RBD 構造およびVOC間の共受容体結合における N343 グリコシル化の役割
#GotGlycans : Role of N343 Glycosylation on the SARS-CoV-2 S RBD Structure and Co-Receptor Binding Across Variants of Concern
#GotGlycans : SARS-CoV-2 S RBD 構造における N343 グリコシル化の役割と懸念される変異体間の共受容体結合
Abstract
Glycosylation of the SARS-CoV-2 spike (S) protein represents a key target for viral evolution because it affects both viral evasion and fitness.
SARS-CoV-2 スパイク (S) タンパク質のグリコシル化は、ウイルス回避と適応度の両方に影響を与えるため、ウイルス進化の重要な標的となります。
Successful variations in the glycan shield are difficult to achieve though, as protein glycosylation is also critical to folding and to structural stability.
ただし、タンパク質のグリコシル化はフォールディングと構造の安定性にとっても重要であるため、グリカンシールドの変化を成功させるのは困難です。
Within this framework, the identification of glycosylation sites that are structurally dispensable can provide insight into the evolutionary mechanisms of the shield and inform immune surveillance.
この枠組みの中で、構造的に不要なグリコシル化部位を同定することで、シールドの進化メカニズムへの洞察が得られ、免疫監視に情報を提供することができます。
In this work we show through over 45 μs of cumulative sampling from conventional and enhanced molecular dynamics (MD) simulations, how the structure of the immunodominant S receptor binding domain (RBD) is regulated by N-glycosylation at N343 and how this glycan’s structural role changes from WHu-1, alpha (B.1.1.7), and beta (B.1.351), to the delta (B.1.617.2) and omicron (BA.1 and BA.2.86) variants.
この研究では、従来の分子動力学 (MD) シミュレーションと強化された分子動力学 (MD) シミュレーションからの 45 μs を超える累積サンプリングを通じて、免疫優性 S 受容体結合ドメイン (RBD) の構造が N343 の N-グリコシル化によってどのように制御されるか、このグリカンの構造的役割が WHu-1、アルファ (B.1.1.7)、ベータ (B.1.351) からデルタ (B.1.617.2) およびオミクロン (BA.1 および BA.2.86) バリアントにどのように変化するかを示します。
More specifically, we find that the amphipathic nature of the N-glycan is instrumental to preserve the structural integrity of the RBD hydrophobic core and that loss of glycosylation at N343 triggers a specific and consistent conformational change.
より具体的には、N-グリカンの両親媒性の性質がRBD疎水性コアの構造的完全性を維持するのに役立ち、N343でのグリコシル化の喪失が特異的かつ一貫した構造変化を引き起こすことを我々は見出した。
We show how this change allosterically regulates the conformation of the receptor binding motif (RBM) in the WHu-1, alpha and beta RBDs, but not in the delta and omicron variants, due to mutations that reinforce the RBD architecture.
我々は、RBD構造を強化する変異により、この変化がWHu-1、アルファおよびベータRBDの受容体結合モチーフ(RBM)の立体構造をアロステリックに制御するが、デルタおよびミクロン変異体では制御しないことを示す。
In support of these findings, we show that the binding of the RBD to monosialylated ganglioside co-receptors is highly dependent on N343 glycosylation in the WHu-1, but not in the delta RBD, and that affinity changes significantly across VoCs.
これらの発見を裏付けるように、モノシアル化ガングリオシド共受容体への RBD の結合は、WHu-1 の N343 グリコシル化に大きく依存するが、デルタ RBD では依存しないこと、および親和性が VoC 間で大きく変化することを示します。
Ultimately, the molecular and functional insight we provide in this work reinforces our understanding of the role of glycosylation in protein structure and function and it also allows us to identify the structural constraints within which the glycosylation site at N343 can become a hotspot for mutations in the SARS-CoV-2 S glycan shield.
最終的に、この研究で私たちが提供する分子的および機能的洞察は、タンパク質の構造と機能におけるグリコシル化の役割についての理解を強化します。 また、N343 のグリコシル化部位が SARS-CoV-2 S グリカン シールドの変異のホットスポットになり得る構造的制約を特定することもできます。
Introduction
The SARS-CoV-2 spike (S) glycoprotein is responsible for viral fusion with the host cell, initiating an infection that leads to COVID-19.
SARS-CoV-2 スパイク (S) 糖タンパク質は、ウイルスと宿主細胞の融合を担い、COVID-19 につながる感染を開始します。
S is a homotrimer with a structure subdivided in two topological domains, namely S1 and S2, see Figure 1a, separated by a furin site, which is cleaved in the pre-fusion architecture.
S は、2 つのトポロジカル ドメイン、つまり S1 と S2 に細分される構造を持つホモ三量体です (図 1a を参照)。フューリン部位によって分離されており、融合前構造で切断されます。
In the Wuhan-Hu-1 strain (WHu-1), and still in most variants of concern (VoCs), host cell fusion is predominantly triggered by S binding to the Angiotensin-Converting Enzyme 2 (ACE2) receptor located on the host cell surface.
武漢-Hu-1株(WHu-1)、そして依然として懸念されるほとんどの変異株(VoC)では、 宿主細胞融合は主に、宿主細胞表面にあるアンジオテンシン変換酵素 2 (ACE2) 受容体への S 結合によって引き起こされます。
This process is supported by glycan co-receptors, such as heparan sulfate (HS) in the extracellular matrix and by monosialylated gangliosides oligosaccharides (GM1os and GM2os) peeking from the surface of the host cells.
このプロセスは、細胞外マトリックス内のヘパラン硫酸 (HS) などのグリカン共受容体と、宿主細胞の表面から覗くモノシアル化ガングリオシド オリゴ糖 (GM1os および GM2os) によってサポートされています。
The interaction with ACE2 requires a dramatic conformational change of the S, known as ‘opening’, where one or more Receptor Binding Domains (RBDs) in the S1 subdomain become exposed.
ACE2 との相互作用には、「開口」として知られる S の劇的な構造変化が必要であり、S1 サブドメイン内の 1 つ以上の受容体結合ドメイン (RBD) が露出します。
The region of the RBD in direct contact with the ACE2 surface is known as receptor binding motif (RBM).
ACE2 表面と直接接触する RBD の領域は、受容体結合モチーフ (RBM) として知られています。
Ultimately, S binding to ACE2 causes shedding of the S1 subdomain and the transition to a post-fusion conformation, which exposes the fusion peptide near the host cell surface, leading to viral entry.
最終的に、S が ACE2 に結合すると、S1 サブドメインが脱落し、融合後構造への移行が引き起こされます。 これにより、宿主細胞表面近くの融合ペプチドが露出され、ウイルスの侵入が引き起こされます。
To exert its functions, S sticks out from the viral envelope where it is exposed to recognition.
その機能を発揮するために、S はウイルスのエンベロープから突き出て認識にさらされます。
To evade the host immune system, enveloped viruses hijack the host cell’s glycosylation machinery to cover S with a dense coat of host carbohydrates, known as a glycan shield.
宿主免疫系を回避するために、エンベロープウイルスは宿主細胞のグリコシル化機構を乗っ取り、グリカンシールドとして知られる宿主炭水化物の緻密なコートでSを覆います。
In SARS-CoV-2 the glycan shield screens effectively over 60% of the S protein surface, leaving the RBD, when open, and regions of the N-terminal domain (NTD) vulnerable to immune recognition.
SARS-CoV-2 では、グリカン シールドが S タンパク質表面の 60% 以上を効果的に遮蔽し、RBD が開いたままにし、N 末端ドメイン (NTD) の領域を免疫認識に対して脆弱にします。
The RBD targeted by approximately 90% of serum neutralising antibodies and thus a highly effective model not only to screen antibody specificity and interactions with host cell co-receptors, but also as a protein scaffold for COVID-19 vaccines.
RBD は血清中和抗体の約 90% を標的とするため、抗体の特異性や宿主細胞の共受容体との相互作用をスクリーニングするだけでなく、COVID-19ワクチンのタンパク質足場としても非常に効果的なモデルです。
As a direct consequence the RBD is under great evolutionary pressure.
その直接的な結果として、RBD は大きな進化圧力にさらされています。
Mutations of the RBD leading to immune escape are particularly concerning, especially when such changes enhance the binding affinity for ACE2 or give access to alternative entry routes.
免疫回避を引き起こす RBD の変異は、特にそのような変化によって ACE2 への結合親和性が強化される場合や、別の侵入経路へのアクセスが可能になる場合に特に懸念されます。
The identification of mutational hotspots and the effects of mutations in and around the RBM have been and are under a great deal of scrutiny.
突然変異のホットスポットの特定と、RBM 内およびその周囲の突然変異の影響については、これまでも、そして現在も厳しい精査が行われています。
Yet, less attention is devoted to mutations in the glycan shield, which have been shown to lead to dramatic changes in infectivity and in immune escape.
しかし、感染力や免疫逃避に劇的な変化をもたらすことがわかっているグリカンシールドの変異にはあまり注目されていません。
Successful changes in the glycan shield are evolutionarily difficult to achieve, due to the fact that the nature and pattern of glycosylation of the S is crucial not only to the efficiency of viral entry and evasion, but also to facilitate folding and to preserve the structural integrity of the functional fold.
糖鎖シールドの変化を成功させることは進化的に困難です。これは、S のグリコシル化の性質とパターンがウイルスの侵入と回避の効率にとって重要であるだけでなく、フォールディングを促進し、機能的なフォールディングの構造的完全性を維持するためにも重要であるという事実によるものです。
Therefore, identifying potential evolutionary hotspots in the S shield is a complex matter, yet of crucial importance to immune surveillance.
したがって、Sシールド内の潜在的な進化的ホットスポットを特定することは複雑な問題ですが、免疫監視にとって非常に重要です。
Potential changes of the shield, e.g. loss, shift or gain of new glycosylation sites, can likely occur only where these do not negatively impact the integrity of the underlying, functional protein architecture.
新しいグリコシル化部位の喪失、シフト、獲得などのシールドの潜在的な変化は、基礎となる機能的なタンパク質構造の完全性に悪影響を及ぼさない場合にのみ発生する可能性があります。
In this work we present and discuss the case of N343, a key glycosylation site on the RBD.
この研究では、RBD 上の重要なグリコシル化部位である N343 のケースを提示し、議論します。
Results of extensive sampling from molecular dynamics (MD) simulations exceeding 45 μs, show how the loss of N-glycosylation at N343 affects the structure, dynamics and co-receptor binding of the RBD and how these effects are modulated by mutations in the underlying protein, going from the WHu-1 strain through the VoCs designated as alpha (B.1.1.7), beta (B.1.351), delta (B.1.617.2) and omicron (BA.1).
