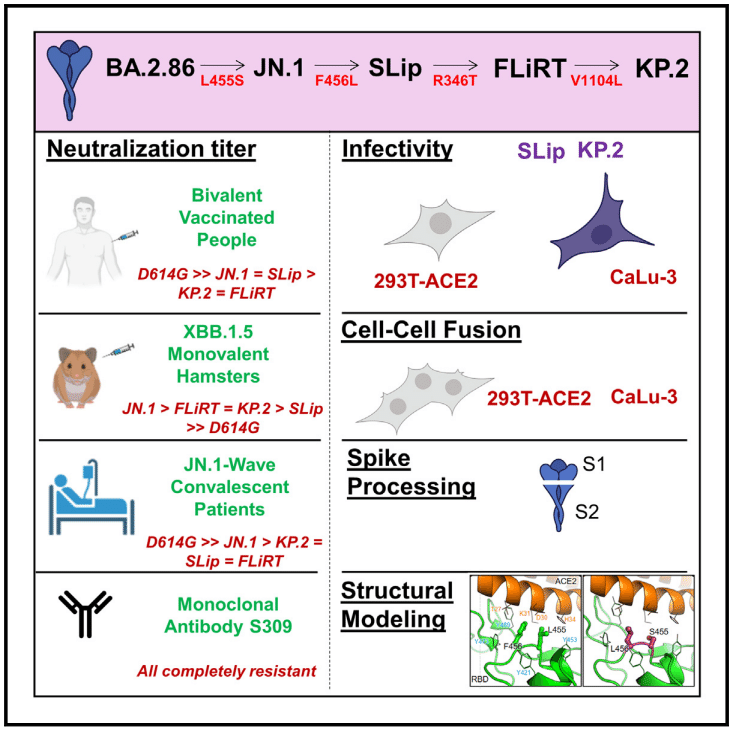
Introduction
Tracking the ongoing evolution of severe acute respiratory syndrome coronavirus 2 (SARS-CoV-2) and its impacts on spike protein biology, particularly sensitivity to neutralizing antibodies (nAbs), is critical as the pandemic continues.
パンデミックが続く中、重症急性呼吸器症候群コロナウイルス2(SARS-CoV-2)の進行中の進化と、スパイクタンパク質の生物学、特に中和抗体(nAbs)に対する感受性への影響を追跡することは非常に重要です。
The pandemic underwent a turning point in late summer 2023 with the emergence of BA.2.86, a variant characterized by over 30 spike protein mutations relative to then-dominant variant XBB.1.5.
パンデミックは、2023年夏の終わりに、当時優勢だった変異体XBB.1.5と比較して30を超えるスパイクタンパク質の変異を特徴とする変異体であるBA.2.86の出現により転換点を迎えました。
Fortunately, despite its myriad mutations, BA.2.86 did not exhibit increased immune evasion but was better neutralized by antibodies in convalescent and vaccinated sera relative to XBB-lineage variants.
幸いなことに、BA.2.86 は無数の変異にもかかわらず、免疫回避の増加は示さず、XBB 系統の変異体と比較して回復期血清およびワクチン接種血清中の抗体によってよりよく中和されました。
However, mounting concern has arisen with the subsequent variants that have evolved from BA.2.86.
しかし、BA.2.86 から進化したその後の変異体に対する懸念が高まっています。
This includes JN.1, which emerged in late 2023 and is characterized by the single spike mutation L455S relative to BA.2.86.
これには、2023 年後半に出現し、BA.2.86 と比較して単一スパイク変異 L455S を特徴とする JN.1 が含まれます。
This single mutation launched JN.1 to dominance worldwide from late 2023 through May 2024.
この単一の変異により、JN.1 は 2023 年後半から 2024 年 5 月にかけて世界中で優勢になりました。
L455S contributes to the lower affinity of JN.1 for human ACE2 but enhances its immune evasion to nAbs and viral transmission.
L455S は、JN.1 のヒト ACE2 に対する親和性を低下させる一方で、nAbs に対する免疫回避およびウイルス伝染を強化します。
Since JN.1’s emergence, a series of variants that possess mutations at key sites in spike have been identified, including L455, F456, and R346 (Figure 1A).
JN.1 の出現以来、L455、F456、R346 など、スパイクの主要部位に変異を持つ一連の変異体が特定されています (図 1A)。
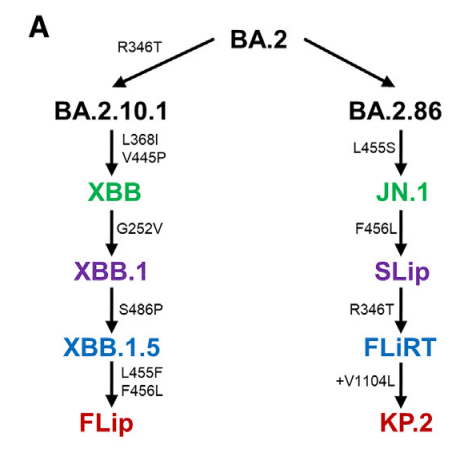
Initially, the so-called FLip variants emerged, possessing L455F and F456L mutations in the backbone of XBB.1.5, hence the name “FLip.”
当初は、XBB.1.5 のバックボーンに L455F および F456L 変異を持つ、いわゆる FLip 変異体が出現したため、「FLip」という名前が付けられました。
These sites have continued to be hotspots, with a strain called “SLip” having emerged, which has the JN.1 spike protein with the F456L mutation—the “S” referring to the L455S mutation that characterizes JN.1.
これらの部位は引き続きホットスポットであり、F456L 変異を伴う JN.1 スパイクタンパク質を持つ「SLip」と呼ばれる株が出現しました。「S」は、JN.1 の特徴である L455S 変異を指します。
More recently, we have seen the emergence of the FLiRT variant, which harbors an additional R346T mutation in the backbone of SLip.
最近では、SLip のバックボーンにさらに R346T 変異を持つ FLiRT 変異体の出現が見られました。
Another FLiRT variant, called KP.2, contains both R346T and F456L in S1, as well as a V1140L mutation in S2.
別の FLiRT 変異体である KP.2 は、S1 に R346T と F456L の両方を含み、S2 に V1140L 変異を含みます。
JN.1 is currently waning in dominance around the world, becoming quickly supplanted in circulation by KP.2 and other JN.1-derived variants (Figure 1B).
現在、JN.1 は世界中で優位性を失いつつあり、KP.2 やその他の JN.1 由来の変異体に急速に取って代わられています (図 1B)。
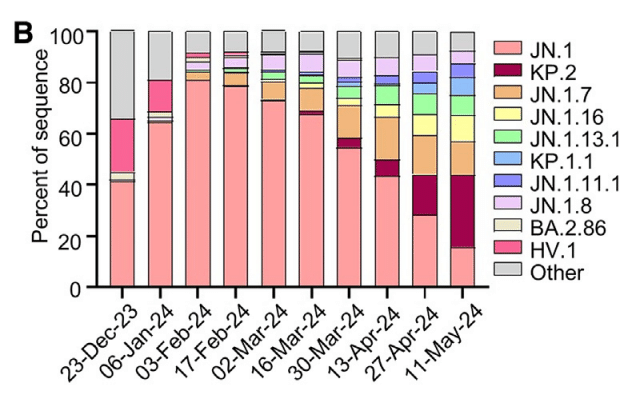
It has been shown that JN.1 can be neutralized by XBB.1.5 monovalent-vaccinated sera, albeit with reduced efficiency.
JN.1 は、効率は低下するものの、XBB.1.5 一価ワクチン接種血清によって中和できることが示されています。
However, it is currently unclear whether additional spike mutations gained in the JN.1-lineage subvariants will affect the efficacy of this COVID-19 vaccine formulation, especially with the approach of upcoming fall and winter seasons.
しかし、JN.1 系統の亜変異体で得られた追加のスパイク変異が、特に秋と冬の季節が近づいている中で、この COVID-19 ワクチン製剤の有効性に影響を与えるかどうかは、現時点では不明です。
In this study, we investigated the ability of SLip, FLiRT, and KP.2, in parallel with their parental JN.1, to be neutralized by sera from (1) individuals vaccinated with at least 2 doses of the monovalent ancestral spike (wild type [WT]) mRNA vaccine with 1 dose of the bivalent WT + BA.4/5 booster, (2) hamsters vaccinated with 2 doses of the XBB.1.5 monovalent recombinant mumps vaccine, and (3) individuals infected during the BA.2.86/JN.1 wave of infection in Columbus, OH (USA).
この研究では、SLip、FLiRT、KP.2 が、親 JN.1 と並行して、(1) 一価祖先スパイク (野生型 [WT]) mRNA ワクチンを少なくとも 2 回接種し、二価 WT + BA.4/5 ブースターを 1 回接種した個人、(2) 一価組換えムンプスワクチン XBB.1.5 を 2 回接種したハムスター、および (3) オハイオ州コロンバス (米国) での BA.2.86/JN.1 感染波中に感染した個人からの血清によって中和される能力を調査しました。
These analyses were conducted alongside the ancestral D614G and are supplemented with antigenic cartography analyses.
これらの分析は、祖先の D614G と並行して行われ、抗原地図作成分析によって補完されています。
We also characterized the entry and fusogenicity of these variants in 293T-ACE2 and CaLu-3 cells, as well as the spike processing and expression on plasma membranes.
