COVID-19の基礎的復習
CLINICAL UPDATE Virology, transmission, and pathogenesis of COVID-19
BMJ Published: 23 October 2020
Muge Cevik, Krutika Kuppalli, Jason Kindrachuk, Malik Peiris
COVID-19のウイルス学からの解説を引用させて頂きます。
ウイルスの感染メカニズム等、ウイルス学という科学的側面からの解説です。
わたしのnoteにおいては、最新の科学・経済・社会等の問題に関して、英語の記事を引用し、その英文が読み易いように加工し、「英語の勉強ツール」と「最新情報収集ツール」としてご利用頂くことをmain missionとさせて頂きます。勿論、私論を書かせて頂くこともしばしです。
重症急性呼吸器症候群(SARS)
重症急性呼吸器症候群(SARS)は、SARSコロナウイルス (SARS-CoV-1) によって引き起こされるウイルス性の呼吸器疾患である。動物起源の人獣共通感染症と考えられている。2002年11月から2003年7月にかけて、中華人民共和国南部を中心に起きたアウトブレイクでは、広東省や香港を中心に8,096人が感染し、37ヶ国で774人が死亡した(致命率9.6%/WHO発表)(なお、世界30ヶ国8,422人が感染、916人が死亡〈致命率11%〉とする報告もある)。このアウトブレイク終息後は、封じ込め宣言後いくつかの散発例があったが、現在に至るまで、新規感染報告例は無い。
中国南部の広東省を起源とした重症な非定型性肺炎の世界的規模の集団発生が、2003年に重症急性呼吸器症候群(SARS: severe acute respiratory syndrome)の呼称で報告され、これが新型のコロナウイルスが原因であることが突き止められた。わが国においては、同年4月に新感染症に、ウイルス が特定された6月に指定感染症に指定され、2003年11月5日より感染症法の改正に伴い、第一類感染症としての報告が義務づけられるようになった。前回 の集団発生は2002年11月16日の中国の症例に始まり、台湾の症例を最後に、2003年7月5日にWHOによって終息宣言が出されたが、32の地域と 国にわたり8,000人を超える症例が報告された。
今回のCOVID-19 pandemic
世界保健機関(WHO)は、中国を中心に流行している新型コロナウイルスによる病気の正式名称を「COVID-19」に決定したと発表した。これまで使われていた「コロナウイルス」という単語はこの病気が属するウイルス群の名称で、病気そのものを指してはいなかった。一方、この新型ウイルス自体の名前は、国際ウイルス分類委員会(ICTV)によって「SARS-CoV-2」と名付けられている。
「COVID-19」は疾患名、その原因ウイルスが「SARS-CoV-2」
「SARS-CoV-1」は、「SARS」(疾患名)の原因ウイルス
今回のpandemicは、「COVID-19」:疾患名、その原因ウイルス:「SARS-CoV-2」であり、「SARS / 重症急性呼吸器症候群」:疾患名、その原因ウイルス:「SARS-CoV-1」とは異なるものである。
INTRODUCTION
Severe acute respiratory syndrome (SARS) is a viral respiratory disease of zoonotic (人畜[人獣]共通伝染病の、動物原性感染症の/zòuənóutik) origin caused by the first identified strain of severe acute respiratory syndrome coronavirus (SARS-CoV or SARS-CoV-1). The first known cases occurred in November 2002, and the syndrome caused the 2002–2004 SARS outbreak. Around late 2017, Chinese scientists traced the virus through the intermediary (仲介/ìntərmíːdièri) of Asian palm civets (ジャコウネコ/sívit) to cave-dwelling horseshoe bats in Xiyang Yi Ethnic Township, Yunnan. SARS was a relatively rare disease; at the end of the epidemic in June 2003, the incidence was 8,469 cases with a case fatality rate (CFR) of 11%. No cases of SARS-CoV-1 have been reported worldwide since 2004.
In December 2019, another strain of SARS-CoV was identified as severe acute respiratory syndrome coronavirus 2 (SARS-CoV-2). This new strain causes coronavirus disease 2019 (COVID-19), a disease which brought about the COVID-19 pandemic.
Severe acute respiratory syndrome coronavirus 1 (SARS-CoV-1 or SARS-CoV) is a strain of coronavirus that causes severe acute respiratory syndrome (SARS), the respiratory illness responsible for the 2002–2004 SARS outbreak. It is an enveloped, positive-sense, single-stranded RNA virus which infects the epithelial cells within the lungs. The virus enters the host cell by binding to angiotensin-converting enzyme 2. It infects humans, bats, and palm civets. On 16 April 2003, following the outbreak of SARS in Asia and secondary cases elsewhere in the world, the World Health Organization (WHO) issued a press release stating that the coronavirus identified by a number of laboratories was the official cause of SARS. The Centers for Disease Control and Prevention (CDC) in the United States and National Microbiology Laboratory (NML) in Canada identified the SARS-CoV-1 genome in April 2003. Scientists at Erasmus University in Rotterdam, the Netherlands, demonstrated that the SARS coronavirus fulfilled Koch's postulates (前提[必要・基礎]条件/pɔ́stjulit), thereby confirming it as the causative agent. In the experiments, macaques infected with the virus developed the same symptoms as human SARS victims.