45 μs を超える分子動力学 (MD) シミュレーションからの大規模なサンプリングの結果は、次を示しています。つまり、 N343 での N-グリコシル化の喪失が RBD の構造、動態、共受容体結合にどのような影響を与えるか、そしてこれらの効果が、WHu-1株から指定されたVoC(アルファ株・ベータ株・デルタ株・オミクロン株)に至るまで、基礎となるタンパク質の変異によってどのように調節されるのか。
In addition, we provide important insight into the structure and dynamics of the omicron BA.2.86 RBD.
さらに、我々は、オミクロン BA.2.86 RBD の構造とダイナミクスについての重要な洞察を提供します。
This variant, designated as variant under monitoring (VUM) and commonly referred to as ‘pirola’, carries a newly gained N-glycosylation site at N354, which represents the first change in the RBD shielding since the ancestral strain.
モニタリング中の変異体(VUM)として指定され、一般に「ピロラ」と呼ばれるこの変異体は、N354に新たに獲得されたN-グリコシル化部位を有しており、これは祖先株以来のRBDシールドの最初の変化を表している。
The SARS-CoV-2 S RBD (aa 327-540) from the WHu-1 (China, 2019) to the EG.5.1 (China, 2023) shows two highly conserved N-glycosylation sites, one at N331 and the other at N343.
WHu-1 (中国、2019) から EG.5.1 (中国、2023) までの SARS-CoV-2 S RBD (aa 327-540) は、高度に保存された 2 つの N-グリコシル化部位 (1 つは N331、もう 1 つは N343) を示しています。
While glycosylation at N331 is located on a highly flexible region linking the RBD to the NTD, the N343 glycan covers a large portion of the RBD, stretching across the protein surface and forming a bridge connecting the two helical regions that frame the beta sheet core, see Figure 1b.
N331 のグリコシル化は RBD を NTD に連結する柔軟性の高い領域に位置しますが、N343 グリカンは RBD の大部分を覆い、タンパク質表面全体に伸びて、ベータ シート コアを構成する 2 つのらせん領域を接続する橋を形成します。 図 1b を参照してください。
In this work we show that removal of the N343 glycan induces a conformational change which in WHu-1, alpha and beta allosterically controls the structure and dynamics of the RBM, see Figure 1c.
この研究では、N343 グリカンの除去により立体構造変化が誘導され、WHu-1 ではアルファおよびベータが RBM の構造とダイナミクスをアロステリックに制御することを示します (図 1c を参照)。
In delta and omicron these effects are significantly dampened by mutations that strengthen the RBD architecture.
デルタおよびオミクロンでは、これらの効果は、RBD アーキテクチャを強化する突然変異によって大幅に弱められます。
Further to this molecular insight, we show that enzymatic removal of the N343 glycan affects binding of monosialylated ganglioside co-receptors in the WHu-1 RBD, but not in delta.
この分子的洞察に加えて、我々は、N343 グリカンの酵素的除去が WHu-1 RBD のモノシアル化ガングリオシド共受容体の結合に影響を与えるが、デルタ株では影響しないことを示します。
We also observe that the affinity of the RBD for GM1 GM1os and GM2 GM1os changes significantly across the VoCs, with beta and omicron exhibiting the weakest binding.
また、GM1 GM1os および GM2 GM1os に対する RBD の親和性が VoC 間で大きく変化し、ベータとオミクロンが最も弱い結合を示すことも観察されました。
Ultimately, the molecular insight we provide in this work adds to the ever growing evidence supporting the role of glycosylation in protein folding and structural stability.
最終的に、この研究で我々が提供する分子的洞察は、タンパク質のフォールディングと構造安定性におけるグリコシル化の役割を裏付ける証拠をさらに増やします。
This information is not only central to structural biology, but also critical to the design of novel COVID-19 vaccines that may or may not carry glycans, as well as instrumental to our understanding of the evolutionary mechanisms regulating the shield.
この情報は構造生物学の中心であるだけでなく、糖鎖を含む場合と含まない場合がある新しいCOVID-19ワクチンの設計にも重要であり、シールドを制御する進化メカニズムを理解するのにも役立ちます。
Results
In this section we start with a brief overview of the architecture of the RBD, we then explain how the RBD structure is modulated by interactions with ACE2 and why the N343 glycan is integral to its stability.
このセクションでは、RBD の構造の簡単な概要から始めて、RBD 構造が ACE2 との相互作用によってどのように調節されるのか、そしてなぜ N343 グリカンがその安定性に不可欠であるのかについて説明します。
We then describe how and why the loss of N343 glycosylation affects the RBD structure and its binding affinity for GM1os and GM2os to different degrees in the VoCs.
次に、N343 グリコシル化の喪失が VoC の RBD 構造と GM1os および GM2os に対する結合親和性にさまざまな程度で影響を与える方法と理由を説明します。
SARS-CoV-2 S RBD structure and antigenicity.
SARS-CoV-2 S RBD の構造と抗原性。
The SARS-CoV-2 S RBD encompasses both structured and intrinsically disordered regions.
SARS-CoV-2 S RBD には、構造化された領域と本質的に無秩序な領域の両方が含まれます。
The structured region is supported by a largely hydrophobic beta sheet core, framed by two flanking, partially helical loops (aa 335-345 and aa 365-375), linked by a bridging N-glycan at N343, see Figure 1b.
構造化領域は、N343の架橋N-グリカンによって連結された2つの隣接する部分的に螺旋状のループ(aa 335-345およびaa 365-375)で囲まれた、主に疎水性のベータシートコアによって支えられています(図1bを参照)。
The aa 335-345 loop carries the N343 glycosylation site and it is part of an important antigenic region targeted by Class 2 and 3 antibodies.
aa 335-345 ループには N343 グリコシル化部位があり、クラス 2 およびクラス 3 抗体の標的となる重要な抗原領域の一部です。
In the bridging conformation, the N343 glycan pentasaccharide extends across the RBD beta sheet to reach the aa 365-375 loop forming highly populated hydrogen bonding and dispersion interactions with the backbone and with the sidechains of residues 365 to 375, see Figures 1b,c and S.2.
架橋構造では、N343 グリカン五糖は RBD ベータ シート全体に伸びてアミノ酸 365 ~ 375 ループに到達し、バックボーンおよび残基 365 ~ 375 の側鎖との高密度の水素結合および分散相互作用を形成します。 図 1b、c、および S.2 を参照してください。
The bridging N343 glycan shields the hydrophobic beta sheet core of the RBD from the surrounding water, preventing energetically unfavourable contacts.
架橋N343グリカンはRBDの疎水性ベータシートコアを周囲の水から守り、エネルギー的に好ましくない接触を防ぎます。
Due to its amphipathic nature, the N343 forms dispersion interactions with the hydrophobic residues of the beta sheet through its core GlcNAc-β(1-4)GlcNAc, while engaging in hydrogen bonds with the surrounding water and with the aa 365-375 helical loop.
その両親媒性の性質により、N343 はそのコア GlcNAc-β(1-4)GlcNAc を介してベータ シートの疎水性残基と分散相互作用を形成します。 同時に、周囲の水およびaa 365-375のらせんループと水素結合します。
Notably, the key anchoring residues S371, S373 and S375 within this loop are all mutated to hydrophobic residues in all omicron variants (BA.1-2, BA.4-5, BQ.1.1, EG.5.1, XBB.1.5).
注目すべきことに、このループ内の重要なアンカー残基 S371、S373、および S375 はすべて、すべてのオミクロン バリアント (BA.1-2、BA.4-5、BQ.1.1、EG.5.1、XBB.1.5) で疎水性残基に変異しています。
The receptor binding motif (RBM) encompasses aa 439-506 and counts all the RBD residues in direct contact with ACE2.
受容体結合モチーフ (RBM) は aa 439 ~ 506 を包含し、ACE2 と直接接触するすべての RBD 残基をカウントします。
The RBM is heavily targeted by both Class 1 and 2 antibodies and under high evolutionary pressure, with all VoCs carrying mutations in this region.
RBM はクラス 1 とクラス 2 の両方の抗体によって強く標的にされ、高い進化圧力下にあり、すべての VoC がこの領域に変異を持っています。
As shown by earlier MD simulations studies, the RBM in unbound S is largely unstructured and dynamic, an insight also supported by the low resolution cryo-EM maps of this region.
以前の MD シミュレーション研究で示されているように、非結合 S の RBM は大部分が構造化されておらず動的であり、この洞察はこの領域の低解像度クライオ EM マップによっても裏付けられています。
The RBM’s inherent flexibility is likely an important feature in the opening and closing mechanism of the RBD, where the N343 from adjacent RBDs engage with the protein in closed conformation and gate RBD opening.
RBM の固有の柔軟性は、RBD の開閉メカニズムにおける重要な特徴であると考えられます。 ここで、隣接する RBD からの N343 が閉じた立体構造でタンパク質と結合し、RBD が開きます。
The only relatively structured region of the RBM is what we define here on as the hydrophilic patch, see Figure 1b, a hairpin stabilised by a network of interlocking salt-bridges and polar residues, namely R454, R457, K458, K462, E465, D467, S469, and E471, that faces the interior of the S when the RBD is closed.
RBM の唯一の比較的構造化された領域は、ここで親水性パッチとして定義されるものです (図 1b を参照)。 ヘアピンは、連結した塩橋と極性残基、すなわち R454、R457、K458、K462、E465、D467、S469、および E471 のネットワークによって安定化され、RBD が閉じているときに S の内部に面します。
When in complex with ACE2 or with antibodies, the RBM adopts a structured fold, also shared by the SARS-CoV-1 S RBD.
ACE2 または抗体と複合体を形成すると、RBM は SARS-CoV-1 S RBD と共有される構造化された折り畳みを採用します。
In this conformation only the terminal hairpin of the RBM (aa 476 to 486) retains a high degree of flexibility, as shown in this work and by others.
この立体構造では、この研究や他の研究者によって示されているように、RBM の末端ヘアピン (aa 476 ~ 486) のみが高度な柔軟性を保持しています。
The RBM bound fold is stabilised by a hydrophobic patch supported by the stacking of the aromatic and aliphatic residues L455, F456, Y473, A475, see Figure 1b, which are part of the protein interface with ACE2.
RBM 結合フォールドは、芳香族残基と脂肪族残基 L455、F456、Y473、A475 の積み重ねによってサポートされる疎水性パッチによって安定化されます (図 1b を参照)。 これらは、ACE2とのタンパク質インターフェースの一部です。
Notably, all residues in the hydrophobic and hydrophilic patches are highly conserved across the VoCs, possibly due to their critical function in inducing and/or stabilising the RBD into its ACE2-bound conformation.