また、293T-ACE2 および CaLu-3 細胞におけるこれらの変異体の侵入と融合性、およびスパイクの処理と細胞膜上での発現を特徴付けました。
Critically, our investigation focuses on the comparison between XBB.1.5 and JN.1 as immunogens against these JN.1-lineage variants and determines whether any dramatic changes in spike protein biology have occurred, as well as their impact on neutralization escape and viral infectivity.
重要なことに、私たちの調査は、これらの JN.1 系統の変異体に対する免疫原としての XBB.1.5 と JN.1 の比較に焦点を当てており、スパイクタンパク質の生物学的特性に劇的な変化が生じたかどうか、および中和回避とウイルス感染性への影響を判断しています。
Results
Impacts of JN.1-derived variants on viral entry and infectivity in 293T-ACE2 and CaLu-3 cells
JN.1由来変異体が293T-ACE2およびCaLu-3細胞へのウイルス侵入および感染性に与える影響
We first investigated the efficiency with which pseudotyped lentiviruses bearing SARS-CoV-2 spikes of interest can enter 293T cells overexpressing human ACE2 (293T-ACE2) and the human lung epithelial cell line CaLu-3.
まず、関心のある SARS-CoV-2 スパイクを持つ擬似型レンチウイルスが、ヒト ACE2 を過剰発現する 293T 細胞 (293T-ACE2) およびヒト肺上皮細胞株 CaLu-3 に侵入できる効率を調査しました。
As reported previously by our group, earlier Omicron variants, including BA.2 and XBB.1.5, exhibited higher infectivity relative to the ancestral D614G variant in 293T-ACE2 cells; however, infectivity decreased modestly for BA.2.86 and JN.1 (Figure 1C).
当グループが以前に報告したように、BA.2 および XBB.1.5 を含む初期の Omicron 変異体は、293T-ACE2 細胞において祖先 D614G 変異体と比較して高い感染性を示しました。ただし、BA.2.86 および JN.1 では感染性がわずかに低下しました (図 1C)。
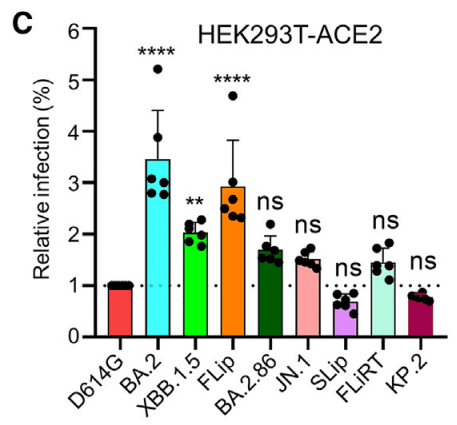
Here, we found that while FLiRT exhibited a similar infectivity to JN.1 in this cell line, KP.2 (p > 0.05), and especially SLip (p < 0.05), demonstrated modest reductions compared to JN.1 (Figure 1C).
ここで、FLiRT はこの細胞株で JN.1 と同様の感染性を示しましたが、KP.2 (p > 0.05)、特に SLip (p < 0.05) は JN.1 と比較してわずかに低下しました (図 1C)。
As we demonstrated previously, Omicron variants maintain a markedly reduced infectivity in CaLu-3 cells relative to D614G, with a notable increase for BA.2.86.
以前に示したように、Omicron 変異体は D614G と比較して CaLu-3 細胞で著しく低い感染性を維持しています。 BA.2.86 では顕著な増加が見られます。
However, we observed here that JN.1, SLip, FLiRT, and KP.2 exhibited decreased infectivity in CaLu-3 cells compared to BA.2.86 (p < 0.0001 for all), with SLip exhibiting the lowest infectivity of the group, a ∼2.2-fold decrease compared to JN.1 (p < 0.001) (Figure 1D).
しかし、ここでは JN.1、SLip、FLiRT、および KP.2 が BA.2.86 と比較して CaLu-3 細胞で低い感染性を示し (すべて p < 0.0001)、SLip はグループの中で最も低い感染性を示し、JN.1 と比較して約 2.2 倍減少しました (p < 0.001) (図 1D)。
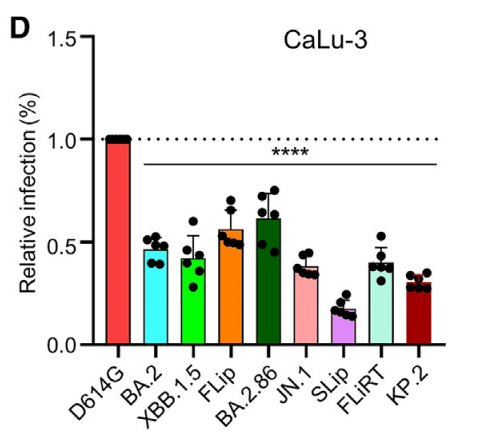
Overall, the recently emerged FLiRT and KP.2 subvariants, especially SLip, exhibit decreased infectivity in CaLu-3 cells compared to the earlier variants BA.2 and XBB.1.5, as well as their parental BA.2.86.
全体的に、最近出現した FLiRT および KP.2 サブ変異体、特に SLip は、以前の変異体 BA.2 および XBB.1.5、およびその親 BA.2.86 と比較して CaLu-3 細胞で低い感染性を示しています。
Increased resistance of SLip, FLiRT, and KP.2 to COVID-19 bivalent-vaccinated human sera by newer variants compared to parental JN.1
親株のJN.1と比較して、新しい変異体によるSLip、FLiRT、KP.2のCOVID-19二価ワクチン接種ヒト血清に対する耐性が増加
To test the extent of escape from neutralization by selected variants, we first used sera were from a cohort of healthcare workers (HCWs) at The Ohio State University Wexner Medical Center who had received at least 2 doses of the monovalent mRNA vaccine (WT) plus at least 1 dose of the bivalent vaccine containing both WT and BA.4/5 spikes (n = 10) (Figures 2A and 2B).
選択された変異体による中和からの回避の程度をテストするために、まず、オハイオ州立大学ウェクスナー医療センターの医療従事者(HCW)のコホートから採取した血清を使用しました。このコホートは、一価mRNAワクチン(WT)を少なくとも2回接種し、さらにWTとBA.4/5スパイクの両方を含む二価ワクチンを少なくとも1回接種しました(n = 10)(図2Aおよび2B)。
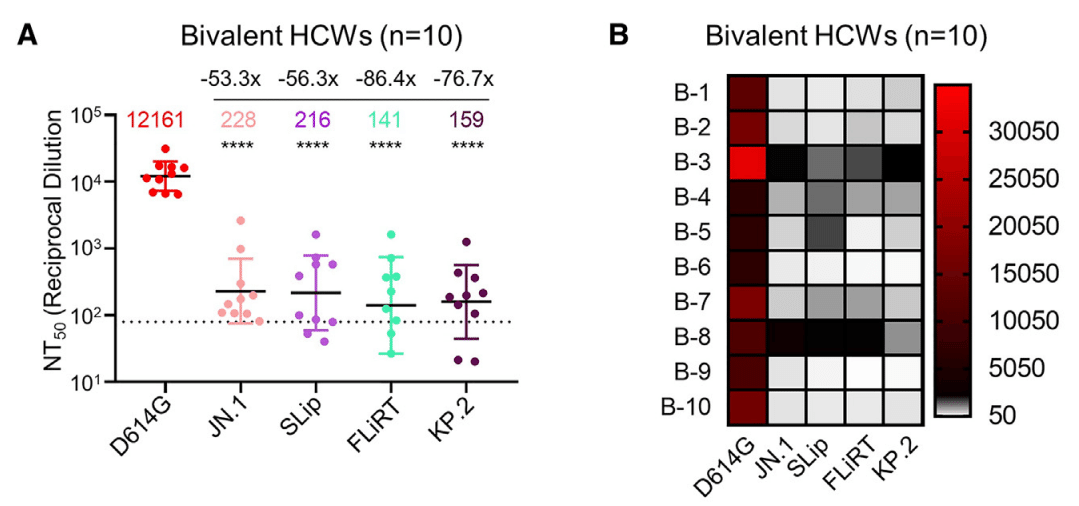
Neutralization was measured using pseudotyped lentiviruses mixed with serial dilutions of sera and infected 293T-ACE2 cells to determine neutralization titers at 50% (NT50) for each variant spike.
中和は、血清の連続希釈液と感染した 293T-ACE2 細胞を混合した擬似型レンチウイルスを使用して測定し、各変異スパイクの 50% での中和力価 (NT50) を決定しました。
As shown for all previous Omicron variants, JN.1 had markedly lower titers relative to the ancestral D614G, with a 53.3-fold decrease (p < 0.0001).
以前のすべての Omicron 変異体で示されているように、JN.1 は祖先の D614G と比較して力価が著しく低く、53.3 倍減少しました (p < 0.0001)。
SLip, FLiRT, and KP.2 also exhibited dramatically decreased titers, with NT50 56.3- (p < 0.0001), 86.4- (p < 0.0001), and 76.7-fold (p < 0.0001) lower than D614G, respectively.
SLip、FLiRT、および KP.2 も力価が大幅に低下し、NT50 はそれぞれ D614G より 56.3 倍 (p < 0.0001)、86.4 倍 (p < 0.0001)、および 76.7 倍 (p < 0.0001) 低くなりました。
Notably, whereas SLip showed a similar titer to JN.1, FLiRT and KP.2 had more dramatic decreases in titer, 1.62- (p > 0.05) and 1.43-fold (p > 0.05) lower than JN.1, respectively (Figures 2A and 2B).