A virus very similar to SARS was discovered in late 2019. This virus, named severe acute respiratory syndrome coronavirus 2 (SARS-CoV-2), is the causative pathogen of COVID-19, the propagation of which started the COVID-19 pandemic. The COVID-19 pandemic, also known as the coronavirus pandemic, is an ongoing global pandemic of coronavirus disease 2019 (COVID-19) caused by severe acute respiratory syndrome coronavirus 2 (SARS-CoV-2). The novel virus was first identified from an outbreak in the Chinese city of Wuhan in December 2019, and attempts to contain it there failed, allowing it to spread across the globe. The World Health Organization (WHO) declared a Public Health Emergency of International Concern on 30 January 2020 and a pandemic on 11 March 2020. As of 6 January 2022, the pandemic had caused more than 298 million cases and 5.46 million deaths, making it one of the deadliest in history. COVID-19 symptoms range from undetectable to deadly. Severe illness is more likely in elderly patients and those with certain underlying medical conditions. COVID‑19 transmits when people breathe in air contaminated by droplets and small airborne particles containing the virus. The risk of breathing these in is highest when people are in close proximity, but they can be inhaled over longer distances, particularly indoors. Transmission can also occur if contaminated fluids reach the eyes, nose or mouth, and, rarely, via contaminated surfaces. Infected persons are typically contagious (感染力がある/kəntéidʒəs) for 10 days, and can spread the virus even if they do not develop symptoms. Mutations have produced many strains (variants) with varying degrees of infectivity and virulence.
ここから本文
What you need to know
• SARS-CoV-2 is genetically similar to SARS-CoV-1, but characteristics of SARS-CoV-2—eg, structural differences in its surface proteins and viral load kinetics—may help explain its enhanced rate of transmission
• In the respiratory tract, peak SARS-CoV-2 load is observed at the time of symptom onset or in the first week of illness, with subsequent decline thereafter, indicating the highest infectiousness potential just before or within the first five days of symptom onset
• Reverse transcription polymerase chain reaction (RT-PCR) tests can detect viral SARS-CoV-2 RNA in the upper respiratory tract for a mean of 17 days; however, detection of viral RNA does not necessarily equate to infectiousness, and viral culture from PCR positive upper respiratory tract samples has been rarely positive beyond nine days of illness
• Symptomatic and pre-symptomatic transmission (1-2 days before symptom onset), is likely to play a greater role in the spread of SARS-CoV-2 than asymptomatic transmission
Since the emergence of SARS-CoV-2 in December 2019, there has been an unparalleled global effort to characterise the virus and the clinical course of disease. Coronavirus disease 2019 (covid-19), caused by SARS-CoV-2, follows a biphasic pattern of illness that likely results from the combination of an early viral response phase and an inflammatory second phase. Most clinical presentations are mild, and the typical pattern of covid-19 more resembles an influenza-like illness—which includes fever, cough, malaise, myalgia, headache, and taste and smell disturbance—rather than severe pneumonia (although emerging evidence about long term consequences is yet to be understood in detail). In this review, we provide a broad update on the emerging understanding of SARS-CoV-2 pathophysiology, including virology, transmission dynamics, and the immune response to the virus. Any of the mechanisms and assumptions discussed in the article and in our understanding of covid-19 may be revised as further evidence emerges. What we know about the virus SARS-CoV-2 is an enveloped β-coronavirus, with a genetic sequence very similar to SARS-CoV-1 (80%) and bat coronavirus RaTG13 (96.2%). The viral envelope is coated by spike (S) glycoprotein, envelope (E), and membrane (M) proteins (fig 1). Host cell binding and entry are mediated by the S protein. The first step in infection is virus binding to a host cell through its target receptor. The S1 sub-unit of the S protein contains the receptor binding domain that binds to the peptidase domain of angiotensin-converting enzyme 2 (ACE 2). In SARS-CoV-2 the S2 sub-unit is highly preserved and is considered a potential antiviral target. The virus structure and replication cycle are described in figure 1.