注目すべきことに、疎水性および親水性パッチ内のすべての残基は、おそらく RBD を ACE2 結合立体構造に誘導および/または安定化する重要な機能のため、VoC 全体で高度に保存されています。
As an interesting observation, the loss of stacking in the hydrophobic patch due to the recent F456L mutation in the EG.5.1 variant (China, 2023) is recovered by the L455F mutation in the, appropriately named, FLip variant.
興味深い観察として、EG.5.1 変異体 (中国、2023) における最近の F456L 変異による疎水性パッチでのスタッキングの喪失は、適切に名付けられた FLip 変異体における L455F 変異によって回復されています。
Based on evidence from screenings, we subdivided the RBD into three different antigenic regions known to be targeted by different classes of antibodies, see Figure 1d.
スクリーニングからの証拠に基づいて、我々はRBDを、異なるクラスの抗体の標的となることが知られている3つの異なる抗原領域に細分しました。図1dを参照してください。
Region 1 stretches from aa 337-353, which includes the N343 glycosylation site, and counts residues targeted by class 2 and 3 antibodies.
領域 1 は、N343 グリコシル化部位を含む aa 337 ~ 353 に広がり、クラス 2 および 3 抗体の標的となる残基をカウントします。
The aa sequence in Region 1 has been highly conserved so far, allowing specific antibodies to retain their neutralisation activity across all VoCs, such as S309, whose binding mode also directly involves the N343 glycan.
領域 1 の aa 配列はこれまでのところ高度に保存されており、S309 などのすべての VoC にわたって特定の抗体が中和活性を保持できます。S309 の結合モードには N343 グリカンも直接関与します。
A notable and dramatic exception to this high degree of conservation in Region 1 is given by the BA.2.86 variant (Denmark, 2023), known as ‘pirola’, where the K356T mutation introduces a new N-glycosylation sequon at N354.
領域 1 におけるこの高度な保存性の注目すべき劇的な例外は、「ピロラ」として知られる BA.2.86 変異体 (デンマーク、2023) です。この変異体では、K356T 変異により N354 に新しい N-グリコシル化セクオンが導入されています。
Region 2 coincides with the RBM, which, in addition to binding ACE2 and neutralising antibodies, used to bind the N370 glycan from adjacent RBDs.
領域 2 は RBM と一致しており、ACE2 および中和抗体への結合に加えて、隣接する RBD からの N370 グリカンへの結合にも使用されます。
N370 glycosylation is lost in SARS-CoV-2 with the RBM binding cleft available to bind glycan co-receptors, such as glycosaminoglycans, blood group antigens, monosialylated gangliosides, among others.
SARS-CoV-2 では N370 グリコシル化が失われており、特にグリコサミノグリカン、血液型抗原、モノシアル化ガングリオシドなどのグリカン共受容体に結合するために利用できる RBM 結合裂が存在します。
Region 3 is a short, relatively structured loop stretching between aa 411-426, located on the opposite side of the RBD relative to Region 1, see Figure 1d.
領域 3 は、aa 411 ~ 426 の間に伸びる短い比較的構造化されたループで、領域 1 に対して RBD の反対側に位置します (図 1d を参照)。
Effect of the loss of N343 glycosylation on the structure of the WHu-1, alpha and beta RBDs.
WHu-1、アルファおよびベータ RBD の構造に対する N343 グリコシル化の喪失の影響。
Results obtained for the WHu-1 strain and for the alpha (B.1.1.7) and beta (B.1.351) VoCs are discussed together due to their sequence and structure similarity, with alpha counting only one mutation (N501Y) and beta three mutations (K417N, E484K and N501Y) relative to the WHu-1 RBD.
WHu-1 株とアルファ (B.1.1.7) およびベータ (B.1.351) VoC について得られた結果は、それらの配列と構造の類似性により一緒に議論されます。 WHu-1 RBDと比較して、アルファは1つの突然変異(N501Y)のみをカウントし、ベータは3つの突然変異(K417N、E484KおよびN501Y)をカウントします。
Extensive sampling through conventional MD, i.e. 4 μs for the alpha and beta VoC and 8 μs with an additional 4 μs of Gaussian accelerated MD (GaMD) for the WHu-1 RBD, see Table S.1, shows that the loss of N343 glycosylation induces a dramatic conformational change in the RBD, where one or both helical loops flanking the hydrophobic beta sheet core pull towards each other, see Figure 2.
従来のMDによる広範なサンプリング、 つまり、アルファおよびベータ VoC では 4 μs、WHu-1 RBD では 8 μs とガウス加速 MD (GaMD) の追加 4 μs(表S.1を参照)は、N343 グリコシル化の喪失が RBD の劇的な構造変化を誘導することを示しています。疎水性ベータシートコアに隣接する一方または両方の螺旋ループが互いに引き寄せられます。 図 2 を参照してください。
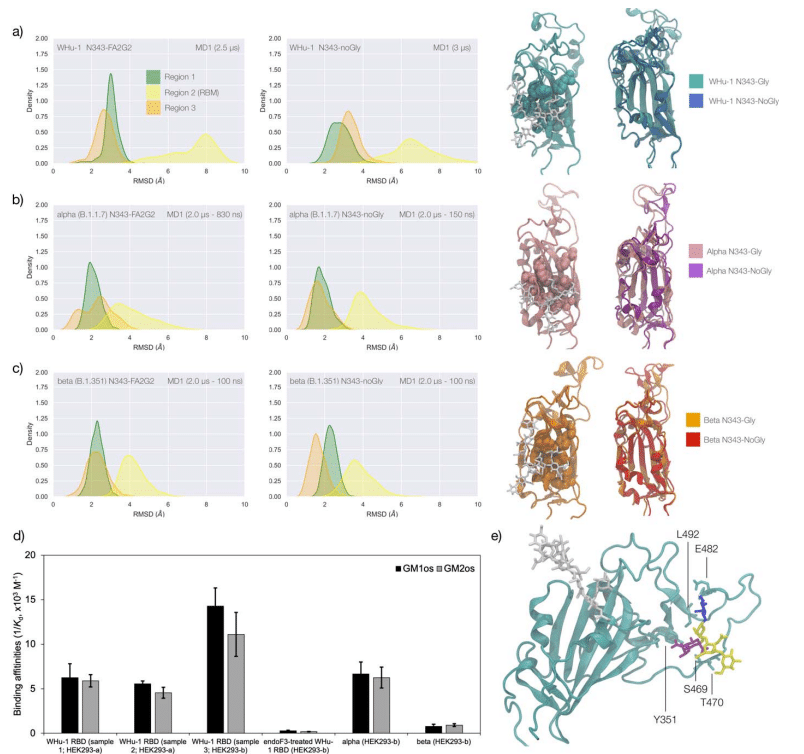
This conformational change can occur very rapidly upon removal of the N-glycan or after a longer delay due to the complexity of the conformational energy landscape.
この構造変化は、N-グリカンの除去時に非常に急速に起こることもあれば、構造エネルギー状況の複雑さにより長い遅れの後に起こることもあります。
The data used for the analysis corresponds to systems that have reached structural stability, i.e. equilibrium; we discarded the timeframes corresponding to conformational transitions.
分析に使用されるデータは、構造的安定性、つまり平衡に達したシステムに対応します; 構造遷移に対応する時間枠を破棄しました。
To explore the effects of the loss of N343 glycosylation in the WHu-1 RBD, we started the MD simulations from different conformations.
WHu-1 RBD における N343 グリコシル化の喪失の影響を調べるために、さまざまな立体構造から MD シミュレーションを開始しました。
In one set of conventional sampling MD trajectories (MD1) and in the GaMD simulations the starting structure corresponds to an open RBD from an MD equilibrated S ectodomain obtained in earlier work.
従来のサンプリング MD 軌道 (MD1) の 1 セットと GaMD シミュレーションでは、開始構造は以前の研究で得られた MD 平衡化外部ドメインからの開いた RBD に対応します。
In this system the RBM is unfolded and retains the maximum degree of flexibility.
このシステムでは、RBM は展開され、最大限の柔軟性が維持されます。
MD2 was started from a conformation corresponding to the ACE2-bound structure.
MD2 は、ACE2 結合構造に対応する立体構造から開始されました。
Results obtained from MD2 and GaMD are entirely consistent with results from MD1, and thus are included as Supplementary Material in Figure S.2.
MD2 および GaMD から得られた結果は、MD1 の結果と完全に一致しています。 したがって、図 S.2 に補足資料として含まれています。
The GaMD simulation shows a lower degree of contact of the N343 glycan with the aa 365-375 stretch of the opposite loop, see Figure 1c, because most of the contacts are with residues further downstream from position 365.
GaMD シミュレーションでは、N343 グリカンと反対側のループの aa 365-375 ストレッチとの接触度が低いことが示されています (図 1c を参照)。これは、ほとんどの接触が 365 位からさらに下流の残基とであるためです。
Nevertheless, the N343 remains engaged in a bridging conformation throughout the simulation.
それにもかかわらず、N343 はシミュレーション全体を通じて架橋構造を維持したままになります。
As shown by the RMSD values distributions, represented through Kernel Density Estimates (KDE) in Figure 2, the structure of Region 1 in the WHu-1 RBD is stable.
図 2 のカーネル密度推定 (KDE) によって表される RMSD 値の分布が示すように、 WHu-1 RBD の領域 1 の構造は安定しています。
In the glycosylated RBD the stability of Region 1 is largely due to the contribution of the bridging N343 FA2G2 glycan, forming hydrogen bonds with the residues in loop aa 365-375 throughout the simulations, see Figures 1c and S.2.
グリコシル化された RBD では、領域 1 の安定性は主に、シミュレーションを通じてループ aa 365 ~ 375 の残基と水素結合を形成する架橋 N343 FA2G2 グリカンの寄与によるものです。図 1c および S.2 を参照してください。
Conversely the conformation of the RBM (Region 2) is very flexible in both glycosylated and nonglycosylated forms.
逆に、RBM (領域 2) の立体構造は、グリコシル化型と非グリコシル化型の両方で非常に柔軟です。
Loss of N343 glycosylation triggers a conformational change in the Region 1 of the WHu-1 RBD, shown by a broader KDE peak in Figure 2a.
N343 グリコシル化の喪失は、WHu-1 RBD の領域 1 の構造変化を引き起こします。これは、図 2a のより広い KDE ピークによって示されています。
This conformational change ultimately triggers the complete detachment of the hydrophilic loop from Region 1, see Figure 1c, through rupture of the non-covalent interactions network between Y351 (Region 1) and S469 or T470 (Region 2) via of hydrogen bonding, and Y351 and L452 (Region 2) via CH-π stacking.