注目すべきことに、SLip は JN.1 と同等の力価を示したのに対し、FLiRT と KP.2 は力価の減少がより顕著で、それぞれ JN.1 より 1.62 倍 (p > 0.05) と 1.43 倍 (p > 0.05) 低かった (図 2A と 2B)。
Overall, FLiRT and KP.2 exhibit increased escape from nAbs in bivalent-vaccinated sera compared to parental variant JN.1 and related variant SLip.
全体的に、FLiRT と KP.2 は、親変異体 JN.1 および関連変異体 SLip と比較して、二価ワクチン接種血清中の nAbs からの逃避が増加している。
Antibodies in XBB.1.5 monovalent-vaccinated hamster sera robustly neutralize FLiRT and KP.2, with reduced efficiency for SLip
XBB.1.5一価ワクチン接種ハムスター血清中の抗体はFLiRTとKP.2を強力に中和するが、SLipに対する効率は低下
We next measured neutralization using sera from golden Syrian hamsters vaccinated twice with the XBB.1.5 monovalent recombinant mumps vaccine (n = 10) (Figures 2C and 2D).
次に、XBB.1.5 単価組換えおたふく風邪ワクチンを 2 回接種したゴールデン シリアン ハムスターの血清を使用して中和を測定しました (n = 10) (図 2C および 2D)。
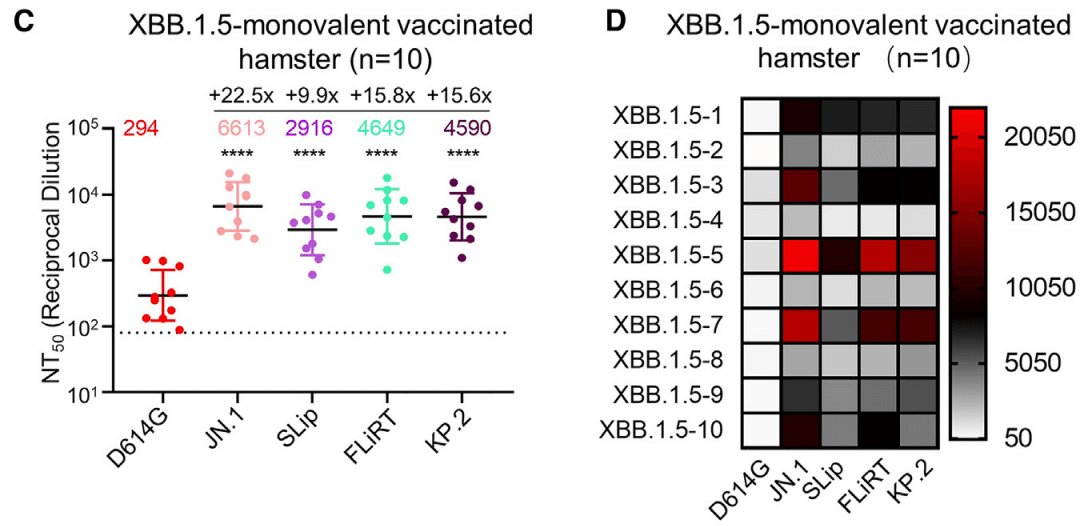
As demonstrated previously, the hamster sera had robust nAb titers against some of the latest Omicron subvariants, including BA.2.86, as compared to the ancestral D614G.
以前に示したように、ハムスターの血清は、BA.2.86 を含む最新の Omicron サブバリアントの一部に対して、先祖の D614G と比較して強力な nAb 力価を示しました。
Neutralization titers for JN.1 were also increased, with a calculated NT50 value of 6,613, which was 22.5-fold higher than D614G (p < 0.0001).
JN.1 の中和力価も増加し、計算された NT50 値は 6,613 で、D614G の 22.5 倍でした (p < 0.0001)。
The NT50 values for SLip, FLiRT, and KP.2 showed 9.9- (p < 0.0001), 15.8- (p < 0.0001), and 15.6-fold (p < 0.0001) increases compared to D614G, respectively, corresponding to 2.3-, 1.4-, and 1.4-fold decreases, respectively, relative to JN.1 (p > 0.05 for each) (Figures 2C and 2D).
SLip、FLiRT、KP.2 の NT50 値は、それぞれ D614G と比較して 9.9 倍 (p < 0.0001)、15.8 倍 (p < 0.0001)、15.6 倍 (p < 0.0001) 増加し、JN.1 と比較してそれぞれ 2.3 倍、1.4 倍、1.4 倍減少しました (それぞれ p > 0.05) (図 2C および 2D)。
The overall high titer of XBB.1.5 monovalent-vaccinated hamster sera against these new JN.1-derived variants was in sharp contrast to the generally low antibody titer exhibited by the bivalent-vaccinated group (Figures 2A–2D), but the downward trends of each variant were similar except for SLip.
これらの新しい JN.1 由来の変異体に対する XBB.1.5 一価ワクチン接種ハムスター血清の全体的な高い力価は、二価ワクチン接種グループが示す一般に低い抗体力価とは対照的でしたが (図 2A~2D)、各変異体の下降傾向は SLip を除いて同様でした。
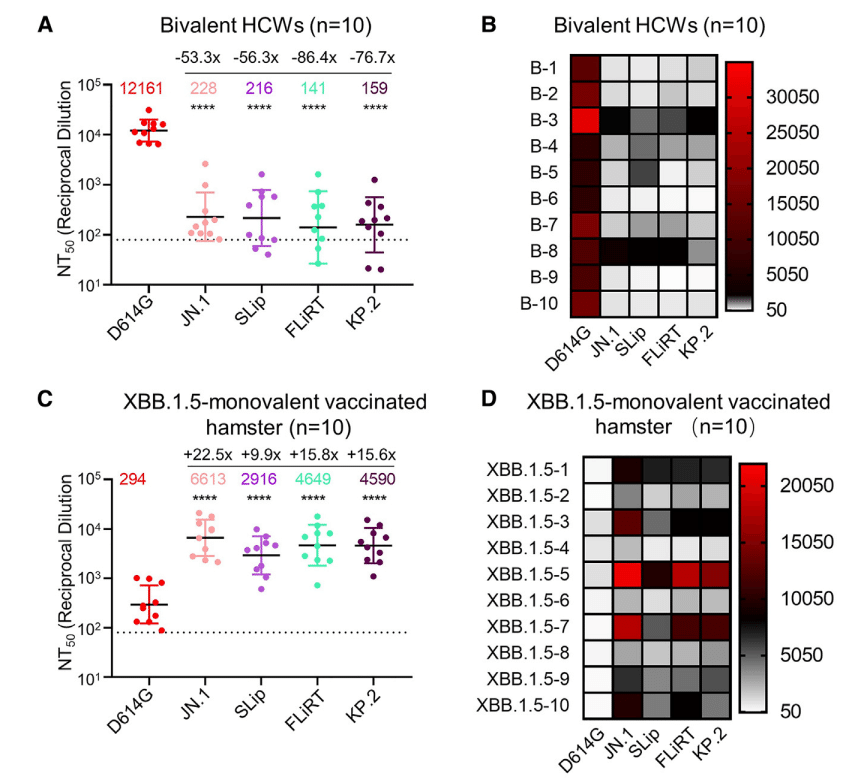
The relatively strong neutralization escape of SLip from the XBB.1.5 monovalent vaccine, as compared to FLiRT and KP.2, was likely due to a specific amino acid R346T change in the receptor-binding domain (RBD) of XBB.1.5, FLiRT, and KP.2 spikes but not in the SLip spike (see discussion).
FLiRT および KP.2 と比較して、XBB.1.5 単価ワクチンからの SLip の中和回避が比較的強力だったのは、XBB.1.5、FLiRT、および KP.2 スパイクの受容体結合ドメイン (RBD) における特定のアミノ酸 R346T の変化によるものと考えられます。 ただし、SLip スパイクではそうではありません (説明を参照)。
Nonetheless, sera from XBB.1.5-vaccinated hamsters can effectively neutralize JN.1 and JN.1-derived subvariants.
それにもかかわらず、XBB.1.5 ワクチン接種を受けたハムスターの血清は、JN.1 および JN.1 由来の亜変異体を効果的に中和できます。
SLip, KP.2, and especially FLiRT exhibit decreased sensitivity to neutralization by BA.2.86/JN.1-wave convalescent sera compared to parental JN.1
SLip、KP.2、特にFLiRTは、親JN.1と比較して、BA.2.86/JN.1波回復期血清による中和に対する感受性が低下
To examine variant neutralization by antibodies induced during a natural infection, we employed sera from individuals who tested positive for COVID during the BA.2.86/JN.1-wave of infection between November 2023 and February 2024 in Columbus, OH (USA; n = 7) (Figures 2E and 2F).