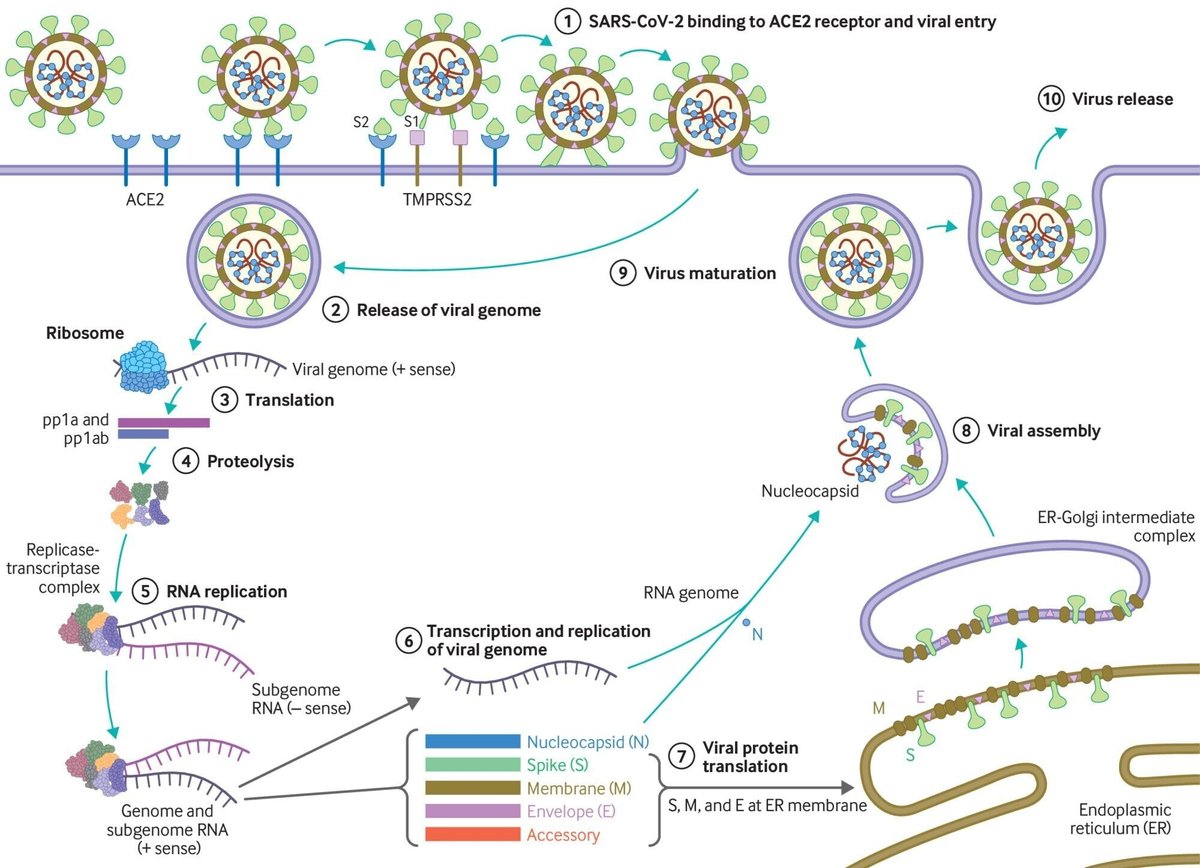
Coronaviruses have the capacity for proofreading (replicationの際に、相補的塩基を繋げていくが、その際に、誤って、相補的でない塩基を繋いでも、それを修正する能力) during replication, and therefore mutation rates are lower than in other RNA viruses. As SARS-CoV-2 has spread globally it has, like other viruses, accumulated some mutations in the viral genome, which contains geographic signatures. Researchers have examined these mutations to study virus characterisation and understand epidemiology and transmission patterns. In general, the mutations have not been attributed to phenotypic changes affecting viral transmissibility or pathogenicity.
The G614 variant in the S protein has been postulated to increase infectivity and transmissibility of the virus. Higher viral loads were reported in clinical samples with virus containing G614 than previously circulating variant D614, although no association was made with severity of illness as measured by hospitalisation outcomes.