水素結合による Y351 (領域 1) と S469 または T470 (領域 2) の間の非共有相互作用ネットワークの破壊、および CH-π スタッキングによる Y351 と L452 (領域 2) の非共有相互作用ネットワークの破壊によって、この構造変化は最終的に領域 1 からの親水性ループの完全な分離を引き起こします (図 1c を参照)。
Structural changes in Region 3 upon loss of glycosylation at N343 appear to be negligible.
N343 でのグリコシル化の喪失による領域 3 の構造変化は無視できるほどであるようです。
The starting structure used for the simulations of the alpha RBD derives from the ACE2-bound conformation of the WHu-1 RBD (PDB 6M0J) modified with the N501Y mutation.
アルファ RBD のシミュレーションに使用される開始構造は、N501Y 変異で修飾された WHu-1 RBD (PDB 6M0J) の ACE2 結合立体配座に由来します。
The reconstructed glycan at N343 interacts with the aa 365-375 throughout the entire trajectory, but it adopts a stable conformation only after 830 ns, where we started collecting the data shown in Figure 2b.
N343 で再構築されたグリカンは、軌道全体を通じて aa 365-375 と相互作用しますが、安定した構造をとるのは 830 ns 以降です。 ここで、図 2b に示すデータの収集を開始しました。
Again, we see that the loss of glycosylation at N343 causes a swift conformational change that brings the aa 335-345 and aa 365-375 loops closer together, see Figure 2b.
ここでも、N343 でのグリコシル化の喪失により、aa 335-345 ループと aa 365-375 ループが互いに近づく急速な構造変化が引き起こされることがわかります (図 2b を参照)。
This conformational change involves primarily Region 1, and just like the previous case, it ultimately determines the detachment of the hydrophilic patch from the Y351 in Region 1.
この構造変化は主に領域 1 に関係し、前のケースと同様に、最終的には領域 1 の Y351 からの親水性パッチの剥離を決定します。
Also shown by the KDE plot in Figure 2b, a small conformational change in Region 3, which involves a partial disruption and refolding of a helical turn, can be observed during the trajectory of the N343 glycosylated alpha RBD.
また、図2bのKDEプロットに示されているように、N343グリコシル化αRBDの軌道中に、らせんターンの部分的な破壊と再折り畳みを伴う領域3の小さな構造変化が観察できます。
As in the previous case the structure of Region 3 appears to be unaffected by N343 glycosylation, at least within the sampling accumulated in this work.
前のケースと同様に、少なくともこの研究で蓄積されたサンプリング内では、領域 3 の構造は N343 グリコシル化の影響を受けないようです。
In the beta RBD (starting structure from PDB 7LYN) the reconstructed N343 glycan adopts a bridging conformation quite rapidly and retains this conformation throughout the trajectory with only minor deviations.
ベータ RBD (PDB 7LYN からの開始構造) では、再構築された N343 グリカンは非常に急速に架橋構造を採用し、わずかな偏差のみで軌道全体にわたってこの構造を維持します。
The corresponding RMSD values KDE distributions for Regions 1 to 3, see Figure 2c, reflect this structural stability.
領域 1 ~ 3 の対応する RMSD 値 KDE 分布 (図 2c を参照) は、この構造安定性を反映しています。
The stability of the RBM (Region 2) is supported by interactions between Y351 (Region 1) and the hydrophilic loop, as noted earlier.
前述したように、RBM (領域 2) の安定性は、Y351 (領域 1) と親水性ループの間の相互作用によってサポートされています。
Loss of glycosylation at N343 causes a rapid tightening of the RBD core helical loops towards each other, which again in this case ultimately causes the detachment of the hydrophilic loop from Y351 in Region 1 towards the end of the MD trajectory, i.e. after 1.9 μs of sampling.
N343 でのグリコシル化の喪失により、RBD コアのらせんループが相互に急速に緊密になり、この場合も最終的には MD 軌道の終わりに向かって領域 1 の Y351 から親水性ループが剥離します。 つまり、サンプリングの 1.9 μs 後です。
Effect of the loss of N343 glycosylation on the binding affinity of GM1os /2 os for the WHu-1, alpha and beta RBDs.
WHu-1、アルファおよびベータ RBD に対する GM1os /2 os の結合親和性に対する N343 グリコシル化の喪失の影響。
Earlier work from some of us shows that the WHu-1 RBD binds monosialylated gangliosides as co-receptors in SARS-CoV-2 infection.
私たちの何人かによる以前の研究では、WHu-1 RBDがSARS-CoV-2感染における共受容体としてモノシアル化ガングリオシドに結合することが示されています。
The 3D structure of the low affinity complex between the GM1os and the WHu-1 RBD has been proven difficult to obtain experimentally, yet we were able to obtain a promising model through a combination of multiple structural alignments of the GM1os to the WHu-1 RBD-bound N370 and extensive MD simulations, details in Supplementary Material and in ref.
GM1os と WHu-1 RBD 間の低親和性複合体の 3D 構造は、実験的に取得することが困難であることが証明されています。それでも、WHu-1 RBD結合N370に対するGM1osの複数の構造アラインメントと広範なMDシミュレーションの組み合わせを通じて、有望なモデルを得ることができました。詳細は補足資料および参考文献を参照してください。
The predicted GM1os binding site is located at the junction between Region 1 and Region 2 of the WHu-1 RBD and it involves all the residues that stabilise the region, namely Y351, L452, S469 and T470, see Figures 1c and 2e.
予測される GM1os 結合部位は、WHu-1 RBD の領域 1 と領域 2 の間の接合部に位置し、この領域を安定化するすべての残基、すなわち Y351、L452、S469 および T470 が関与します。図 1c と 2e を参照してください。
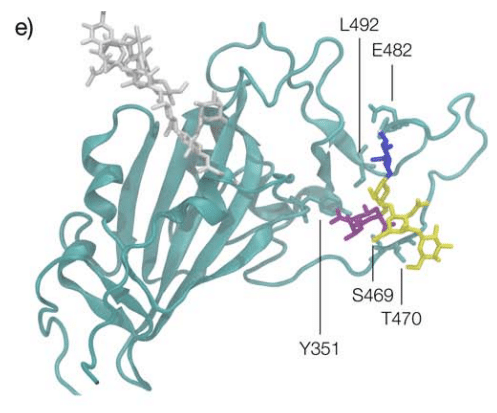
As part of our investigation of glycan co-receptors binding to the SARS-CoV-2 RBD, we used direct ESI-MS assay to determine the impact of the loss of N343 glycosylation on GM1os and GM2os binding.
SARS-CoV-2 RBD に結合するグリカン共受容体に関する研究の一環として、直接 ESI-MS アッセイを使用して、N343 グリコシル化の喪失が GM1os および GM2os の結合に及ぼす影響を調べました。
Here, we used endoF3-treatment to trim down the fucosylated biantennary and triantennary complex N-glycans into core nonfucosylated or fucosylated GlcNAc (Gn or GnF, respectively).
ここでは、endoF3 処理を使用して、フコシル化二分岐および三分岐複合 N-グリカンをコアの非フコシル化またはフコシル化 GlcNAc (それぞれ Gn または GnF) にトリミングしました。
LC-MS analysis suggests that N-glycans on N343 but not N331 of WHu-1 RBD were trimmed down (Figure S.6).
LC-MS 分析は、WHu-1 RBD の N331 ではなく N343 上の N-グリカンがトリミングされたことを示唆しています (図 S.6)。
From the zero-charge mass spectra of endoF3-treated WT RBD (Figure S.5), we performed glycan assignment (Table S.8) and found that 31% of detected glycoforms contained Gn/GnF at N343 while the remaining was the intact form.
endoF3 処理 WT RBD のゼロ電荷質量スペクトルから (図 S.5)、 グリカンの割り当てを実行したところ (表 S.8)、検出されたグリコフォームの 31% には N343 に Gn/GnF が含まれており、残りは無傷のグリコフォームであることがわかりました。
Affinity data in Figure 2d show that the enzymatic removal of the N343 glycan from the WHu-1 RBD causes a complete loss of GM1os/2os binding, which is consistent with both, the involvement of the junction between Regions 1 and 2 the binding and its allosteric control of the RBM dynamics.
図 2d の親和性データは、WHu-1 RBD から N343 グリカンを酵素的に除去すると、GM1os/2os 結合が完全に失われることを示しています。 これは、領域 1 と 2 の間の結合の関与、結合と RBM ダイナミクスのアロステリック制御の両方と一致します。
Furthermore, while binding of GM1os and GM2os to the alpha RBD appears to be slightly decreased relative to WHu-1, binding to the beta RBD is dramatically reduced.
さらに、アルファ RBD への GM1os および GM2os の結合は、WHu-1 と比較してわずかに減少しているように見えますが、ベータ RBD への結合は劇的に減少しています。
We can reconcile this finding with the mutation E484K in beta, which changes the key interaction between E484 and GM1os, see Figure 2 and with changes in structure and dynamics of the RBM terminal hairpin induced by mutations, which have also been suggested to affect the S opening kinetics.
この発見は、E484 と GM1os の間の重要な相互作用を変化させる、ベータ版の変異 E484K と一致します。図 2 を参照してください。 また、変異によって引き起こされる RBM 末端ヘアピンの構造と動態の変化も、S 開口速度に影響を与えることが示唆されています。
Effects of N343 glycosylation on the structure of the delta RBD.
デルタ RBD の構造に対する N343 グリコシル化の影響。
The delta (B.1.617.2) RBD carries two mutations, namely L452R and T478K, relative to the WHu-1 strain.
デルタ (B.1.617.2) RBD は、WHu-1 株と比較して、2 つの突然変異、すなわち L452R および T478K を保有しています。
The open RBD in the cryo-EM structure PDB 7V7Q was used as starting conformation for the MD simulations of both the glycosylated, and the non-glycosylated delta RBDs.
クライオ EM 構造 PDB 7V7Q のオープン RBD は、グリコシル化デルタ RBD と非グリコシル化デルタ RBD の両方の MD シミュレーションの開始立体構造として使用されました。
To understand how the mutations in delta affect the RBD structure and modulate the response to the loss of glycosylation at N343, we ran two uncorrelated conventional MD simulations (2 μs) and one GaMD simulation (2 μs) for both the glycosylated and non-glycosylated systems, for a total (cumulative) sampling of 12 μs.