自然感染中に誘導される抗体による変異体中和を調べるために、オハイオ州コロンバス(米国)で2023年11月から2024年2月までのBA.2.86/JN.1感染波中にCOVID陽性となった個人の血清を使用しました(n = 7)(図2Eおよび2F)。
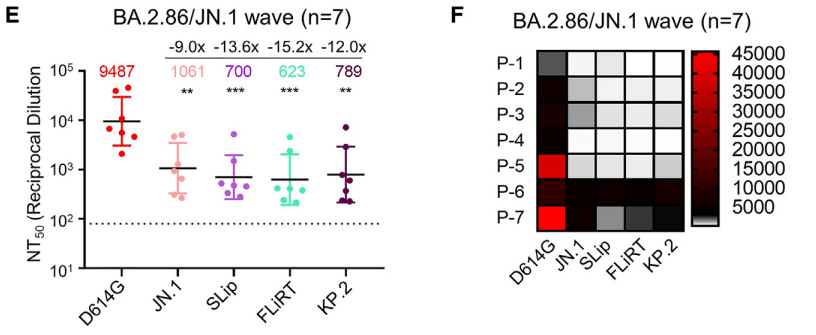
Four were Columbus first responders and their household contacts (n = 4, P1–P4) and three were intensive care unit (ICU) COVID-19 patients admitted to the OSU Medical Center (n = 3, P5–P7).
4人はコロンバスの救急隊員とその家庭内接触者(n = 4、P1~P4)で、3人はOSUメディカルセンターに入院した集中治療室(ICU)のCOVID-19患者(n = 3、P5~P7)でした。
All patients had received different doses of mRNA vaccine, with samples being collected between 34 and 892 days following the last vaccination (Table S1).
すべての患者は異なる用量のmRNAワクチンを接種しており、サンプルは最後のワクチン接種から34~892日後に採取されました(表S1)。
As we have shown previously, neutralization titers were detectable, albeit modest, especially for P1–P4 against JN.1, with about 9-fold reduction relative to D614G (p < 0.01).
以前に示したように、中和力価は、特に JN.1 に対する P1~P4 では、D614G と比較して約 9 分の 1 に減少し、控えめではあるものの検出可能でした (p < 0.01)。
Titers against SLip, FLiRT, and KP.2 were further decreased, with reductions of 13.6- (p < 0.001), 15.2- (p < 0.001), and 12-fold (p < 0.01) relative to D614G, respectively.
SLip、FLiRT、KP.2 に対する力価はさらに減少し、それぞれ D614G と比較して 13.6 分の 1 (p < 0.001)、15.2 分の 1 (p < 0.001)、12 分の 1 (p < 0.01) に減少しました。
Similar to the bivalent cohort, the FLiRT variant exhibited the biggest drop in titers compared to JN.1 (1.70-fold decrease), though the difference was not statistically significant (p > 0.05) (Figures 2E and 2F).
二価コホートと同様に、FLiRT 変異体は JN.1 と比較して力価が最も低下しました (1.70 分の 1 の減少) が、その差は統計的に有意ではありませんでした (p > 0.05) (図 2E および 2F)。
Samples P5, P6, and P7 were collected from individuals admitted to the ICU at the Ohio State University Wexner Medical Center (Figure 2F; Table S1).
サンプル P5、P6、P7 は、オハイオ州立大学ウェクスナー医療センターの ICU に入院した患者から採取されました (図 2F、表 S1)。
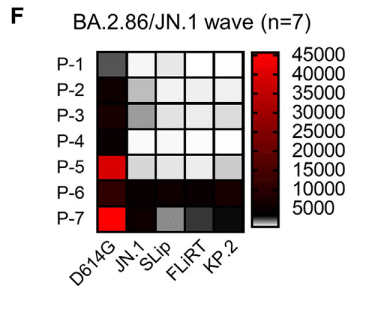
Notably, two of these patients, P6 and P7, exhibited higher titers against the JN.1-lineage variants.
注目すべきことに、これらの患者のうち 2 人、P6 と P7 は、JN.1 系統の変異体に対して高い力価を示しました。
P6 is a 77-year-old male who received one dose of the Moderna monovalent vaccine and one dose of the Pfizer bivalent vaccine, with the sample taken 434 days after his last vaccination.
P6 は 77 歳の男性で、モデルナ社の単価ワクチンを 1 回、ファイザー社の二価ワクチンを 1 回接種し、最後のワクチン接種から 434 日後にサンプルを採取しました。
P7 is a 46-year-old female who received three doses of the Moderna monovalent vaccine and one dose of the Moderna bivalent vaccine, with her sample collected 334 days after her last vaccination.P7 は 46 歳の女性で、モデルナ社の一価ワクチンを 3 回、モデルナ社の二価ワクチンを 1 回接種し、最後のワクチン接種から 334 日後にサンプルを採取しました。
P5 is a 49-year-old male ICU patient and had only received two doses of the Moderna monovalent vaccine; his sample was taken 892 days after his last vaccination, with his nAb titers against JN.1, SLip, FLiRT, and KP.2 being the lowest among the three ICU patients.
P5 は 49 歳の男性 ICU 患者で、モデルナ社の一価ワクチンを 2 回しか接種していません;サンプルは最後のワクチン接種から 892 日後に採取され、JN.1、SLip、FLiRT、KP.2 に対する nAb 力価は 3 人の ICU 患者の中で最も低いものでした。
Overall, sera of BA.2.86/JN.1 convalescent individuals effectively neutralized the latest JN.1-lineage subvariants SLip, FLiRT, and KP.2 but with somewhat reduced efficiency for FLiRT.
全体的に、BA.2.86/JN.1 回復期患者の血清は、最新の JN.1 系統の亜種 SLip、FLiRT、KP.2 を効果的に中和しましたが、FLiRT に対する効率はやや低下しました。
Class III mAb S309 does not neutralize SLip or FLiRT
クラスIII mAb S309はSLipまたはFLiRTを中和しない
Another critical strategy for pandemic control measures is the use of therapeutic monoclonal antibodies (mAbs), which was demonstrated during the COVID-19 pandemic, especially between 2020 and 2021.
パンデミック制御対策のもう1つの重要な戦略は、治療用モノクローナル抗体(mAb)の使用であり、これはCOVID-19パンデミック、特に2020年から2021年の間に実証されました。
However, because of their binding being limited to a single epitope on the SARS-CoV-2 spike, single mutations can easily disrupt their efficacy, making most of the developed mAbs completely ineffective for Omicron.
ただし、結合がSARS-CoV-2スパイク上の単一のエピトープに限定されているため、単一の変異によってその有効性が簡単に損なわれ、開発されたmAbのほとんどがオミクロンに対して完全に無効になります。
Notably, we and others have previously shown that the binding of class III mAb S309 is largely maintained against Omicron subvariants, apart from BA.2.75.2, CA.3, CH.1.1, BA.2.86, and JN.1.
特に、私たちと他の研究者は以前、クラスIII mAb S309の結合が、BA.2.75.2、CA.3、CH.1.1、BA.2.86、およびJN.1を除くオミクロンのサブバリアントに対してほぼ維持されることを示しました。
This trend appeared to continue, as both SLip and FLiRT exhibited a complete escape of neutralization by S309 (Figures 3A and 3B).
SLipとFLiRTの両方がS309による中和を完全に回避したことから、この傾向は続いているようです(図3Aおよび3B)。
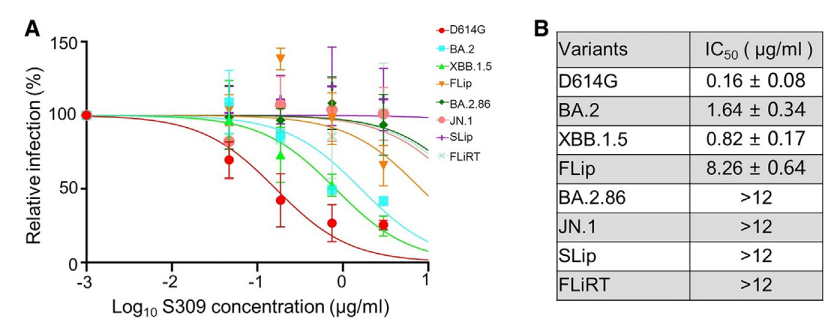
We did not perform this experiment for KP.2, which harbors the conserved D339H mutation critical for S309 resistance (see discussion).
S309 耐性にとって重要な保存された D339H 変異を保持する KP.2 については、この実験は実行しませんでした (説明を参照)。
Antigenic cartography analysis demonstrates decreased antigenic distances of SLip, FLiRT, and KP.2 in XBB.1.5 monovalent-vaccinated and BA.2.86/JN.1-infected groups
抗原地図作成分析により、XBB.1.5一価ワクチン接種群とBA.2.86/JN.1感染群におけるSLip、FLiRT、KP.2の抗原距離の減少が示される
To further elucidate the relationships between these variants, we conducted antigenic cartography analysis (Figure 4).