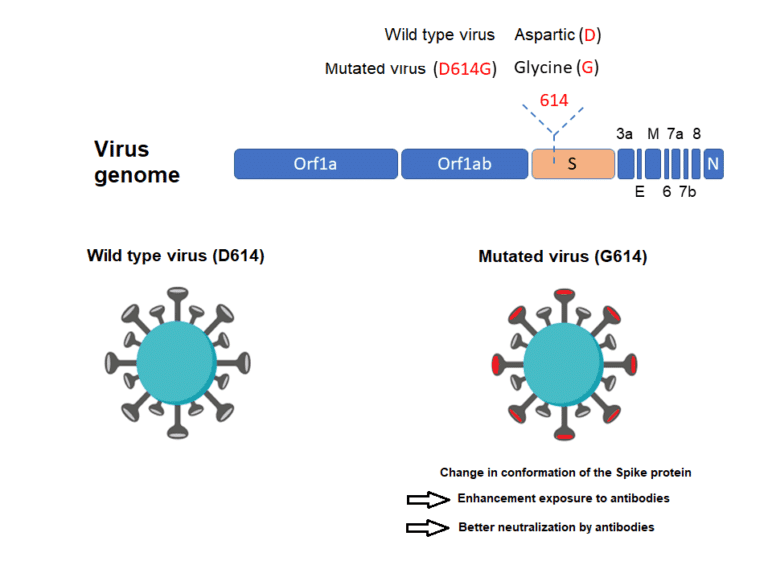
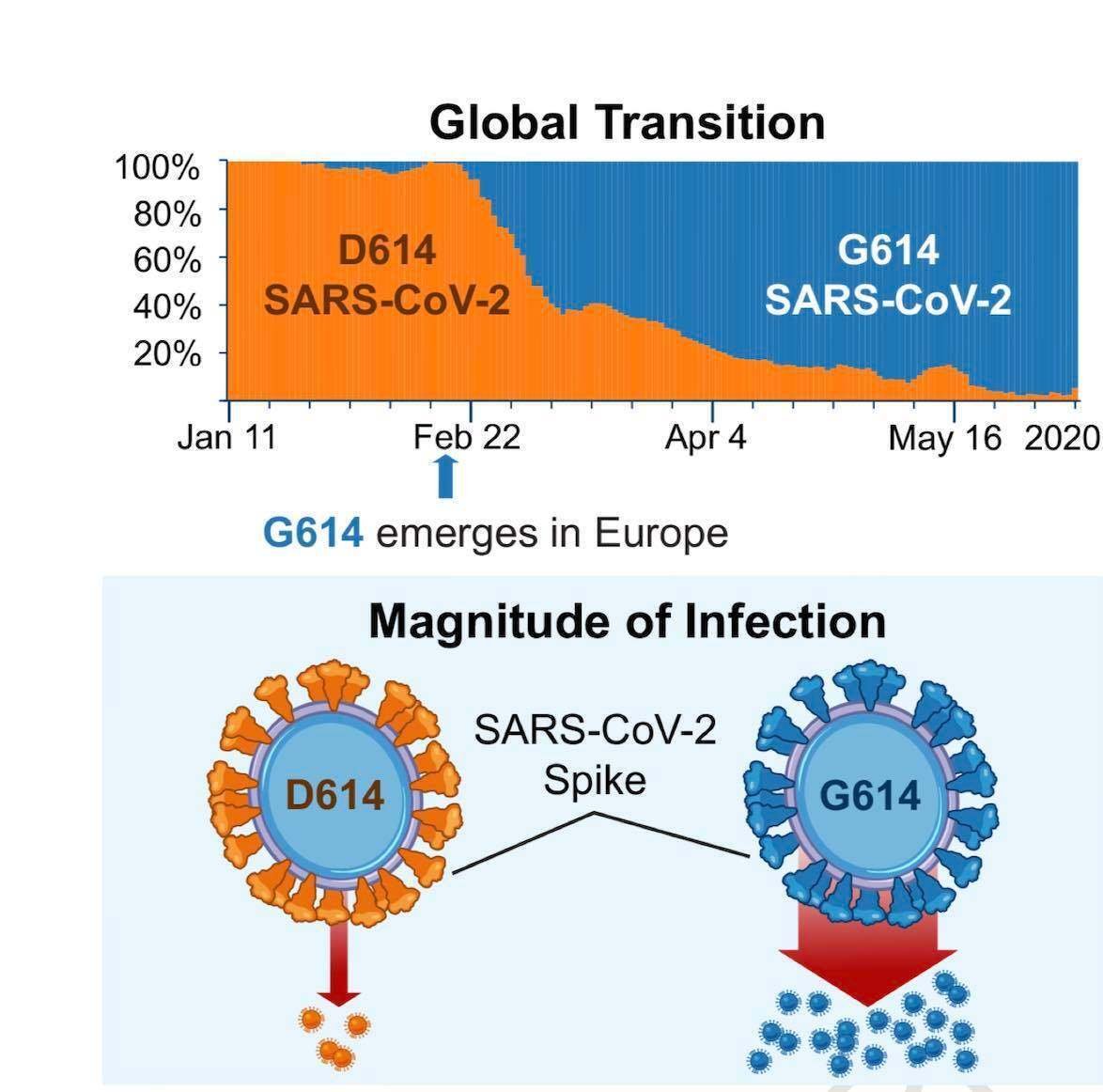
These findings have yet to be confirmed with regards to natural infection. Why is SARS-CoV-2 more infectious than SARS-CoV-1? SARS-CoV-2 has a higher reproductive number than SARS-CoV-1, indicating much more efficient spread. Several characteristics of SARS-CoV-2 may help explain this enhanced transmission. While both SARS-CoV-1 and SARS-CoV-2 preferentially interact with the angiotensin-converting enzyme 2 (ACE 2) receptor, SARS-CoV-2 has structural differences in its surface proteins that enable stronger binding to the ACE 2 receptor and greater efficiency at invading host cells. SARS-CoV-2 also has greater affinity (or bonding) for the upper respiratory tract and conjunctiva (結膜/kɑ̀ndʒəŋktáivə), thus can infect the upper respiratory tract and can conduct airways more easily.