デルタの変異が RBD 構造にどのように影響し、N343 でのグリコシル化の喪失に対する応答を調節するかを理解するために、グリコシル化システムと非グリコシル化システムの両方について、相関のない従来の MD シミュレーション (2 μs) と 1 つの GaMD シミュレーション (2 μs) を実行しました。 合計 (累積) サンプリングは 12 μs です。
Results are shown in Figure 3.
結果を図 3に示します。
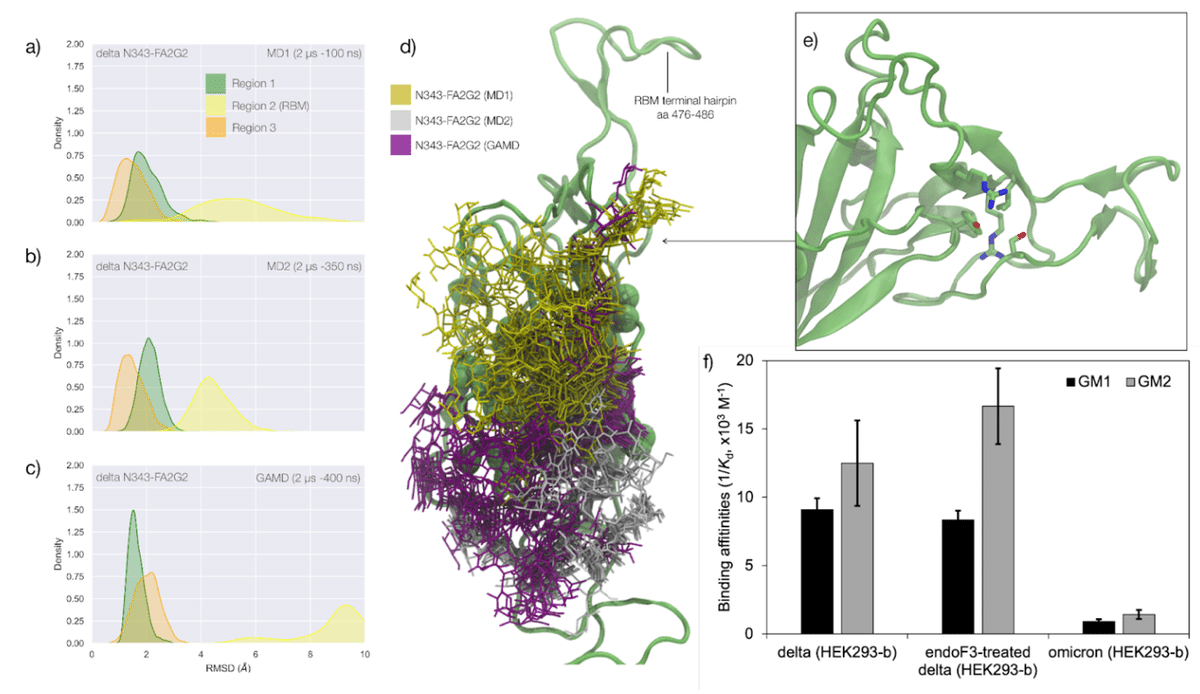
In the glycosylated delta RBD the N343 glycan is observed to be much more dynamic than in the WHu-1, alpha and beta RBDs, engaging in contacts with different regions of the RBD in addition to the loop aa 365-375.
グリコシル化デルタ RBD では、N343 グリカンが WHu-1、アルファ、およびベータ RBD よりもはるかに動的であることが観察され、ループ aa 365 ~ 375 に加えて RBD のさまざまな領域と接触しています。
In response to these fluctuations the conformation of the RBD remains stable with only minor deviations from the average structure of Regions 1 and 3.
これらの変動に応じて、RBD の立体構造は領域 1 および 3 の平均構造からわずかに逸脱するだけで安定した状態を保ちます。
All trajectories, and in particular the results of the GaMD simulation in Figure 3c, show that RBM (Region 2) of delta is highly dynamic.
すべての軌跡、特に図 3c の GaMD シミュレーションの結果は、デルタの RBM (領域 2) が非常に動的であることを示しています。
This flexibility appears to involve specifically the terminal hairpin of the RBM (aa 476 to 486), which includes the T478K mutation, while the rest of the RBM is tightly anchored due to the L454R mutation.
この柔軟性には、T478K 変異を含む RBM の末端ヘアピン (aa 476 ~ 486) が特に関与しているようです。 一方、残りの RBM は L454R 変異によりしっかりと固定されています。
More specifically, the R452 of delta can establish a new hydrogen bond with Y351, in addition to S469 and T470, reinforcing the junction between Regions 1 and 2.
より具体的には、デルタの R452 は、S469 および T470 に加えて、Y351 と新しい水素結合を確立することができ、領域 1 と 2 の間の結合を強化します。
Furthermore, in the delta RBM the role of L452 in CH-π stacking to Y351 is taken by the proximal L492, through a twist of the beta sheet, see Figure 3e.
さらに、デルタRBMでは、Y351へのCH-πスタッキングにおけるL452の役割は、ベータシートのねじれを通じて近位のL492によって引き受けられます(図3eを参照)。
These interactions also contribute to reinforcing the R454 orientation, tightening the link with the RBM hydrophilic patch.
これらの相互作用は、R454 の配向の強化にも寄与し、RBM 親水性パッチとのつながりを強化します。
The effect of the loss of glycosylation at N343 on the delta RBD was assessed by running two uncorrelated MD simulations, one by conventional sampling (MD1 of 3 μs) and the other through enhanced sampling (GaMD of 2 μs).
デルタ RBD に対する N343 でのグリコシル化の喪失の影響は、2 つの無相関 MD シミュレーションを実行することによって評価されました。1 つは従来のサンプリング (3 μs の MD1) によるもので、もう 1 つは強化されたサンプリング (2 μs の GaMD) によるものです。
As a consequence of the L452R mutation shown in Figure 3, the tightening of the helical loops aa 335-345 and aa 365-375 over the hydrophobic core of the RBD occurring upon loss of glycosylation at N343 does not affect the structure and dynamic of the junction between Regions 1 and 2, see Figure S.3.
図 3 に示す L452R 変異の結果として、N343でのグリコシル化の喪失時に起こるRBDの疎水性コア上のらせんループaa 335-345およびaa 365-375の締め付けは、領域1と2の間の接合の構造および動態に影響を与えません。 図S.3を参照してください。
Results of the conventional MD simulation show that the tightening of the loops is mainly achieved by a larger displacement of the aa 365-375 loop rather than of Region 1, while the GaMD results show tightening of both loops, see Figure S.3.
従来の MD シミュレーションの結果は、ループの締め付けは主に、領域 1 ではなく aa 365-375 ループのより大きな変位によって達成されることを示しています。 一方、GaMD の結果は両方のループの締め付けを示しています (図 S.3 を参照)。
In all simulations the structure of the junction between Regions 1 and 2 remains undisturbed, with no detachment of the hydrophilic patch within the sampling we collected.
すべてのシミュレーションにおいて、領域 1 と 2 の間の接合部の構造は乱されず、収集したサンプル内の親水性パッチが剥離することはありませんでした。
Effect of the loss of N343 glycosylation on the binding affinity of GM1/2 for the delta RBD.
デルタRBDに対するGM1/2の結合親和性に対するN343グリコシル化の喪失の影響。
To examine the effect of N343 glycosylation on glycan binding of delta RBD, we used the direct ESI-MS assay to quantify the binding affinities between endo F3-treated delta and GM1os and GM2os.
デルタ RBD のグリカン結合に対する N343 グリコシル化の影響を調べるために、直接 ESI-MS アッセイを使用して、endo F3 処理デルタと GM1os および GM2os の間の結合親和性を定量しました。
From the zero-charge mass spectra of endoF3-treated RBD, see Figure S.5, we performed glycan assignment, see Table S.9, and found that both N331 and N343 glycans were trimmed down to Gn/GnF.
endoF3 処理 RBD のゼロ電荷質量スペクトル (図 S.5 を参照) から、グリカンの割り当てを実行しました (表 S.9 を参照)。その結果、N331 グリカンと N343 グリカンの両方が Gn/GnF までトリミングされたことがわかりました。
Direct ESI-MS data in Figure 3f show no loss of GM1/2 binding in the delta RBD upon loss of N343 glycosylation, which further supports the involvement of the Region 1 to 2 junction in sialylated glycans recognition.
図 3f の直接 ESI-MS データは、N343 グリコシル化の喪失時にデルタ RBD における GM1/2 結合の喪失がないことを示しており、これはシアル化グリカン認識における領域 1 と 2 の接合部の関与をさらに裏付けています。
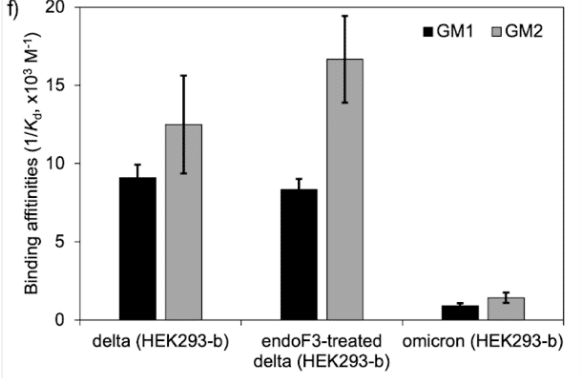
Effects of N343 glycosylation on the RBD structure in the omicron BA.1 SARS-CoV-2.
オミクロン BA.1 SARS-CoV-2 の RBD 構造に対する N343 グリコシル化の影響。
The omicron BA.1 RBD carries 15 mutations relative to the WHu-1 strain, namely S371L, S373P, S375F, K417N, N440K, G446S, S477N, T478K, E484A, Q493R, G496S, Q498R, N501Y, Y505H, and T547K.
オミクロン BA.1 RBD は、WHu-1 株と比較して 15 個の変異を持っています。 すなわち、S371L、S373P、S375F、K417N、N440K、G446S、S477N、T478K、E484A、Q493R、G496S、Q498R、N501Y、Y505H、およびT547K。
The S371L, S373P, and S375F mutations, retained in all omicron VoCs including the most recently circulating XBB.1.5, EG.5.1 and BA.2.86, remove all hydroxyl sidechains that we have seen being involved in hydrogen bonding interactions with the N343 glycan in the WHu-1, alpha, beta and delta RBDs, see Figures 1c and S.3.