これらの変異体間の関係をさらに解明するために、抗原地図作成分析を実施しました (図 4)。
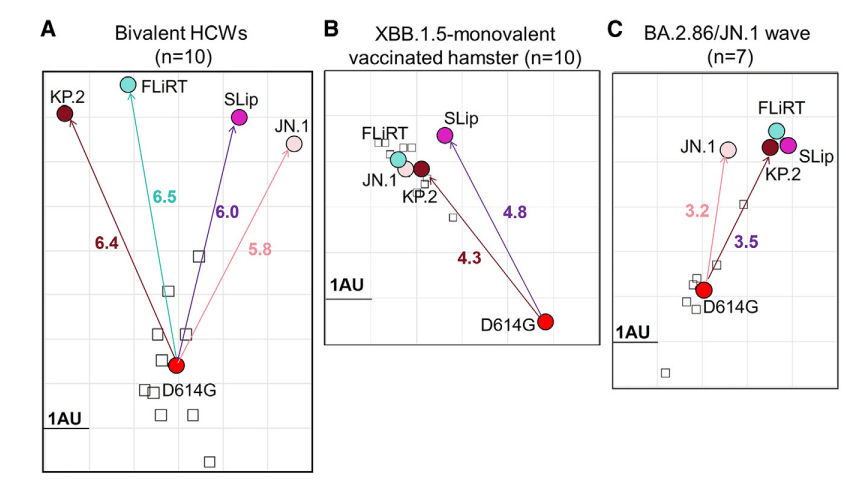
Briefly, this analysis transforms neutralization titers based on relative differences between titers for each variant (circles) and each serum sample (squares) displayed as antigenic units (AUs).
簡単に言うと、この分析では、抗原単位 (AU) として表示される各変異体 (円) と各血清サンプル (四角) の力価の相対的な差に基づいて中和力価を変換します。
As would be expected, JN.1 subvariants are antigenically distinct from the ancestral D614G in the bivalent cohort, with FLiRT being the most distinctive compared to SLip, KP.2, and their parental JN.1 (Figure 4A).
予想どおり、JN.1 サブ変異体は二価コホートの祖先 D614G とは抗原的に異なり、SLip、KP.2、およびそれらの親 JN.1 と比較して FLiRT が最も特徴的です (図 4A)。
In the XBB.1.5 monovalent-vaccinated hamster group, distances between D614G and the JN.1 variants are markedly reduced, from ∼6 AUs in the bivalent cohort down to ∼4–5 AUs.
XBB.1.5 一価ワクチン接種ハムスター グループでは、D614G と JN.1 変異体間の距離が二価コホートの約 6 AU から約 4~5 AU に大幅に短縮されています。
The variants are also clustered more closely to each other, with SLip being slightly further away from FLiRT and KP.2 (Figure 4B).
変異体同士もより密集しており、SLip は FLiRT および KP.2 からわずかに離れています (図 4B)。
A similar phenomenon was observed in the BA.2.86/JN.1-wave cohort, with overall shorter antigenic distances (∼3–4 AUs) compared to the bivalent and XBB.1.5 monovalent cohorts.
BA.2.86/JN.1 波コホートでも同様の現象が見られ、二価および XBB.1.5 一価コホートと比較して、全体的な抗原距離が短くなっています (約 3~4 AU)。
Again, JN.1-derived subvariants largely cluster together, with parental JN.1 being relatively distant from SLip, FLiRT, and KP.2 (Figure 4C).
また、JN.1 由来のサブ変異体は大部分が密集しており、親 JN.1 は SLip、FLiRT、および KP.2 から比較的離れています (図 4C)。
SLip, FLiRT, and KP.2 spikes exhibit modestly decreased fusogenicity, surface expression, and processing relative to JN.1
SLip、FLiRT、KP.2スパイクは、JN.1と比較して、融合性、表面発現、および処理がわずかに低下
Our previous studies revealed notable changes in the spike biology of many Omicron variants, including their membrane fusogenicity and processing.
これまでの研究では、多くの Omicron バリアントのスパイク生物学に、膜融合性や処理など、顕著な変化がみられました。
Here, we characterized the ability of spikes from new JN.1-derived subvariants to trigger fusion between cell membranes (Figures 5A–5D ), their expression on cell plasma membranes (Figures 5E and 5F), and their processing into S1/S2 subunits by furin in virus producer cells (Figure 5G).
ここでは、新しい JN.1 由来のサブバリアントのスパイクが細胞膜間の融合を引き起こす能力 (図 5A~5D)、細胞膜上での発現 (図 5E および 5F)、およびウイルス産生細胞におけるフリンによる S1/S2 サブユニットへの処理 (図 5G) を特徴付けました。
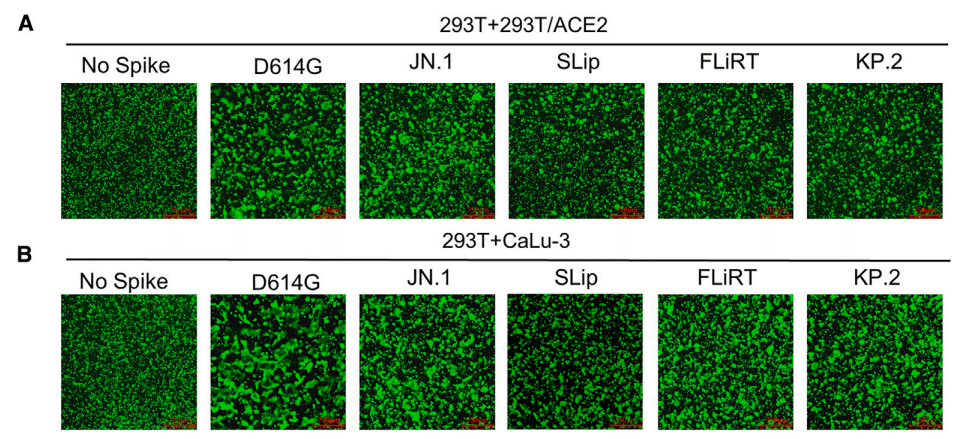
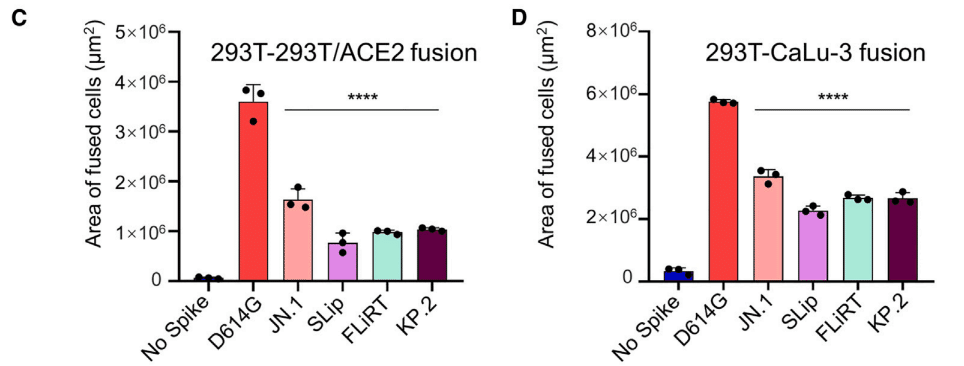
Similar to other Omicron variants, JN.1 exhibited markedly reduced cell-cell fusion activity relative to D614G.
他の Omicron バリアントと同様に、JN.1 は D614G と比較して細胞間融合活性が著しく低下しました。
This downtrend was maintained for FLiRT and KP.2, and more so for SLip, in both 293T-ACE2 and CaLu-3 cells (p < 0.0001 compared to D614G).
この下降傾向は、293T-ACE2 細胞と CaLu-3 細胞の両方で FLiRT と KP.2 で維持され、SLip ではさらに維持されました (D614G と比較して p < 0.0001)。
The level of cell-cell fusion activity for these three new subvariants appeared to be lower than parental JN.1 (p < 0.01).
これら 3 つの新しいサブバリアントの細胞間融合活性のレベルは、親 JN.1 よりも低いようでした (p < 0.01)。
The expression of spikes on the surface of 293T cells used to produce pseudotyped vectors was determined.
擬似型ベクターを生成するために使用された 293T 細胞の表面上のスパイクの発現が測定されました。
We found that JN.1-derived subvariants exhibited a 2- to 3-fold decrease in expression compared to ancestral D614G (p < 0.0001), with FLiRT being significantly lower than the parental JN.1 (p < 0.0001) (Figures 5E and 5F).
JN.1由来の亜変異体は、祖先のD614Gと比較して発現が2~3倍減少していることが分かりました(p < 0.0001)。また、FLiRTは親のJN.1よりも大幅に低いことがわかりました(p < 0.0001)(図5Eおよび5F)。
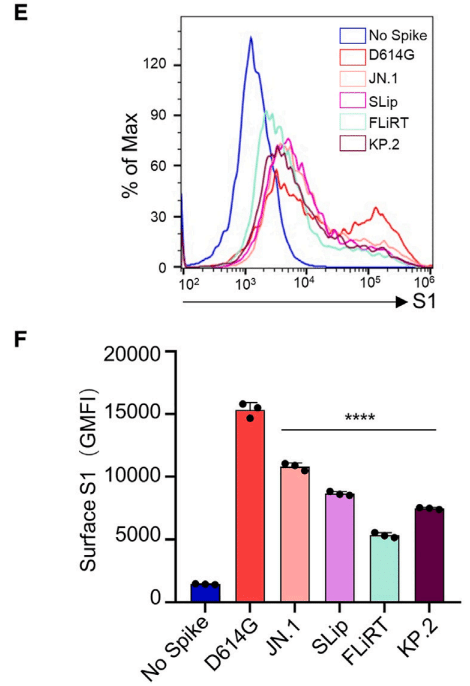
We probed lysates of the transfected 293T cells for S2 subunits of spike to determine the extent of furin cleavage efficiency by quantifying the ratio of S2/S, and we observed that the processing efficiency for SLip, FLiRT, and KP.2 spikes was modestly decreased compared to JN.1 (Figure 5G).