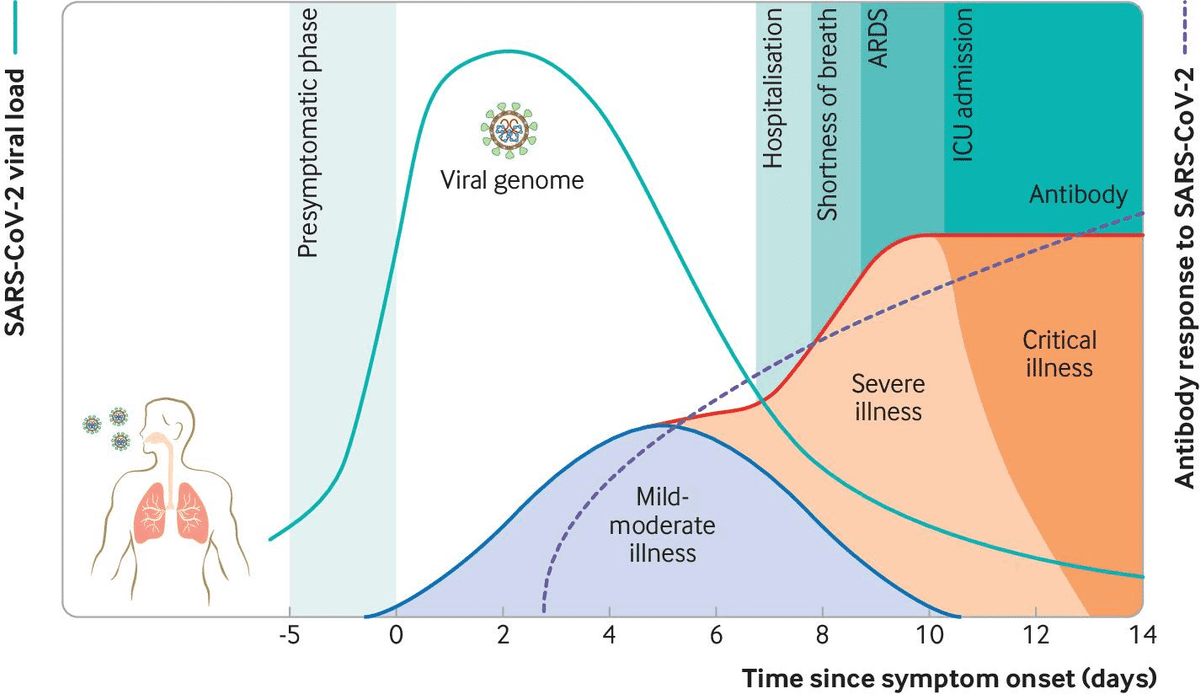
Viral load dynamics and duration of infectiousness
Viral load kinetics could also explain some of the differences between SARS-CoV-2 and SARS-CoV-1. In the respiratory tract, peak SARS-CoV-2 load is observed at the time of symptom onset or in the first week of illness, with subsequent decline thereafter, which indicates the highest infectiousness potential just before or within the first five days of symptom onset (fig 2). In contrast, in SARS-CoV-1 the highest viral loads were detected in the upper respiratory tract in the second week of illness, which explains its minimal contagiousness (伝染性) in the first week after symptom onset, enabling early case detection in the community.
Quantitative reverse transcription polymerase chain reaction (qRT-PCR) technology can detect viral SARS-CoV-2 RNA in the upper respiratory tract for a mean of 17 days (maximum 83 days) after symptom onset. However, detection of viral RNA by qRT-PCR does not necessarily equate to infectiousness, and viral culture from PCR positive upper respiratory tract samples has been rarely positive beyond nine days of illness. This corresponds to what is known about transmission based on contact tracing studies, which is that transmission capacity is maximal in the first week of illness, and that transmission after this period has not been documented. Severely ill or immune-compromised patients may have relatively prolonged virus shedding, and some patients may have intermittent RNA shedding; however, low level results close to the detection limit may not constitute infectious viral particles. While asymptomatic individuals (those with no symptoms throughout the infection) can transmit the infection, their relative degree of infectiousness seems to be limited. People [with mild symptoms] and those [whose symptom have not yet appeared] still carry large amounts of virus in the upper respiratory tract, which might contribute to the easy and rapid spread of SARS-CoV-2. Symptomatic and pre-symptomatic transmission (one to two days before symptom onset) is likely to play a greater role in the spread of SARS-CoV-2. A combination of preventive measures, such as physical distancing and testing, tracing, and self-isolation, continue to be needed.