最近流行した XBB.1.5、EG.5.1、BA.2.86 を含むすべてのオミクロン VoC に保持されている、S371L・S373P・S375F 変異は、WHu-1、アルファ、ベータおよびデルタ RBD の N343 グリカンとの水素結合相互作用に関与していることが確認されているすべてのヒドロキシル側鎖を除去します。図 1c および S.3 を参照してください。
We investigated the effects of the loss of glycosylation at N343 in the structure of the BA.1 RBD through two sets of uncorrelated conventional MD simulations (MD1 and MD2) and one set of GaMD, with total cumulative simulation time of 12 μs.
我々は、2 セットの相関のない従来の MD シミュレーション (MD1 および MD2) と 1 セットの GaMD を介して、BA.1 RBD の構造における N343 でのグリコシル化の損失の影響を調査しました。合計累積シミュレーション時間は 12 μs でした。
Starting structures correspond to the open RBD in PDB 7QO7 (MD1) and in PDB 7WVN (MD2 and GaMD), where the N343 glycan was reconstructed in different conformations, depending on the spatial orientation of the N343 sidechain.
開始構造は、PDB 7QO7 (MD1) および PDB 7WVN (MD2 および GaMD) のオープン RBD に対応します。 ここで、N343 グリカンは、N343 側鎖の空間的配向に応じて、異なる立体構造で再構築されました。
The results of the MD1 and GaMD simulations show that, despite the S371L, S373P, and S375F mutations, the N343 glycan is still forms stable contacts with the aa 365-375 loop, see Figures 1c and S.3, and these interactions contribute to the stability of the RBD structure, see Figure 4.
MD1 および GaMD シミュレーションの結果は、S371L、S373P、および S375F 変異にもかかわらず、N343 グリカンが依然として aa 365-375 ループと安定した接触を形成していることを示しています (図 1c および S.3 を参照)。 これらの相互作用は RBD 構造の安定性に寄与します (図 4 を参照)。
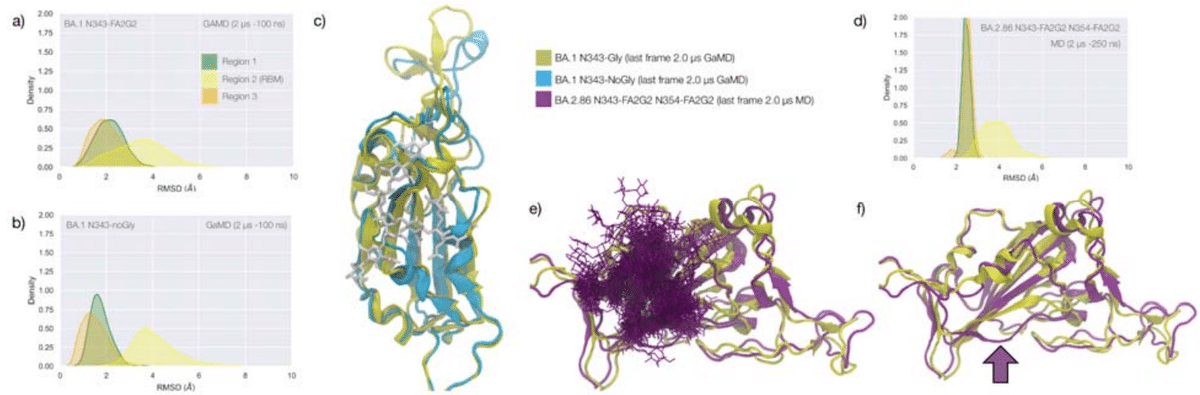
In the starting structure we used for MD2 the N343 glycan was built with the core pentasaccharide pointing away from the RBD hydrophobic core.
MD2 に使用した出発構造では、N343 グリカンは、RBD 疎水性コアから離れる方向を向いたコア五糖で構築されました。
Consequently, the N-glycan adopts different transient conformations during the MD2 trajectory, which terminate with an interaction with the hydrophobic interior of the RBD and with the N331 glycan, see Figure S.6.
その結果、N グリカンは MD2 軌道中に異なる一時的な構造をとり、RBD の疎水性内部および N331 グリカンとの相互作用で終了します。図 S.6 を参照してください。
In all simulations the loss of glycosylation at N343 causes a tightening of the aa 335-345 and aa 365-375 loops, which in omicron is stabilised by more efficient packing of the aa 365-375 loop within the hydrophobic core, driven by the embedding of the L371 and F375 sidechains.
すべてのシミュレーションにおいて、N343 でのグリコシル化の喪失により、aa 335-345 および aa 365-375 ループの締め付けが引き起こされます。 これは、L371およびF375側鎖の埋め込みによって駆動され、疎水性コア内のaa 365-375ループのより効率的なパッキングによってオミクロンで安定化されます。
The non-glycosylated RBD adopts a stable conformation where we do not see a detachment of the hydrophilic patch.
非グリコシル化 RBD は安定な立体構造をとり、親水性パッチの剥離は見られません。
The stabilising effect of the aa 365-375 loop mutations in omicron could not be tested by means of affinity for GM1os/2osas omicron (BA.1) binds those epitopes only weakly, see Figure 3f.
オミクロンにおけるaa 365-375ループ変異の安定化効果は、GM1os/2osasオミクロン(BA.1)に対する親和性によってはテストできませんでした。これらのエピトープには弱くしか結合しません。図3fを参照してください。
Based on the binding site we predicted by MD simulations in earlier work, see Figures 1d and 2e, and as observed for beta, the loss of E484 due to the E484A mutation in omicron may negate GM1os/2os binding.
以前の研究で MD シミュレーションによって予測され(図 1d と 2e を参照)、ベータで観察された結合部位に基づいて、オミクロンにおける E484A 変異による E484 の喪失は、GM1os/2os 結合を無効にする可能性があります。
The stability of the RBD structure is further enhanced by the presence of an additional glycosylation site at N354, which appeared in the recently detected omicron BA.2.86 ‘pirola’ variant.
RBD 構造の安定性は、N354 に追加のグリコシル化部位が存在することによってさらに強化されます。この部位は、最近検出されたオミクロン BA.2.86 の「ピロラ」変異体に現れています。
As shown in Figure 4d-f, the N-glycans at N343 and N354 are tightly intertwined throughout the trajectory stabilising Region 1, also shielding the area very effectively.
図 4d ~ f に示すように、N343 と N354 の N-グリカンは領域 1 を安定させる軌道全体にわたって緊密に絡み合っており、その領域を非常に効果的にシールドしています。
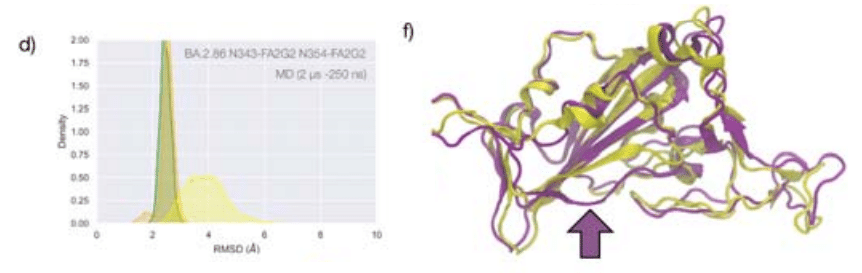
The presence of an additional N-glycosylation site at N354 also changes the conformation of the loop that hosts the site relative to the BA.1 starting structure we used as a template to run the MD simulation, see Figure 4f.
N354 に追加の N-グリコシル化部位が存在すると、MD シミュレーションを実行するためのテンプレートとして使用した BA.1 開始構造と比較して、その部位をホストするループの立体構造も変化します (図 4f を参照)。
To note, based on earlier glycoproteomics analysis and on the exposure to the solvent of the reconstructed glycan structure at N354, we chose to occupy all glycosylation sites with FA2G2 N-glycans.
以前の糖プロテオミクス分析と、N354 で再構築されたグリカン構造の溶媒への曝露に基づいて、すべてのグリコシル化部位を FA2G2 N-グリカンで占めることを選択したことに注意してください。
Discussion
Quantifying the role of glycosylation in protein folding and structural stability is a complex task due to the dynamic nature of the glycan structures and to the micro- and macro-heterogeneity in their protein functionalization that hinder characterization.
タンパク質のフォールディングと構造安定性におけるグリコシル化の役割を定量化することは、グリカン構造の動的な性質と特性評価を妨げるタンパク質の機能化におけるミクロおよびマクロの不均一性により複雑な作業です。
Yet, the fact that protein folding occurs within a context where glycosylation types and occupancy can change on the fly, suggests that not all glycosylation sites are essential for the protein to achieve and retain a native fold and that those sites may be displaced without consequences to function.
しかし、タンパク質のフォールディングは、グリコシル化の種類と占有率がその場で変化する可能性がある状況で発生するという事実は、次のことを示唆しています。 すべてのグリコシル化部位がタンパク質が天然の折り畳みを達成して保持するために必須であるわけではなく、それらの部位が機能に影響を与えずに置換される可能性もあります。
In this work we investigated the structural role of the N-glycosylation at N343 in the SARS-CoV-2 S RBD, one of the most highly conserved sites in the viral phylogeny.
この研究では、ウイルス系統発生において最も高度に保存されている部位の 1 つである SARS-CoV-2 S RBD の N343 における N-グリコシル化の構造的役割を調査しました。
Extensive MD simulations in this and in earlier work by us and others show that the RBD core is effectively shielded by this glycan.
この研究および我々や他の研究者による以前の研究における広範な MD シミュレーションは、RBD コアがこのグリカンによって効果的に保護されていることを示しています。
Furthermore, the N343 glycan has been shown to be mechanistically involved in the opening and closing of the S, making this glycosylation site functionally essential towards viral infection.
さらに、N343 グリカンは S の開閉に機構的に関与していることが示されており、このグリコシル化部位はウイルス感染に対して機能的に不可欠となっています。
In this work we performed over 45 μs of cumulative MD sampling with both conventional and enhanced schemes to show that the N343 glycan also plays a fundamental structural role in the WHu-1 SARS-CoV-2 and that this role has changed in the variants circulating thus far.
この研究では、従来のスキームと拡張スキームの両方を使用して 45 μs を超える累積 MD サンプリングを実行し、次のことを示しました。 N343 グリカンは WHu-1 SARS-CoV-2 においても基本的な構造的役割を果たしており、この役割はこれまでに流行している変異株では変化していることがわかっています。
While we cannot gauge how fundamental is N343 glycosylation towards RBD folding, we see that the amphipathic nature of the complex N-glycan at N343 enhances the stability of the RBD architecture, bridging between the two partially helical loops that frame a highly hydrophobic beta sheet core.
RBD フォールディングに向けて N343 グリコシル化がどの程度基礎的であるかを測定することはできませんが、 N343 の複合 N-グリカンの両親媒性の性質が RBD 構造の安定性を高め、疎水性の高いベータ シート コアを構成する 2 つの部分的に螺旋状のループの間を橋渡ししていることがわかります。
To note, we determined the same bridging structures also for oligomannose types N-glycans at N343 in earlier work.