トランスフェクトされた293T細胞の溶解液でスパイクのS2サブユニットを調べ、S2/S比を定量化してフーリン切断効率の程度を決定したところ、SLip、FLiRT、およびKP.2スパイクの処理効率はJN.1と比較してわずかに低下していることがわかりました(図5G)。
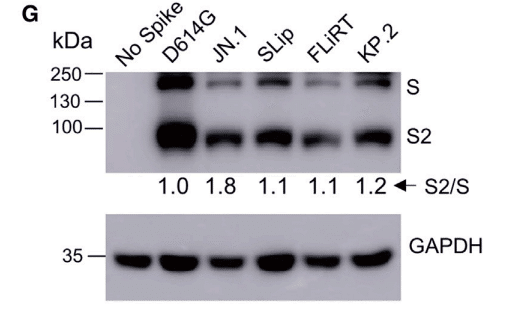
Overall, the decreases in cell-cell fusion and spike processing are consistent with their attenuated infectivity in 293T-ACE2 and CaLu-3 cells (Figures 1C and 1D).
全体として、細胞間融合およびスパイク処理の減少は、293T-ACE2およびCaLu-3細胞における感染性の減衰と一致しています(図1Cおよび1D)。
Structural modeling of mutations in SLip, FLiRT, and KP.2 spikes
SLip、FLiRT、KP.2スパイクの変異の構造モデリング
To better understand the impact of spike mutations on these new variants, we performed homology modeling to investigate alterations in receptor engagement, spike conformational stability, and antibody interactions.
スパイク変異がこれらの新しい変異体に与える影響をより深く理解するために、受容体のエンゲージメント、スパイク構造の安定性、および抗体の相互作用の変化を調べるために相同性モデリングを実行しました。
Residue R346 in the receptor-binding motif (RBM) can form both a hydrogen bond and a salt bridge with residue D450, which is present in the parental BA.2.86 lineage (N450D).
受容体結合モチーフ (RBM) の残基 R346 は、親 BA.2.86 系統 (N450D) に存在する残基 D450 と水素結合と塩橋の両方を形成できます。
This interaction pulls residue D450 and its loop away from the RBM and potentially disturbs the process of receptor engagement.
この相互作用により、残基 D450 とそのループが RBM から引き離され、受容体との結合プロセスが妨げられる可能性があります。
The R346T mutation abolishes this interaction, releasing tension on residue D450 and the RBM, thus potentially enhancing ACE2 binding affinity (Figure 6A).
R346T 変異はこの相互作用を無効にし、残基 D450 と RBM の張力を解放して、ACE2 結合親和性を高める可能性があります (図 6A)。
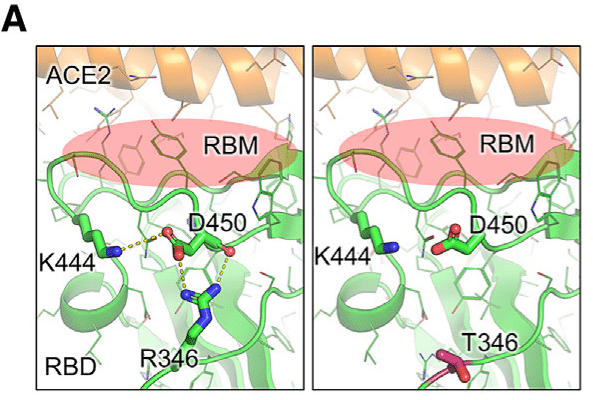
Conversely, residues L455 and F456, which are centrally located within the RBM, are encased in a hydrophobic cage formed by Y421, Y453, Y473, and Y489.
逆に、RBM 内の中心に位置する残基 L455 と F456 は、Y421、Y453、Y473、および Y489 によって形成される疎水性ケージに包まれています。
This hydrophobic core is crucial for ACE2 binding.
この疎水性コアは、ACE2 の結合に不可欠です。
Mutations such as F456L and L455S found in strains JN.1 and SLip can reduce the local hydrophobicity of the RBM, diminishing interactions with ACE2 residues T27, K31, D30, and H34 and thus potentially decreasing viral affinity for ACE2 (Figure 6B).
JN.1 株および SLip 株に見られる F456L や L455S などの変異は、RBM の局所的疎水性を低下させ、ACE2 残基 T27、K31、D30、および H34 との相互作用を減少させ、ACE2 に対するウイルス親和性を低下させる可能性があります (図 6B)。
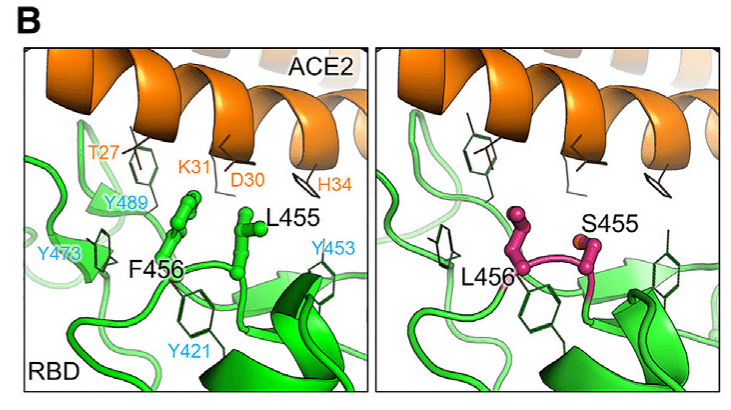
Additionally, structural analysis indicates that residue V1104 is situated in a hydrophobic core, together with P1090, F1095, and I1115, on the spike stem region.
さらに、構造解析により、残基 V1104 は、スパイク ステム領域上の P1090、F1095、および I1115 とともに疎水性コアに位置していることが示されています。
The V1104L mutation fills a cavity and improves the local hydrophobic interaction, potentially stabilizing the prefusion spike conformation; this would reduce the efficiency of spike protein transition to a postfusion conformation (Figure 6C).
V1104L 変異は空洞を埋めて局所的な疎水性相互作用を改善し、融合前のスパイク構造を安定化させる可能性があります。これにより、融合後の構造へのスパイクタンパク質の遷移効率が低下します (図 6C)。
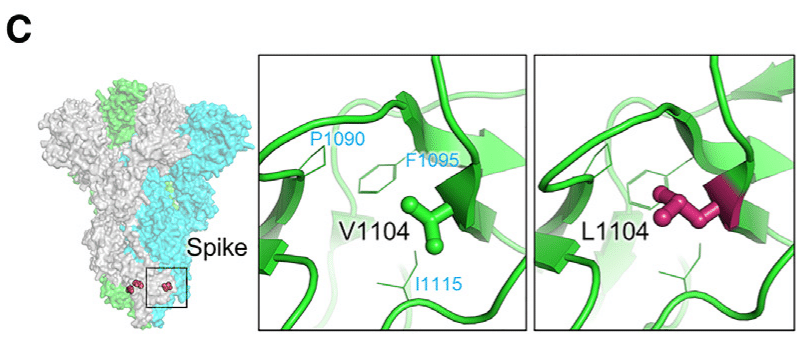
Residues D339 and R346 lie within the epitope region of class III antibodies, including S309 (Figure 6D).
残基 D339 と R346 は、S309 を含むクラス III 抗体のエピトープ領域内にあります (図 6D)。
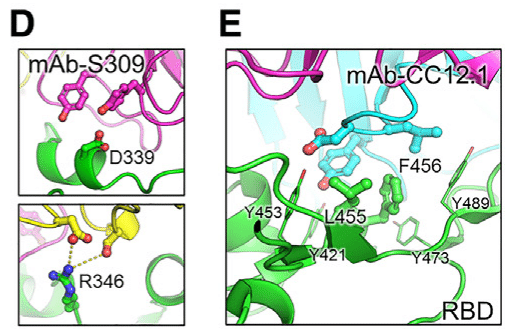
Mutations at these positions are present in the BA.2.86 lineage and subsequent lineages FLiRT and KP.2, which could enhance viral evasion from antibody neutralization.
これらの位置の変異は、BA.2.86 系統とそれに続く系統の FLiRT および KP.2 に存在し、抗体中和からのウイルスの回避を強化する可能性があります。
Lastly, residues F456 and L455 on the RBM are frequently targeted by class I RBD nAbs, such as CC12.1 (Figure 6E), and the L455S and F456L mutations, which involve changes in size and chemical properties, effectively enabled viral evasion from S309 and CC12.1.
最後に、RBM 上の残基 F456 と L455 は、CC12.1 などのクラス I RBD nAbs によって頻繁に標的にされ (図 6E)、サイズと化学的特性の変化を伴う L455S および F456L 変異は、S309 および CC12.1 からのウイルスの回避を効果的に可能にしました。
Discussion
The ongoing evolution of the SARS-CoV-2 spike protein continues to pose significant challenges to controlling the COVID-19 pandemic.
SARS-CoV-2 スパイクタンパク質の進化は、COVID-19 パンデミックの制御に大きな課題をもたらし続けています。
Though BA.2.86 did not prove to have increased evasion of nAbs in vaccinated and convalescent sera relative to prior variants, subsequent variants, namely JN.1, have shown mounting escape.
BA.2.86 は、以前の変異体と比較してワクチン接種を受けた血清および回復期血清における nAbs の回避が増加したとは証明されていませんが、その後の変異体、つまり JN.1 は回避が増加しています。
Recent SARS-CoV-2 Omicron variants have been accumulating convergent mutations in the spike, particularly at key sites including L455, F456, and R346.