Pathogenesis
Viral entry and interaction with target cells
SARS-CoV-2 binds to ACE 2, the host target cell receptor. Active replication and release of the virus in the lung cells lead to non-specific symptoms such as fever, myalgia, headache, and respiratory symptoms. In an experimental hamster model, the virus causes transient damage to the cells in the olfactory (嗅覚器官/ɑlfǽktəri) epithelium, leading to olfactory dysfunction, which may explain temporary loss of taste and smell commonly seen in covid-19. The distribution of ACE 2 receptors in different tissues may explain the sites of infection and patient symptoms. For example, the ACE 2 receptor is found on the epithelium of other organs such as the intestine and endothelial cells in the kidney and blood vessels, which may explain gastrointestinal symptoms and cardiovascular complications. Lymphocytic endotheliitis (Endotheliitis is an immune response within the endothelium in blood vessels, in which they become inflamed.) has been observed in postmortem pathology examination of the lung, heart, kidney, and liver as well as liver cell necrosis and myocardial infarction in patients who died of covid-19. These findings indicate that the virus directly affects many organs, as was seen in SARS-CoV-1 and influenzae. Much remains unknown. Are the pathological changes in the respiratory tract or endothelial dysfunction the result of direct viral infection, cytokine dysregulation, coagulopathy (血液凝固障害), or are they multifactorial? And does direct viral invasion or coagulopathy directly contribute to some of the ischaemic (虚血性) complications such as ischaemic infarcts? These and more, will require further work to elucidate. Immune response and disease spectrum (figure 2) after viral entry, the initial inflammatory response attracts virus-specific T cells to the site of infection, where the infected cells are eliminated before the virus spreads, leading to recovery in most people. In patients who develop severe disease, SARS-CoV-2 elicits an aberrant (異常な/ǽbərənt) host immune response. For example, postmortem histology of lung tissues of patients who died of covid-19 have confirmed the inflammatory nature of the injury, with features of bilateral diffuse alveolar damage, hyaline-membrane formation,
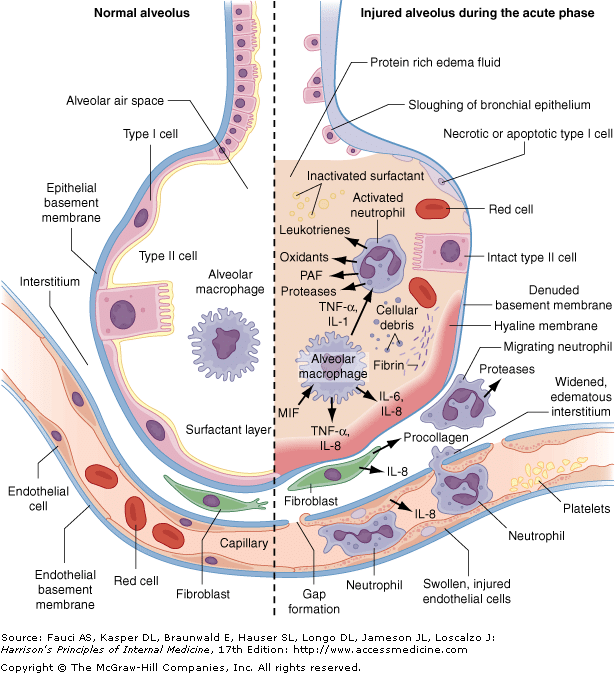
interstitial (間質性の/ìntərstíʃəl) mononuclear inflammatory infiltrates, and desquamation (細胞剥離/dèskwəméiʃən) consistent with acute respiratory distress syndrome (ARDS), and is similar to the lung pathology seen in severe Middle East respiratory syndrome (MERS) and severe acute respiratory syndrome (SARS). A distinctive feature of covid-19 is the presence of mucus plugs with fibrinous exudate (A fibrinous exudate is characteristic of inflammation in the lining of body cavities (体腔),
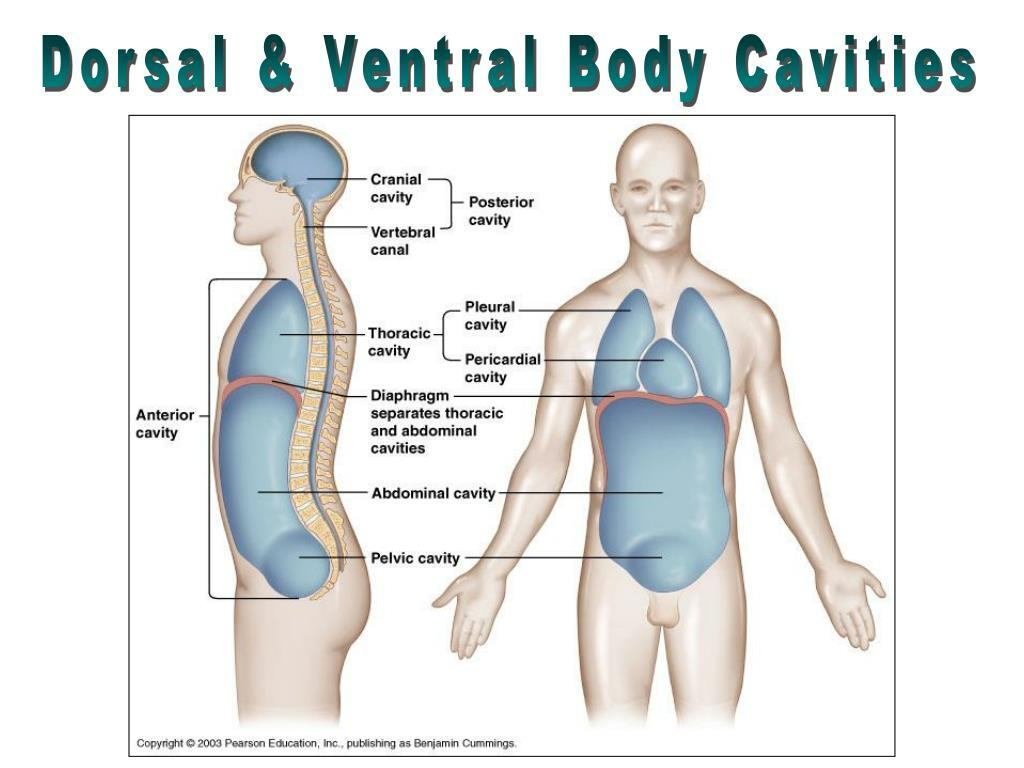