注目すべきことに、我々は以前の研究でN343のオリゴマンノース型N-グリカンについても同じ架橋構造を決定した。
In all variants we observe that the removal of the glycan at N343 triggers a tightening of the loops in a response likely aimed at limiting access of water into the hydrophobic core.
すべての変異体において、N343 のグリカンの除去により、疎水性コアへの水のアクセスを制限することを目的とした応答としてループの締め付けが引き起こされることが観察されています。
In WHu-1, alpha and beta RBDs this event allosterically controls the dynamics of the RBM, ultimately causing the detachment of the hydrophilic patch and misfolding from the ACE2-recognized conformation.
WHu-1、アルファおよびベータ RBD では、このイベントが RBM のダイナミクスをアロステリックに制御し、最終的には親水性パッチの剥離と ACE2 が認識する立体構造からのミスフォールディングを引き起こします。
These results are in agreement with the drastic reduction of viral infectivity observed upon deletion of both N331 and N343 glycosylation in the WHu-1 strain, where loss of structure may add to the loss of function through gating or vice versa.
これらの結果は、WHu-1 株における N331 および N343 グリコシル化の両方の欠失によって観察されたウイルス感染力の大幅な低下と一致しています。 構造の損失がゲート制御による機能の損失に加わる可能性がある場合、またはその逆の場合があります。
As a functional assay to support this molecular insight, we determined how the binding affinity of the RBD for the oligosaccharides of the monosialylated gangliosides GM1os and GM2os is modulated by N343 glycosylation.
この分子洞察を裏付ける機能アッセイとして、モノシアル化ガングリオシド GM1os および GM2os のオリゴ糖に対する RBD の結合親和性が N343 グリコシル化によってどのように調節されるかを決定しました。
These were shown in earlier work by us and others to function as co-receptors in WHu-1 infection.
これらは、我々や他の研究者らによる以前の研究で、WHu-1 感染において共受容体として機能することが示されています。
We predicted through extensive MD sampling that GM1os and GM2os bind the RBD into a site corresponding precisely to the location occupied by the 6-arm of an ancestral N-glycan at N370.我々は広範なMDサンプリングを通じて、GM1osおよびGM2osがN370の祖先N-グリカンの6アームが占める位置に正確に対応する部位にRBDを結合すると予測した。
Note, the N370 site is still occupied in zoonotic sabercoviruses.
N370 部位は依然として人獣共通感染症サベルコウイルスに占有されていることに注意してください。
The GM1os binding site, see Figure 2e, is located precisely at the junction between Regions 1 and 2, which is disrupted by the loss of N343 glycosylation in WHu-1.
GM1os 結合部位 (図 2e を参照) は、領域 1 と 2 の間の接合部に正確に位置しています。 これは、WHu-1 の N343 グリコシル化の喪失によって破壊されます。
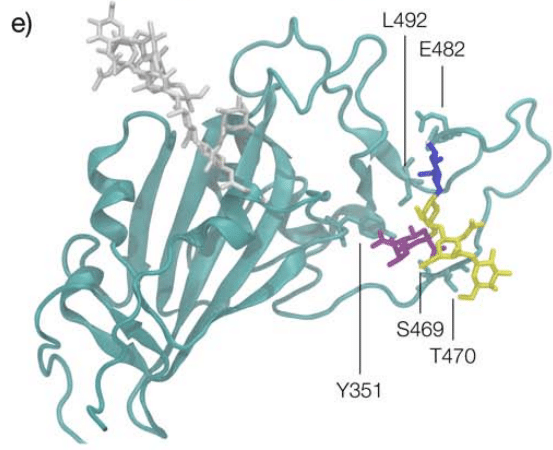
Accordingly, we find that enzymatic removal of the N343 glycan abolishes GM1os and GM2os binding in the WHu-1 RBD, see Figure 2d.
したがって、N343 グリカンの酵素的除去により、WHu-1 RBD における GM1os および GM2os の結合が消失することがわかりました。図 2d を参照してください。
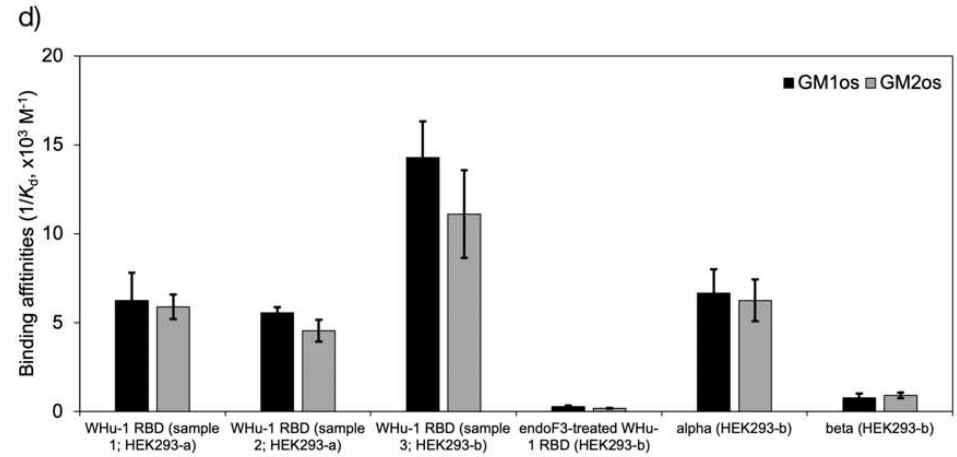
While we expect a similar loss of binding in alpha, within the context of a lower affinity relative to the WHu-1 RBD, we find that the beta RBD does not bind GM1os and GM2os, regardless of its glycosylation state.
WHu-1 RBDと比較して親和性が低いという状況の中で、アルファでも同様の結合の喪失が予想される一方で、ベータRBDは、グリコシル化状態に関係なく、GM1osおよびGM2osに結合しないことがわかりました。
Based on the structure of the GM1-RBD complex we identified, see Figure 2e, where E484 represents a key contact to the oligosaccharides, the mutation of E484K in beta may be key to the loss of binding, together with change in the RBM kinetics linked to this mutation and to variations within the same region.
私たちが同定したGM1-RBD複合体の構造に基づくと(図 2e を参照)、 E484 がオリゴ糖との重要な接触を表す場合、ベータの E484K の変異は、この変異と同じ領域内の変異に関連する RBM 動態の変化とともに、結合喪失の鍵となる可能性があります。
In the delta variant we observed that the L452R mutation is responsible for an increased structural stability of the RBD, reinforcing the non-covalent interactions network between Region 1 and the RBM.
デルタ変異体では、L452R 変異が RBD の構造安定性の向上に関与し、領域 1 と RBM の間の非共有結合的相互作用ネットワークが強化されることが観察されました。
Indeed, the tightening of the loops occurring upon loss of N343 glycosylation does not trigger a misfolding of the RBM, see Figure 3.
実際、N343 グリコシル化の喪失によって生じるループの緊密化は、RBM のミスフォールディングを引き起こすわけではありません (図 3 を参照)。
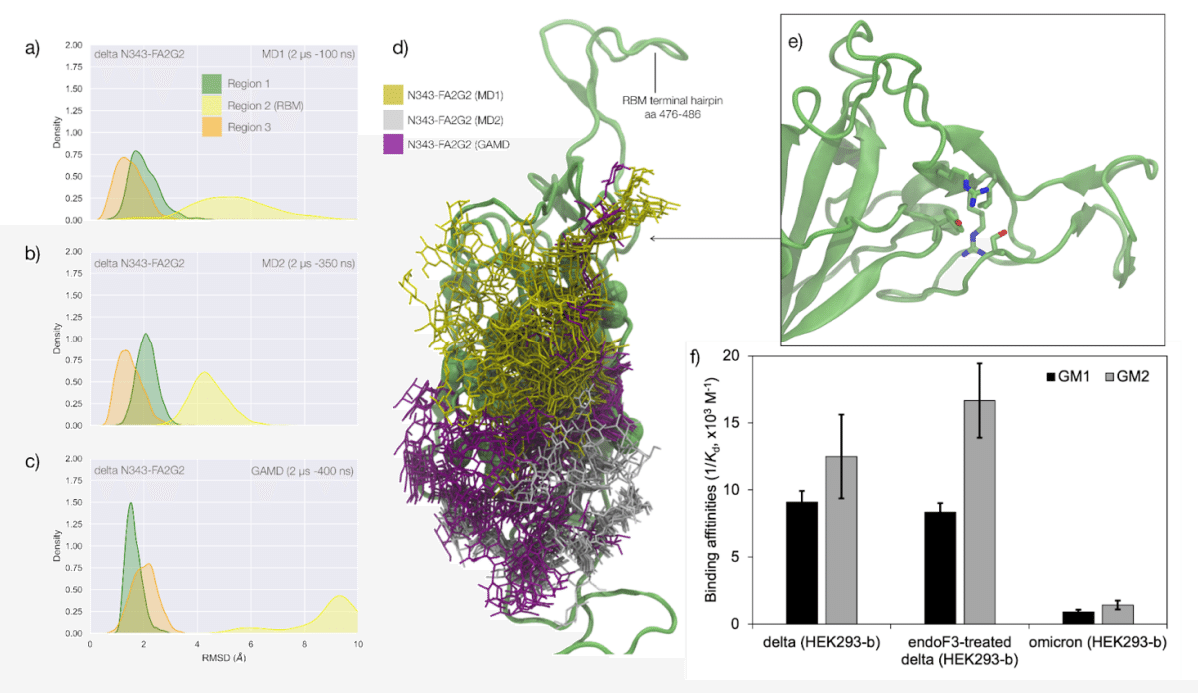
Accordingly, we observe that the delta RBD with the trimming down of N331 and N343 glycans shows no significant change in binding affinity for GM1os and GM2os relative to the fully glycosylated form, see Figure 3f.
したがって、N331およびN343グリカンをトリミングしたデルタRBDは、完全にグリコシル化された形態と比較して、GM1osおよびGM2osに対する結合親和性に有意な変化を示さないことがわかります。図3fを参照してください。
In all omicron variants, including all the currently circulating VoCs and VUMs, the loop aa 365-375 that the N343 glycan hooks on, carries similar mutations, with the highly conserved S371, S373 and S375 all mutated to hydrophobic residues, see Figure S.1.