最近の SARS-CoV-2 オミクロン変異体は、特に L455、F456、R346 などの重要な部位でスパイクに収束変異を蓄積しています。
These sites have critical roles in immunogenicity, receptor binding, and overall viral fitness.
これらの部位は、免疫原性、受容体結合、および全体的なウイルス適応において重要な役割を果たします。
Interestingly, despite its detrimental impact on ACE2 binding, the single L455S mutation that differentiates JN.1 from BA.2.86 led to markedly more immune evasion, sending the variant to dominance worldwide.
興味深いことに、ACE2 結合に悪影響を与えるにもかかわらず、JN.1 と BA.2.86 を区別する単一の L455S 変異は、免疫回避を著しく高め、この変異体が世界中で優勢になりました。
Here, we show that F456L (SLip) and R346T (FLiRT) contribute to further escape of JN.1-derived variants from nAbs in all cohorts tested relative to their parental JN.1 (Figures 2A–2F).
ここでは、F456L (SLip) と R346T (FLiRT) が、テストしたすべてのコホートにおいて、親 JN.1 と比較して、JN.1 由来の変異体が nAbs からさらに逃れるのに寄与していることを示しています (図 2A~2F)。
FLiRT displayed the greatest decreases of nAbs titers in bivalent-immunized HCWs and BA.2.86/JN.1-wave patients, likely due to the fact that the immunogens for both cohorts, i.e., WT, BA.4/5, or BA.2.86/JN.1, lack the R346T mutation (Figures 2A, 2B, 2E, and 2F).
FLiRT は、二価免疫を受けた医療従事者と BA.2.86/JN.1 波の患者で nAbs 力価の最大の減少を示しましたが、これはおそらく、両コホートの免疫原、つまり WT、BA.4/5、または BA.2.86/JN.1 に R346T 変異が欠けていることによるものと考えられます (図 2A、2B、2E、および 2F)。
This notion is further supported by our finding that both FLiRT and KP.2 are neutralized better than SLip by the XBB.1.5 monovalent hamster serum samples and that the XBB.1.5 spike immunogen harbors the critical R346T mutation, which is present in both FLiRT and KP.2 but not SLip (Figures 2C and 2D).
この考えは、XBB.1.5 単価ハムスター血清サンプルによって FLiRT と KP.2 の両方が SLip よりもよく中和され、XBB.1.5 スパイク免疫原が重要な R346T 変異を抱えているという発見によってさらに裏付けられています。この変異は FLiRT と KP.2 の両方に存在し、SLip には存在しません (図 2C および 2D)。
We and other groups have previously demonstrated that R346T is a critical site for mAb binding and immune evasion.
私たちと他のグループは以前、R346T が mAb 結合と免疫回避の重要な部位であることを実証しました。
Another advantage of the R346T mutation is compensating for the loss of affinity for ACE2 caused by the L455S and F456L mutations (Figure 6B).
R346T 変異のもう 1 つの利点は、L455S および F456L 変異によって引き起こされる ACE2 に対する親和性の喪失を補うことです (図 6B)。
Both the L455S and F456L mutations reduce hydrophobic contacts in the RBD, leading to less binding for ACE2. R346T helps compensate for this reduction by strengthening conformational support in the RBM (Figure 6A).
L455S および F456L 変異はいずれも RBD の疎水性接触を減少させ、ACE2 の結合を低下させます。R346T は RBM の立体構造サポートを強化することでこの減少を補います (図 6A)。
The consequences of these effects can be seen in the increased viral infectivity of FLiRT and KP.2 relative to SLip (Figures 1C and 1D).
これらの効果の結果は、SLip と比較して FLiRT および KP.2 のウイルス感染性が増加していることに見られます (図 1C および 1D)。
In particular, the KP.2 variant benefits from the effects of V1104L, which serves to further stabilize the spike conformation through hydrophobic internal cavity filling (Figure 6C); this likely results in a less efficient transition from prefusion and postfusion spikes, therefore impeding infectivity.
特に、KP.2 変異体は V1104L の効果の恩恵を受けており、疎水性内部空洞充填によってスパイク立体構造をさらに安定化させます (図 6C);これにより、融合前スパイクと融合後スパイクの移行が効率が悪くなり、感染性が阻害される可能性があります。
We found that FLiRT and SLip were both resistant to neutralization by class III mAb S309 like their parental variants BA.2.86 and JN.1.
FLiRT と SLip はどちらも、親の変異体 BA.2.86 および JN.1 と同様に、クラス III mAb S309 による中和に耐性があることが分かりました。
Accordingly, all of these variants, including KP.2, possess the D339H mutation, which is situated directly in the center of the class III antibody epitope, creating a steric hindrance that abolishes S309 binding (Figure 6D).
したがって、KP.2 を含むこれらの変異体はすべて、クラス III 抗体エピトープの中央に位置する D339H 変異を有しており、S309 結合を無効にする立体障害を生み出しています (図 6D)。
The SLip variant displayed the lowest nAb titers in the XBB.1.5 monovalent hamster cohort, likely due to the presence of the F456L mutation on top of its parental JN.1 containing L455S.
SLip 変異体は、XBB.1.5 単価ハムスターコホートで最も低い nAb 力価を示しましたが、これはおそらく、L455S を含む親 JN.1 の上に F456L 変異が存在するためでしょう。
The F456L mutation has occurred in several previous circulating variants, including FLip, thus contributing to their strong immune evasion.
F456L 変異は、FLip を含むいくつかの以前の循環変異体で発生しており、強力な免疫回避に寄与しています。
It is of note that this mutation is increasing in frequency among circulating variants.
この変異は、循環変異体の間で頻度が増加していることは注目に値します。
F456L mediates immune evasion by altering key epitopes targeted by class I mAbs (Figure 6E), although other mutations (e.g., L455S) also contribute to this disruption of the class I epitopes.
F456L はクラス I mAbs が標的とする主要なエピトープを変化させることで免疫回避を媒介しますが (図 6E)、他の変異 (L455S など) もクラス I エピトープの破壊に寄与します。
Notably, F456L is not present in the XBB.1.5 spike immunogen, and this could explain why SLip had the lowest nAb titers in the XBB.1.5 monovalent hamster cohort.
注目すべきことに、F456L は XBB.1.5 スパイク免疫原には存在せず、これが XBB.1.5 単価ハムスターコホートで SLip の nAb 力価が最も低かった理由を説明できます。
However, titers of XBB.1.5 monovalent hamster sera were still well above the limit of detection and only displayed a modest drop from JN.1, apart from SLip, suggesting that the XBB.1.5 spike as an immunogen can still provide potentially effective protection against JN.1-lineage variants.
ただし、XBB.1.5 単価ハムスター血清の力価は依然として検出限界をはるかに上回っており、SLip を除いて JN.1 からわずかに低下しただけであったことから、免疫原としての XBB.1.5 スパイクは依然として JN.1 系統の変異体に対して潜在的に効果的な保護を提供できることが示唆されます。
In addition, XBB.1.5 monovalent hamster sera exhibited less distance between the JN.1-lineage spikes, again suggesting that the XBB.1.5 spike as an immunogen could stimulate a broader antibody response than the WT + BA.4/5 bivalent vaccine (Figures 4A and 4B).
さらに、XBB.1.5 単価ハムスター血清では、JN.1 系統のスパイク間の距離が短く、これもまた、免疫原としての XBB.1.5 スパイクが WT + BA.4/5 二価ワクチンよりも幅広い抗体反応を刺激できることを示唆しています (図 4A および 4B)。
The BA.2.86/JN.1-wave cohort had similar antigenic mapping to the XBB.1.5 cohort, with even shorter distances between D614G and the JN.1 subvariants—and the JN.1 variants themselves were also closely clustered (Figure 4C).
BA.2.86/JN.1 波コホートは、XBB.1.5 コホートと同様の抗原マッピングを示し、D614G と JN.1 サブバリアント間の距離はさらに短く、JN.1 バリアント自体も密集していました (図 4C)。
This pattern of response is similar to data presented by other groups, which showed that JN.1 infection, together with prior immunization, stimulates superior nAb titers against JN.1 variants compared to BA.5, XBB, or XBB.1.5 breakthrough infections.
この反応パターンは、他のグループによって提示されたデータと似ており、JN.1 感染と事前の免疫化を組み合わせると、BA.5、XBB、または XBB.1.5 のブレイクスルー感染と比較して、JN.1 バリアントに対する優れた nAb 力価が刺激されることが示されています。
The shorter antigenic distance for our cohort, as well as the results from other groups, suggest that the JN.1 spike can and probably should serve as a more effective immunogen to stimulate nAbs against JN.1-lineage variants.
他のグループの結果と同様に、私たちのコホートの抗原距離が短いことから、JN.1 スパイクは JN.1 系統の変異体に対する nAbs を刺激するより効果的な免疫原として機能でき、またおそらくそうすべきであることが示唆されます。
Overall, the difference between XBB.1.5 and BA.2.86/JN.1 spikes as immunogens against JN.1 variants (except SLip) appears negligible (Figures 2C–2F, 4B, and 4C).