such as the meninges [髄膜の] (fibrinous meningitis), pericardium [心膜、心嚢] (fibrinous pericarditis), and pleura [胸膜] (fibrinous pleuritis).) in the respiratory tract, which may explain the severity of covid-19 even in young adults. This is potentially caused by the overproduction of pro-inflammatory cytokines that accumulate in the lungs, eventually damaging the lung parenchyma (肺実質). Some patients also experience septic shock and multi-organ dysfunction. For example, the cardiovascular system is often involved early in covid-19 disease and is reflected in the release of highly sensitive troponin and natriuretic peptides. Consistent with the clinical context of coagulopathy, focal intra-alveolar haemorrhage and presence of platelet-fibrin thrombi (血栓) in small arterial vessels is also seen. Cytokines normally mediate and regulate immunity, inflammation, and haematopoiesis; however, further exacerbation of immune reaction and accumulation of cytokines in other organs in some patients may cause extensive tissue damage, or a cytokine release syndrome (cytokine storm), resulting in capillary leak, thrombus formation, and organ dysfunction.Mechanisms underlying the diverse clinical outcomes Clinical outcomes are influenced by host factors such as older age, male sex, and underlying medical conditions, as well as factors related to the virus (such as viral load kinetics), host-immune response, and potential cross-reactive immune memory from previous exposure to seasonal coronaviruses.
Sex-related differences in immune response have been reported, revealing that men had higher plasma innate immune cytokines and chemokines at baseline than women. In contrast, women had notably more robust T cell activation than men, and among male participants T cell activation declined with age, which was sustained among female patients. These findings suggest that adaptive immune response may be important in defining the clinical outcome. As older age and male sex is associated with increased risk of severe disease and mortality. Increased levels of pro-inflammatory cytokines correlate with severe pneumonia and increased ground glass opacities
<Ground-glass opacity is a finding seen on chest x-ray or computed tomography imaging of the lungs. It is typically defined as an area of hazy (かすんだ) opacification (不透明化/oupæ̀sifikéiʃən) or increased attenuation due to air displacement by fluid, airway collapse, fibrosis, or a neoplastic process.>
within the lungs. In people with severe illness, increased plasma concentrations of inflammatory cytokines and biomarkers were observed compared with people with non-severe illness. Emerging evidence suggests a correlation between viral dynamics, the severity of illness, and disease outcome. Longitudinal (長期にわたる変化を扱った/lɑ̀ːndʒətúːdənəl) characteristics of immune response show a correlation between the severity of illness, viral load, and IFN- α, IFN-γ, and TNF-α response. In the same study many interferons, cytokines, and chemokines were elevated early in disease for patients who had severe disease and higher viral loads. This emphasises that viral load may drive these cytokines and the possible pathological roles associated with the host defence factors. This is in keeping with the pathogenesis of influenza, SARS, and MERS whereby prolonged viral shedding was also associated with severity of illness. Given the substantial role of the immune response in determining clinical outcomes, several immunosuppressive therapies aimed at limiting immune-mediated damage are currently in various phases of development.