現在流通しているすべての VoC および VUM を含むすべてのオミクロン変異体では、N343 グリカンがフックするループ aa 365-375 に同様の変異が含まれています。 高度に保存された S371、S373、および S375 はすべて疎水性残基に変異しています。 図S.1を参照してください。
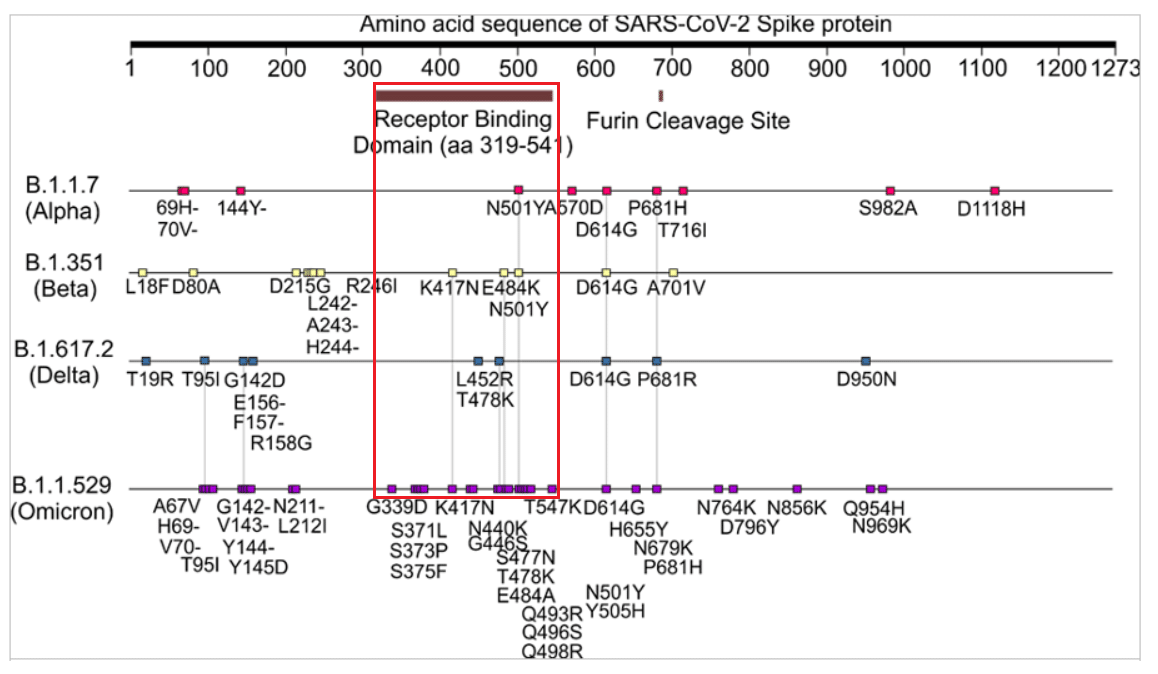
Our MD results on the BA.1 and BA.2.86 RBDs show that hydrophobic residues at positions 371, 372 and 373 can pack within the RBD core, while leading to a loop structure that can support the N343 glycan branches through interactions with the backbone, see Figure 4.
BA.1 および BA.2.86 RBD に関する我々の MD 結果は、371、372、および 373 位の疎水性残基が RBD コア内に詰め込まれる可能性があることを示しています。 バックボーンとの相互作用を通じて N343 グリカンの分岐をサポートできるループ構造をもたらします (図 4 を参照)。
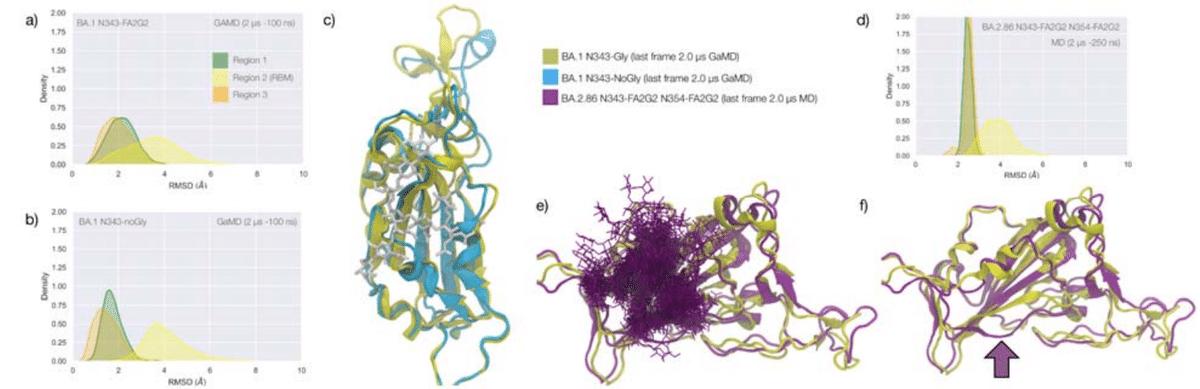
We have shown for all variants that the contacts between the N343 glycan and the aa 365-375 stretch of the opposite loop are fairly equally distributed, between hydrophilic (hydrogen bonding) and hydrophobic (dispersion or van der Waals) type interactions, see Figure 1c.
我々は、すべての変異体について、N343 グリカンと反対側のループの aa 365-375 ストレッチの間の接触が、親水性 (水素結合) と疎水性 (分散またはファンデルワールス) タイプの相互作用の間にかなり均等に分布していることを示しました。 図 1c を参照してください。
Therefore, it is expected that the loss of anchoring hydrogen bonding residues can be supported through other interactions.
したがって、アンカー水素結合残基の喪失は、他の相互作用を通じてサポートされ得ることが予想される。
Within this context, the removal of the N343 glycan does still cause a tightening of the loops, yet through a different mechanism relative to the other variants that ultimately does not appear to affect the RBM dynamics.
これに関連して、N343 グリカンの除去は依然としてループの引き締めを引き起こしますが、他の変異体とは異なるメカニズムを介して、最終的には RBM ダイナミクスに影響を与えないようです。
As in beta, for omicron there is negligible binding of the N343 glycosylated RBD to GM1os and GM2os, likely due to the E484A mutation, which based on the predicted structure of the GM1os/RBD complex, see Figure 1d and 2e, would deny a key interaction within the predicted binding site.
ベータと同様に、オミクロンについては、おそらく E484A 変異が原因で、N343 グリコシル化 RBD の GM1os および GM2os への結合は無視できます。これは、GM1os/RBD複合体の予測構造に基づくと(図 1d と 2e を参照)、予測結合部位内の重要な相互作用を否定することになります。
Taken together, our results show that since the WHu-1, alpha and beta strains, the RBD has evolved to make the N-glycosylation site at N343 structurally dispensable.
まとめると、我々の結果は、WHu-1、アルファおよびベータ株以来、N343 の N-グリコシル化部位が構造的に不要になるように RBD が進化したことを示しています。
Within this framework, provided that an N-glycosylation site in the immediate vicinity of N343 is necessary for folding and for function, a shift of the site within the sequence can potentially occur.
この枠組み内では、N343 のすぐ近くの N-グリコシル化部位がフォールディングと機能に必要である場合、配列内の部位のシフトが潜在的に発生する可能性があります。
Such a modification may negatively affect recognition and binding by neutralising antibodies and thus promote evasion.
このような修飾は、中和抗体による認識と結合に悪影響を及ぼし、回避を促進する可能性があります。
We have also shown for the BA.2.86 that the new glycosylation site at N354 can effectively contribute to the stability of Region 1, while significantly increasing shielding.
また、BA.2.86 については、N354 の新しいグリコシル化部位が、シールドを大幅に強化しながら、領域 1 の安定性に効果的に寄与できることも示しました。
Moreover, we show that specific VoCs lost affinity for monosialylated ganglioside oligosaccharides with a trend in agreement with a binding site located at the junction between Region 1 and the RBM, which is part of the N370 glycan binding cleft on the RBD.
さらに、特定の VoC は、領域 1 と RBM の間の接合部に位置する結合部位と一致する傾向で、モノシアル化ガングリオシドオリゴ糖に対する親和性を失ったことを示します。 これは、RBD 上の N370 グリカン結合溝の一部です。
This conclusion is further supported by how binding affinities for GM1os and GM2os change upon the loss of N343 glycosylation, in agreement with the MD results.
この結論は、MD の結果と一致して、N343 グリコシル化の喪失により GM1os および GM2os に対する結合親和性がどのように変化するかによってさらに裏付けられます。
Further to this, as mutations we identified dampened binding to monosialylated ganglioside oligosaccharides, it is also possible that further mutations may switch the affinity back on or determine a shift of preference of the RBD towards other glycans that can still be recognised within the N370 cleft.
これに加えて、突然変異として、モノシアル化ガングリオシドオリゴ糖への結合が弱まることを確認しました。 また、さらなる変異によって親和性が再びオンになったり、N370 クレフト内で依然として認識できる他のグリカンに対する RBD の優先順位のシフトが決定される可能性もあります。
Further work is ongoing in this area.
この分野ではさらなる作業が進行中です。
Finally, the results from this work point to the importance of understanding the impact of N-glycosylation in protein structure and stability, with immediate consequences to COVID-19 vaccine design.
最後に、この研究の結果は、タンパク質の構造と安定性におけるN-グリコシル化の影響を理解することの重要性を示しており、これはCOVID-19ワクチンの設計に即座に影響を及ぼします。
Indeed, earlier work shows SARS-CoV-2 S-based protein vaccines with increased efficacy due to the removal of N-glycans, and of RBD-based vaccines in use and under development that may be designed with and without N-glycans.
実際、以前の研究では、SARS-CoV-2 S ベースのタンパク質ワクチンが N-グリカンの除去により有効性を高めていることが示されています。 使用中および開発中の RBD ベースのワクチンについては、N-グリカンの有無にかかわらず設計できます。
The design of such constructs may benefit from understanding which N-glycosylation sites are structurally essential and which are dispensable.
このような構築物の設計には、どの N-グリコシル化部位が構造的に必須で、どの部位が不要であるかを理解することが有益となる可能性があります。
Further to this, our results show that taking into consideration the effects on N-glycosylation on protein structural stability and dynamics in the context of specific protein sequences may be key to understanding epistatic interactions among RBD residues, which would be otherwise difficult, where not impossible, to decipher.
これに加えて、我々の結果は、特定のタンパク質配列の文脈におけるタンパク質の構造安定性と動態に対するN-グリコシル化への影響を考慮することが、RBD残基間のエピスタティック相互作用を理解する鍵となる可能性があることを示しています。それはそれで難しいことですが、 不可能ではない場合でも、解読することは可能です。
以下省略。
この記事が気に入ったらサポートをしてみませんか?