全体的に、JN.1 変異体 (SLip を除く) に対する免疫原としての XBB.1.5 と BA.2.86/JN.1 スパイクの違いはごくわずかであるように見えます (図 2C ~ 2F、4B、および 4C)。
We did not find evidence of further enhanced nAb escape for the KP.2 spike in three sets of sera relative to FLiRT and SLip (Figures 2E and 2F) that could explain its increasing dominance in circulation.
3 セットの血清で、KP.2 スパイクの nAb エスケープが FLiRT および SLip と比較してさらに強化されたという証拠は見つかりませんでした (図 2E および 2F)。 これは循環における KP.2 の優位性の増加を説明できるものです。
However, KP.2 acquired additional mutations in other regions of the SARS-CoV-2 genome, including a T2283I mutation in non-structural protein 3 outside the papain-like protease domain, which likely facilitates viral replication and/or modulates the host immune response.
ただし、KP.2 は SARS-CoV-2 ゲノムの他の領域で追加の変異を獲得しました。これにはパパイン様プロテアーゼ ドメイン外の非構造タンパク質 3 の T2283I 変異が含まれます。これはウイルスの複製を促進し、宿主の免疫応答を調節すると考えられます。
Further investigations using authentic KP.2 and related JN.1-lineage variants shall help distinguish between possible mechanisms that endow KP.2 with a selective advantage over JN.1 and other subvariants in the pandemic.
本物の KP.2 および関連する JN.1 系統の変異体を使用したさらなる調査は、パンデミックにおいて KP.2 に JN.1 および他のサブバリアントよりも選択的優位性を与える可能性のあるメカニズムを区別するのに役立つでしょう。
Another interesting and somewhat surprising finding of this work is that most of the newly emerged JN.1 subvariants, especially SLip, exhibit decreased infectivity and cell-cell fusion activity in CaLu-3 cells compared to the parental JN.1 (Figures 1 and 5).
この研究で得られたもう 1 つの興味深い、そしていくぶん意外な発見は、新たに出現した JN.1 サブバリアントのほとんど、特に SLip が、CaLu-3 細胞における感染性と細胞間融合活性が親 JN.1 と比較して低下しているという点です (図 1 および 5)。
Flow cytometric and western blotting analyses reveal decreased expression levels of the variant spike proteins on the plasma membrane of virus-producing cells, as well as reduced efficiency of spike processing by furin in the cell.
フローサイトメトリーおよびウェスタンブロッティング分析により、ウイルス産生細胞の細胞膜上のバリアントスパイクタンパク質の発現レベルが低下していること、および細胞内でのフリンによるスパイク処理の効率が低下していることが明らかになりました。
Together, these findings could explain, in part, the observed increase in infectivity and fusion phenotypes.
これらの発見を合わせると、感染性と融合表現型の増加が観察された理由を部分的に説明できます。
Noticeably, these aspects of spike biology differ markedly from their parental BA.2.86 as well as some of the previously dominating Omicron variants, such as XBB.1.5 and EG.5.1, which exhibit increased spike processing, fusogenicity, and/or infectivity in CaLu-3 cells.
注目すべきことに、スパイク生物学のこれらの側面は、CaLu-3 細胞におけるスパイク処理、融合性、および/または感染性の増加を示す親 BA.2.86 や、以前に優勢だった XBB.1.5 や EG.5.1 などの Omicron バリアントの一部とは著しく異なります。
While the mechanism and implications of these differences in spike biology remain to be further investigated, our results suggest that some of these mutations, including F456L, R346T, and V1140L, though beneficial for antibody escape, could negatively impact other aspects of spike biology, highlighting the critical trade-off between immune evasion and viral fitness.
スパイク生物学におけるこれらの違いのメカニズムと意味についてはさらに調査が必要ですが、私たちの研究結果では、F456L、R346T、V1140L などの変異の一部は、抗体回避には有益であるものの、スパイク生物学の他の側面に悪影響を与える可能性があることが示唆されており、免疫回避とウイルスの適応度の間の重要なトレードオフが浮き彫りになっています。
We want to emphasize that our study was conducted using a pseudotyped lentivirus rather than authentic SARS-CoV-2.
私たちの研究は、本物の SARS-CoV-2 ではなく、擬似型レンチウイルスを使用して実施されたことを強調したいと思います。
The two systems have differences, including the virus protein components and the incorporation of the viral glycoprotein on the plasma membrane for the lentivirus versus in the trans-Golgi for authentic SARS-CoV-2.
2 つのシステムには、ウイルスタンパク質成分や、レンチウイルスの場合はウイルス糖タンパク質が細胞膜上に、本物の SARS-CoV-2 の場合はトランスゴルジ体に組み込まれるなどの違いがあります。
Despite these differences, our group and others have previously demonstrated that the pseudotyped lentivirus system can accurately recapitulate infectivity and neutralization of authentic SARS-CoV-2.
これらの違いにもかかわらず、私たちのグループと他のグループは、擬似型レンチウイルスシステムが本物の SARS-CoV-2 の感染性と中和性を正確に再現できることを以前に実証しています。
While the global COVID-19 pandemic has been declared over, SARS-CoV-2 continues to evolve and escape from host immunity elicited by vaccination and/or infections.
COVID-19の世界的パンデミックは終息したと宣言されましたが、SARS-CoV-2は進化を続け、ワクチン接種や感染によって引き起こされた宿主免疫から逃れています。
Our data suggest that more recent JN.1-lineage variants have altered properties in immune escape and biology, and they will continue to evolve.
私たちのデータは、最近のJN.1系統の変異体は免疫逃避と生物学的特性が変化しており、進化し続けることを示唆しています。
Our findings highlight the importance of continued tracking and characterization of emerging variants of SARS-CoV-2.
私たちの研究結果は、SARS-CoV-2の新たな変異体の継続的な追跡と特性評価の重要性を強調しています。
Such studies are especially critical at this stage in the pandemic, when most people have been exposed to the virus at least once, if not several times, and thus have complex immunogenic backgrounds.
パンデミックのこの段階では、ほとんどの人が少なくとも1回、場合によっては数回ウイルスに曝露しており、したがって複雑な免疫原性背景を持っているため、このような研究は特に重要である。
Future vaccine development should consider JN.1 and/or closely related spikes as potential immunogen(s), though XBB.1.5 monovalent vaccines could still offer some protections.
将来のワクチン開発では、JN.1および/または密接に関連するスパイクを潜在的な免疫原として検討する必要があるが、XBB.1.5単価ワクチンはまだいくらかの保護を提供できる可能性がある。
Limitations of the study
Our experiments make use of a pseudotyped lentivirus bearing the spike protein of interest, not authentic infectious SARS-CoV-2 strains.
私たちの実験では、本物の感染性 SARS-CoV-2 株ではなく、対象のスパイクタンパク質を持つ擬似型レンチウイルスを使用しています。
However, the lentivirus model has proven to be an accurate reflection of the neutralization of live SARS-CoV-2 and has been shown to be instrumental for evaluating the efficacy of COVID-19 vaccines.
ただし、レンチウイルス モデルは、生きた SARS-CoV-2 の中和を正確に反映していることが証明されており、COVID-19 ワクチンの有効性を評価するのに役立つことが示されています。
In addition, the timeliness of this work would not allow for thorough higher biosafety level three experiments to be conducted.
さらに、この研究の適時性により、徹底したバイオセーフティ レベル 3 の実験を行うことはできません。
The sample size of our cohorts, particularly the BA.2.86/JN.1 convalescents, is limited because of the institutional review board (IRB) rules and restrictions.
私たちのコホート、特に BA.2.86/JN.1 回復期患者のサンプル サイズは、機関審査委員会 (IRB) の規則と制限により制限されています。
However, other similar studies have worked with cohorts of similar size, and we have also published work with similar cohort sizes with reliable results in the past.
ただし、他の同様の研究は同様のサイズのコホートで行われており、私たちも過去に同様のコホート サイズで信頼性の高い結果を発表しています。
We also want to acknowledge that due to limitations posed by the IRB, our human cohorts are missing demographic information including gender identity, ancestry, race, ethnicity, and socioeconomic status, all of which can have impacts on the data presented in this study.
また、IRB の制限により、私たちのヒトコホートには、性自認、祖先、人種、民族、社会経済的地位などの人口統計情報が欠落しており、これらはすべて、この研究で提示されたデータに影響を及ぼす可能性があることを認識しています。
Because of the relatively small sample size, we are unable to make meaningful statements about the impact of sex or age on the nAb response, and these analyses were not included.
サンプルサイズが比較的小さいため、性別や年齢が nAb 応答に与える影響について意味のある発言をすることはできず、これらの分析は含まれていませんでした。
Nonetheless, data from these cohorts shall provide important insight into the biology of SARS-CoV-2 and offer timely guidance for future COVID-19 vaccine formulations.
とはいえ、これらのコホートのデータは、SARS-CoV-2 の生物学に関する重要な洞察を提供し、将来の COVID-19 ワクチン製剤にタイムリーなガイダンスを提供します。
We recognize that homology modeling is not as precise as authentic cryoelectron microscopy structures, and the impact of key mutations on ACE2 interaction and antibody engagement would require confirmation by further structural studies.
相同性モデリングは本物のクライオ電子顕微鏡構造ほど正確ではなく、主要な変異が ACE2 相互作用と抗体関与に与える影響については、さらなる構造研究による確認が必要であることを認識しています。
以下省略。
この記事が気に入ったらサポートをしてみませんか?