Immune response to the virus and its role in protection
Covid-19 leads to an antibody response to a range of viral proteins, but the spike (S) protein and nucleocapsid are those most often used in serological diagnosis. Few antibodies are detectable in the first four days of illness, but patients progressively develop them, with most achieving a detectable response after four weeks. A wide range of virus-neutralising antibodies have been reported, and emerging evidence suggests that these may correlate with severity but wane over time. The duration and protectivity of antibody and T cell responses remain to be defined through studies with longer follow-up. CD-4 T cell responses to endemic human coronaviruses appear to manifest cross-reactivity with SARS-CoV-2, but their role in protection remains unclear.
Unanswered questions
Further understanding of the pathogenesis for SARS-CoV-2 will be vital in developing therapeutics, vaccines, and supportive care modalities in the treatment of covid-19. More data are needed to understand the determinants of healthy versus dysfunctional response and immune markers for protection and the severity of disease. Neutralising antibodies are potential correlates of protection, but other protective antibody mechanisms may exist. Similarly, the protective role of T cell immunity and duration of both antibody and T cell responses and the correlates of protection need to be defined. In addition, we need optimal testing systems and technologies to support and inform early detection and clinical management of infection. Greater understanding is needed regarding the long term consequences following acute illness and multisystem inflammatory disease, especially in children.
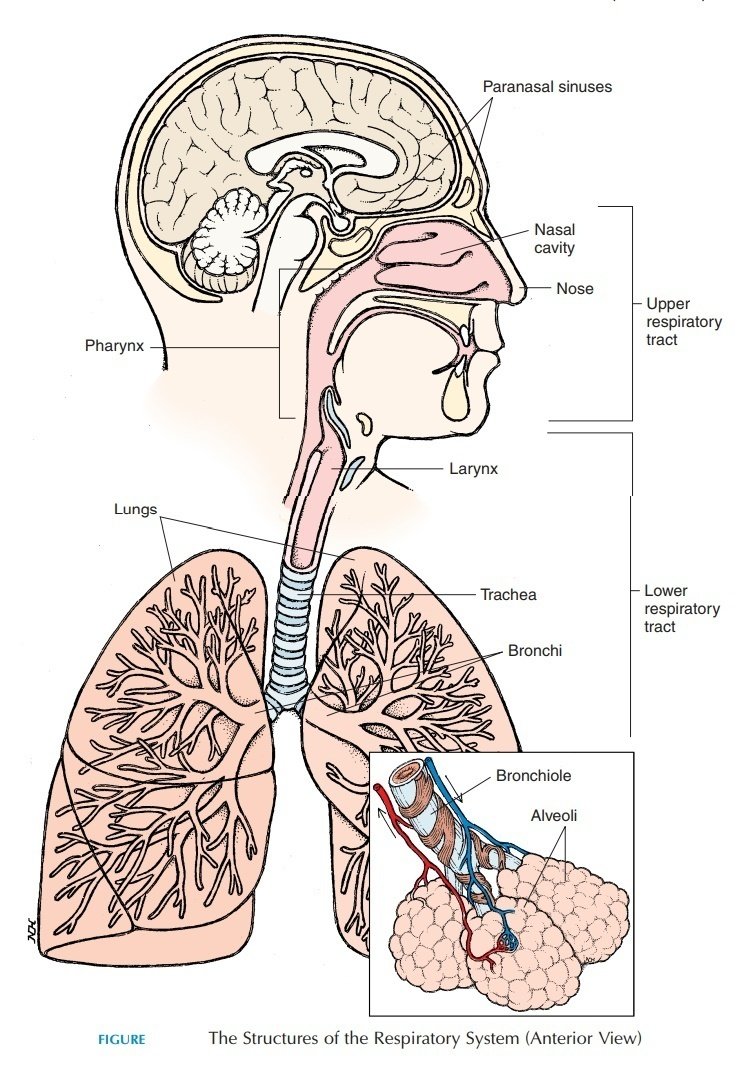
この記事が気に入ったらサポートをしてみませんか?